Abstract
This article is based on an interview between Prof. Martin Chalfie and Noa Segev.
Scientists investigate things by observation. They look at a phenomenon that interests them and try to understand it, using the most advanced tools they have. It is often challenging for scientists to see and measure what they want to study, often because they want to go beyond what had been previously seen. The development of modern imaging techniques has allowed scientists to see things that they could not see before. In this article, I will tell you about one of those breakthroughs in imaging, based on a wonderful glowing protein called green fluorescent protein (GFP). GFP not only changed my life, but the lives of many other scientists, and ultimately of many non-scientists as well. Among other things, GFP allows us to detect and observe the activity of proteins and whole cells in living animals, and to detect the activity of genes that code for specific proteins. By the end of this article, I hope you will understand much more about GFP and how it lights up science.
Professor Martin Chalfie won the Nobel Prize in Chemistry in 2008, jointly with Prof. Osamu Shimomura and Prof. Roger Tsien, for the discovery and development of the green fluorescent protein, GFP.
Glowing Jellyfish and a Miraculous Mistake
Have you ever been lucky enough to see the astoundingly beautiful phenomenon of a glowing firefly lighting up a dark night? Fireflies are part of a fascinating group of organisms that can produce light—a phenomenon that is called bioluminescence. Other bioluminescent organisms include glow-worms, some types of bacteria, and certain types of fish. Our story begins with a bioluminescent jellyfish called Aequorea victoria, or A. victoria for short (Figure 1).
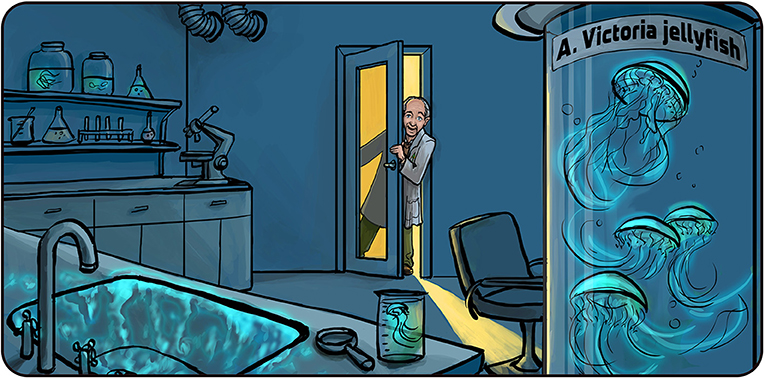
- Figure 1 - Osamu Shimomura and the glowing Aequorea victoria jellyfish.
- After a frustrating day in the lab, Prof. Shimomura turned off the lights and was about to go home for dinner. He suddenly saw that the sink, which had samples of A. victoria cells and sea water in it, was glowing in blue. That led him to the discovery of the glowing protein called aequorin. Illustration by: Iris Gat.
In the 1960s, a Japanese scientist named Osamu Shimomura wanted to understand how bioluminescent organisms produce light. He decided to work on A. victoria, which produces green light. Osamu worked for a whole summer, trying to make cells from A. victoria glow (as happens naturally in the sea) but none of his experiments succeeded. One night, when it was already dark outside and Osamu wanted to go home for dinner, he threw his samples of A. victoria proteins from another failed experiment into the lab’s sink and turned off the light to close up the lab. But just as he was about to leave, he noticed that the sink was glowing blue. Since the sink also contained sea water, Osamu reasoned that something in the sea water must have trigger the production of light. He soon realized that calcium in the seawater made the jellyfish proteins glow. He named the blue-glowing protein aequorin, after the name of the jellyfish [1, 2].
After this great breakthrough, Osamu had to answer another burning question: why did aequorin generate blue light, when the jellyfish it came from glow green? While purifying the aequorin protein, Osamu searched for something else that could create the green light emitted by A. victoria. Eventually, he found another protein that was a fluorescent molecule because it took the blue light, whether made from aequorin or from a hand-held lamp, and converted it into green light. This was another astonishing discovery, as no one at that time had any idea that proteins could be fluorescent. Although Osamu originally called this the green protein, we now call it the green fluorescent protein, or GFP [3]. This story is also a wonderful example of how many scientific discoveries are rather accidental. The role of the scientist, as in Osamu’s case, was to notice, wonder, and investigate these accidental discoveries.
How GFP Changed My Life
On Tuesday, April 25th, 1989, I heard a lunch-time lecture at my university about Osamu Shimomura’s work on GFP. As soon as I heard about GFP, I was fascinated. My lab was working on a tiny (about 1 mm long) transparent worm called Caenorhabditis elegans, or C. elegans. We were studying a group of the worm’s nerve cells that respond to physical stimuli, such as touch and sound, and convert it into electrical and chemical signals. I wondered whether we could find a way to make those nerve cells in C. elegans produce GFP, so we could actually see and study them in a completely new way. GFP could then be used as a biological marker, which is a biological molecule that scientists can observe to learn what is going on inside cells or organisms. At the time, the method we were using to see specific cell types in C. elegans was cumbersome and did not allow us to work with living tissue. These were serious limitations. We first had to “fix” the worm with chemicals to preserve the cell structure, but that process also killed the animal. That meant that our current method gave us only a “snapshot” picture of what was happening inside the worm—one “frame” at a time. I thought that GFP might allow us to view the nerve cells of C. elegans while it was alive and interacting with its environment.
I was so excited that I could not even listen to the rest of the lecture. All I could think about for the next few days was GFP and its potential for my research. I contacted a researcher named Douglas Prasher, who was working on producing and copying the genetic instructions (DNA) that coded for the GFP protein. We were both excited about the possibility of using GFP in C. elegans and other organisms, so we decided to collaborate. After losing contact for a few years due to an unfortunate misunderstanding, we reconnected in September 1992, when a student named Ghia Euskirchen came to my lab and was interested in the project. She had a good background in working with fluorescence, and this reminded me of my idea to use GFP to mark living cells. As we studied scientific papers published on GFP, we discovered that Douglas had recently published a paper on GFP [4] and contacted him again. Soon after, he sent us the DNA coding for GFP and Ghia started working with it.
Making Organisms Glow With GFP
When Ghia started her GFP experiments, she wanted to see if bacteria containing the DNA that codes for GPF would become fluorescent. We did not know at that time whether production of the GFP protein from GFP DNA was enough to make a cell fluorescent (to learn more about how proteins are made from DNA, see this article). It could be that something else was needed—either something that the cell itself produces and adds to the GFP protein to make it fluoresce, or something that must be added externally to make the cells fluoresce.
The first decision we had to make at the start of the experiment involved what to do with the GFP DNA that we got from Douglas. The DNA that Douglas sent us contained extra sequences in addition to the section that coded for GFP (Figure 2A). We knew we had to make lots of copies of the GFP DNA for our experiments, and the question was whether to use Douglas’s GFP DNA as-is (with the extra pieces) or work only with the part that coded for GFP. The tricky part was that the method was used to get only the coding DNA, called polymerase chain reaction (or PCR—which you might know from PCR tests for COVID-19) [5], often introduced mistakes in the copied DNA. Despite this limitation, I decided that we would use PCR, since we were going to be looking at millions of bacteria and some of them would have GFP DNA without any mistakes.
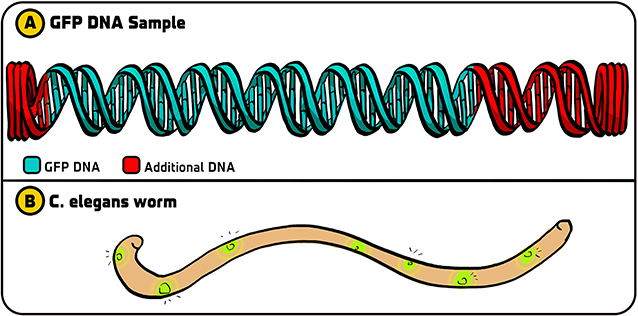
- Figure 2 - Initial experiments with GFP.
- (A) The original GFP DNA that we got from Douglas Prasher contained the DNA that coded for GFP (green) along with additional DNA bits on each side (red). (B) After we made copies of just the GFP DNA using PCR, we could use that DNA in C. elegans so that certain cells of the worm fluoresced with a green light. Illustration by: Iris Gat.
As it turned out, we chose the right strategy. Ghia used PCR to copy the GFP DNA. She then incorporated this GFP DNA into a DNA molecule called a plasmid, which bacteria “swallow” and integrate as their own DNA. After shining blue light on the E. coli containing GFP DNA, they fluoresced [6]. (In contrast, the other groups that used the original GFP DNA with the additional DNA did not see fluorescence. Something in the extra DNA interfered with the production of GFP. If we had not used PCR, our experiment would not have worked). Since our method worked in bacteria, we next used it to incorporate GFP DNA into C. elegans, as I originally dreamt of doing—and we made these worms glow as well (Figure 2B). This paved the way for incorporating GFP into all sorts of organisms and using it for various applications.
How GPF Changed Science
Any new scientific development can give us a better understanding of basic biological or physical principles, and can also help in the creation of new technologies. Many times, the original discovery develops in surprising and unexpected directions over time. The discovery of the laser is a good example. Charles Townes, whose work led to the laser, never imagined it would be useful in grocery stores for scanning the prices of products, or in the record industry for making compact disks, or in the movie business for making DVDs, or in medicine for performing laser surgeries. The same holds true for the discovery of GFP—it has evolved, and will continue to evolve, in many different directions that advance both fundamental scientific knowledge and various technological applications.
In scientific research, GFP can be used as a biological marker to tell us about the action of genes and their products. Genes can be thought of as having two parts: the coding part, which indicates what product should be made (the RNA and protein) and the regulatory part that says where, when, and how much of the product should be made. If the GFP DNA sequence added to just the regulatory part, GFP will be made and fluoresce whenever the normal gene is “turned on.” Alternatively, if the GFP DNA sequence is added to the coding sequencing, the normal protein will have GFP protein attached to it, and it will glow when we shine blue light on it [7]. We can see where the proteins reside in cells (e.g., in the nucleus, at the cell membrane) and also watch them as they move through living cells, a process that was not possible with earlier methods. Importantly, once the GFP DNA is inserted into the DNA of an organism, it can be passed on to that organism’s offspring—this makes the experimental use of GFP very convenient.
I would like to briefly mention two discoveries made using GFP that I personally admire. The first was made by Clifford Brangwynne and Anthony Hyman in 2009 [8]. They were looking at proteins within the cytoplasm—the liquid that fills up the space within cells. Until then, people thought that the cytoplasm was quite uniform, but when these scientists looked at a particular cytoplasmic protein labeled with GFP, it appeared to be contained in particles that were separated from the rest of the cytoplasm. These particles acted like tiny drops of oil in water—sometimes they would come together and fuse, and other times they would split into two. These structures did not mix with the rest of the cytoplasm, they were a separate phase (they are often called phase-separated particles (Figure 3A). This discovery turned out to be exceptionally important for our understanding of the structure and function of cells, and it opened up a very active area of research.
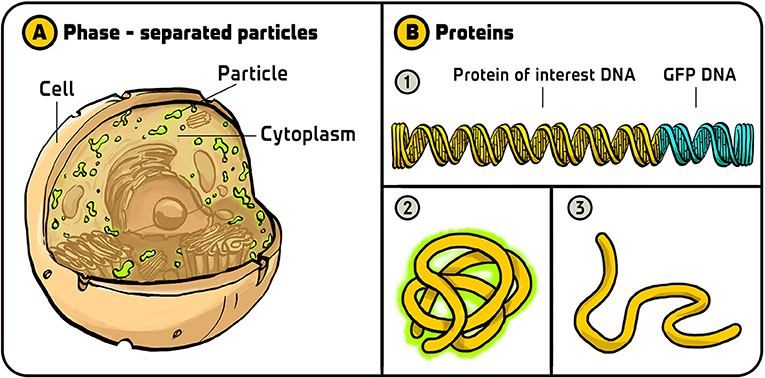
- Figure 3 - Uses of GFP.
- (A) Using GFP, Clifford Brangwynne and Anthony Hyman found that the cytoplasm of cells contains two separate phases—the well-known liquid phase and another phase that behaves like oil particles in water. (B) Geoff Waldo used GFP to monitor the folding of proteins. (1) He added GFP DNA to the end of the DNA coding for a protein he was interested in. (2) If the protein (including the GFP part) folded correctly, it would fluoresce in green. (3) If the protein folded incorrectly, it would not fluoresce. Illustration by: Iris Gat.
Another use of GFP that I particularly like was done by Geoff Waldo [9]. Geoff found a clever way of using GFP to monitor the folding of proteins; he called it a “folding reporter.” After a protein is produced as a long chain of building blocks called amino acids, it must fold into a specific three-dimensional structure to perform its function. If a protein folds incorrectly, it will not work properly. When we study proteins by making them in bacteria, for example, we want to make sure that they are folded properly. Geoff constructed a DNA that encoded both the protein he was interested in and GFP. He reasoned that if the protein of interest did not fold properly, then the GFP would also not fold properly and this would not glow. So, if he saw fluorescent cells, he could conclude that the protein of interest folded correctly, and if there were no fluorescent cells, he could conclude that the protein folded incorrectly. This is a very nice way for scientists to ensure that they are working with properly folded proteins (Figure 3B).
There are many other uses for GFP, so I will touch on just a few more. Some people use GFP to study how viruses infect cells. One notable study used GFP to color HIV, the virus that causes AIDS, to study how the virus spreads from one cell to another [10]. In this study it was found that HIV viruses can move between cells without destroying the cells (as many other viruses do). This discovery has implication as to how the transfer of the virus from one cell to another could be controlled. Other groups are studying how to use GFP to detect land mines and the residues of explosives [11]. In Japan, people even use GFP to produce silk that glows green [12]. There are many more interesting uses of GFP, and of other fluorescent proteins with other colors that scientists have found or have generated. These examples give you an initial glimpse of just how useful fluorescent proteins can be.
Recommendations for Young Minds
I do not think that there is a “recipe” for doing important scientific research, but there are things that I believe to be important. One of the most important abilities of a successful scientist is the ability to ask questions. Many great questions are sitting right under our noses, waiting to be asked, but if we are not in the habit of asking questions, we might miss them. Asking question is an excellent way to understand things. When you learn something new, ask yourself how the new thing you learned might apply to other topics you are interested in. This is an important approach that I use in my work all the time. Another important aspect of asking questions is questioning your assumptions (Figure 4) — why do you believe what you believe? Quite often, when we investigate our assumptions in this way, we find errors in our assumptions. Correcting these errors by updating our assumptions can help us to advance our knowledge and understanding.
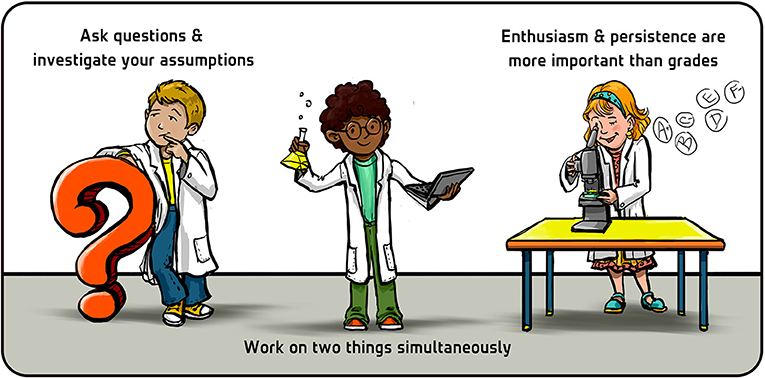
- Figure 4 - Three recommendations for young minds.
- Illustration by: Iris Gat.
I often recommend that students work on two things at the same time. This way, if one project is not working, they still have the other to draw success and motivation from. Science is a journey of grappling with the unknown. It is not always easy and may be frustrating, and we make lots of errors along the way. Our ideas do not always work, but making headway against the unknown is an exciting part of being a scientist. Occasionally, this challenging journey brings great rewards—like discovering something that no one else has known before, and then sharing that discovery with others.
Finally, do not worry too much about your grades. Grades can have absolutely nothing to do with success in science. I think that enthusiasm and persistence are far more important than grades. I got average grades (C’s) in chemistry when I was in university, and then, somehow, was awarded a Nobel Prize in Chemistry some 30 years later (I enjoy the irony of that). If you are interested in science, one of the best ways to become good at it is to do it. Try doing experiments and see what it is like—this is the most reliable way to know if science is the right choice for you.
Glossary
Bioluminescence: ↑ The production of light by living organisms.
Aequorin: ↑ A blue glowing protein that was discovered in the Aequorea victoria jellyfish by Prof. Osamu Shimomura.
Fluorescent Molecules: ↑ Molecules that convert one color of light (blue, for example) to another color (green, for example).
Green Fluorescent Protein (GFP): ↑ A protein that was first identified in A. victoria jellyfish. GFP absorbs blue light and converts it to green light.
Nerve Cells: ↑ Cells of the nervous system that receive information from the environment, process it using electrical and chemical signals, and generate an output, such as movement.
Biological Marker: ↑ A biological molecule used by researchers to indicate a biological process or state.
Polymerase Chain Reaction (PCR): ↑ A laboratory method used for creating many copies of a specific segment of DNA using a DNA-copying enzyme called DNA polymerase.
Gene: ↑ A segment of DNA that carries the instructions for making a specific protein.
Acknowledgments
I wish to thank Noa Segev for conducting the interview which served as the basis for this article and for co-authoring the article, Iris Gat for providing the figures (https://www.irisgat-art.com/), and Susan Debad for copyediting the manuscript.
Additional Materials
- The five myths about scientists according to Nobel Laureate Martin Chalfie
- Martin Chalfie, Nobel Laureate, on Failed and Useless
Conflict of Interest
The author declares that the research was conducted in the absence of any commercial or financial relationships that could be construed as a potential conflict of interest.
References
[1] ↑ Shimomura, O., Johnson, F. H., and Saiga, Y. 1962. Extraction, purification and properties of aequorin, a bioluminescent protein from the luminous hydromedusan, Aequorea. J. Cell. Compar. Physiol. 59:223–39. doi: 10.1002/jcp.1030590302
[2] ↑ Shimomura, O. 2009. Discovery of green fluorescent protein (GFP) (Nobel Lecture). Angew. Chem. Int. Ed. 48:5590–602. doi: 10.1002/anie.200902240
[3] ↑ Morin, J. G., and Hastings, J. W. (1971). Energy transfer in a bioluminescent system. J. Cell. Physiol. 77:313–8. doi: 10.1002/jcp.1040770305
[4] ↑ Prasher, D. C., Eckenrode, V. K., Ward, W. W., Prendergast, F. G., and Cormier, M. J. 1992. Primary structure of the Aequorea victoria green-fluorescent protein. Gene 111:229–33. doi: 10.1016/0378-1119(92)90691-H
[5] ↑ Mullis, K., Faloona, F., Scharf, S., Saiki, R., Horn, G., and Erlich, H. 1992. Specific enzymatic amplification of DNA in vitro: the polymerase chain reaction. Biotechnol. Ser. LI:17.
[6] ↑ Chalfie, M., Tu, Y., Euskirchen, G., Ward, W. W., and Prasher, D. C. 1994. Green fluorescent protein as a marker for gene expression. Science 263:802–5. doi: 10.1126/science.8303295
[7] ↑ Wang, S., and Hazelrigg, T. 1994. Implications for bcd mRNA localization from spatial distribution of exu protein in Drosophila oogenesis. Nature 369:400–3. doi: 10.1038/369400a0
[8] ↑ Brangwynne, C. P., Eckmann, C. R., Courson, D. S., Rybarska, A., Hoege, C., Gharakhani, J., et al. 2009. Germline P granules are liquid droplets that localize by controlled dissolution/condensation. Science 324:1729–32. doi: 10.1126/science.1172046
[9] ↑ Waldo, G. S., Standish, B. M., Berendzen, J., and Terwilliger, T. C. (1999). Rapid protein-folding assay using green fluorescent protein. Nat. Biotechnol. 17:691–5. doi: 10.1038/10904
[10] ↑ Hübner, W., McNerney, G. P., Chen, P., Dale, B. M., Gordon, R. E., Chuang, F. Y., et al. 2009. Quantitative 3D video microscopy of HIV transfer across T cell virological synapses. Science 323:1743–7. doi: 10.1126/science.1167525
[11] ↑ Shemer, B., Palevsky, N., Yagur-Kroll, S., and Belkin, S. 2015. Genetically engineered microorganisms for the detection of explosives’ residues. Front. Microbiol. 6:1175. doi: 10.3389/fmicb.2015.01175
[12] ↑ Shimizu, K. 2018. Genetic engineered color silk: fabrication of a photonics material through a bioassisted technology. Bioinspir. Biomimet. 13:041003. doi: 10.1088/1748-3190/aabbe9