Abstract
Our life experience is shaped by our senses. We see, hear, smell, touch, and taste the things around us, and this is how we get to know the world. In my research, I study receptors—small sensing structures present on cell membranes that react to stimuli from the environment or from within the body. The receptors I tell you about in this article are sensitive to pain and heat, and they respond both to high temperatures and to spicy substances, such as hot chili peppers. We believe that, by studying the structures of these receptors and gaining a better understanding of how they operate, we will be able to develop new drugs to treat long-lasting pain more effectively and safely. Read this article to learn how the chili pepper has opened the door for us to uncover some great mysteries of the sensation of pain, paving the way toward future pain treatments.
As background materials for this article, it is recommended to view two previously published articles on the patch clamp technique and on cryogenic electron microscopy.
Professor David Julius won the Nobel Prize in Physiology or Medicine in 2021, jointly with Prof. Ardem Patapoutian, for their discoveries of receptors for temperature and touch.
Does It Hurt for a Reason?
For all animals, including humans, pain is a very important sensation. Pain tells us when we are injured and need to take care of ourselves, and when we should stop or avoid a potentially harmful activity. In scientific terms, pain is part of somatosensation—the ability to sense touch, temperature, pain, and our limbs position and movement in the space around us. Somatosensation is performed by small sensing structures that are spread throughout the body, which we call receptors. Receptors located on the membrane of nerve cells respond to stimuli from the environment (e.g., hot liquid in a cup) and, consequently, these somatosensory nerve cells send signals to the relevant brain regions, where these signals are processed and deciphered (Figure 1).
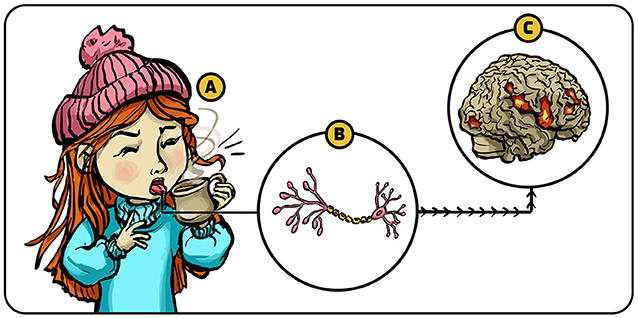
- Figure 1 - Somatosensation.
- (A) Stimuli like temperature and pain are sensed by tiny structures called receptors that are located on the membrane surface of special nerve cells (located in our tongue in this example). (B) When these receptors sense the relevant stimulus, they generate electrical signals in the nerve cell which is then sent to the brain. (C) Specific regions in the brain interpret these signals—in this example, letting the girl know that the liquid she drinks is hot.
In this article, we will focus on one type of receptors called ion channel receptors. These receptors use ion channels—small “tunnels” or “gates” present in cell membranes—to create electrical signals in response to stimuli from the environment (to learn more about ion channels, read this Nobel Collection article). Ion channel receptors are very complicated and fascinating signaling machines that lie at the heart of our ability to sense our internal and external environments. Over the years, scientists developed advanced tools for studying the structures and functions of ion channel receptors. For example, we can use a special imaging method called cryogenic electron microscopy, where we send electrons through frozen specimens, to take highly detailed pictures of these receptors, and can build three-dimensional models of them. We can also use a sophisticated method called patch clamp, to measure electrical charges that flow through these ion channels and this enables us to study the electrical behavior of ion channel receptors.
These methods help us to answer basic scientific questions about how people sense pain, which can further help us to develop new drugs for pain relief. When pain-sensitive ion channel receptors work properly, they help people protect themselves against physical harm. However, occasionally something goes wrong with this protective mechanism, and it gets out of control. Then people might experience chronic pain—pain that is persistent and does not seem to have a useful protective function. So far, conventional pain-relief medications, called analgesics (or painkillers, such as Aspirin), have been very efficient at treating acute pain, but not chronic pain. In addition, common analgesics (painkillers) like morphine are addictive and can have undesired side effects, including dizziness, nausea, and vomiting [1].
Overuse of addictive analgesics has contributed to what is known as the opioid crisis, during which many people have become addicted to analgesics, abusing them in ways that harm their health. We hope that a better understanding of pain receptors—particularly ion channel pain receptors—will help us to develop new analgesics that are more effective at treating chronic pain. Such new pain medicines will likely help us address the opioid crisis. Before we look into the new medicines that might be developed from pain receptor research, I will first tell you a little about the family of pain receptors that my colleagues and I discovered.
Hot Chili Peppers and Pain Receptors
I was always fascinated by how the senses operate and by the ways that certain chemicals, especially natural products, affect the brain and the body. Through my research, I could combine these two fascinations to better understand how somatosensation works. I specifically wanted to understand how humans detect stimuli that might be harmful to the body—through a process called nociception.
During the 1970s, scientists found that capsaicin—the ingredient in hot chili peppers that causes the burning sensation—evokes a sensation of pain in the eye of rats by activating nociception receptors in specific sensory nerve endings [2]. A decade later, scientists suggested that capsaicin evokes pain by allowing ions, including calcium ions, to flow into the sensory neurons that are involved in nociception [3]. These finding gave me and my students the hints we needed to tackle the following questions: Is there a specific receptor for capsaicin in sensory nerve cells? If so, what does it look like, and what role does it have in the ability to sense pain?
To answer this question, my students and I decided to search for the gene that encoded the specific protein of the capsaicin receptor. Because we did not know what that receptor—or its respective gene—looked like, we had to search for them using only of few clues to guide us. We knew (according to the central dogma of molecular biology) that the production of any protein, including pain receptors, involves the generation of a molecule called mRNA, which carries the instructions from the DNA that are needed to make that protein. Our strategy was to take nerve cells that respond to pain (Figure 2A), isolate the mRNA that codes for the pain receptors (Figure 2B), and then transform this mRNA back into the DNA instructions (the gene) that codes for the pain receptor (Figure 2C) [4] (to learn more about mRNA, read this Nobel Collection article; and to learn more about how mRNA can be transformed into DNA, read this Nobel Collection article).
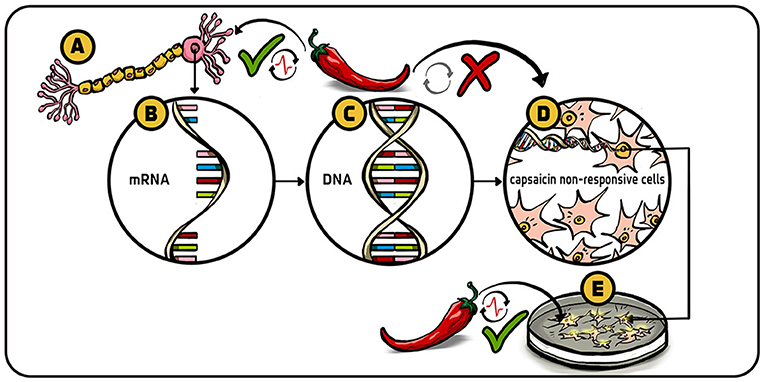
- Figure 2 - Looking for the capsaicin receptor gene.
- (A) Receptor neuron, called nociceptor, that responds to capsaicin and eventually transmits pain information to the brain. (B) We removed from these cells the mRNA that we suspected to be involved with coding for the receptor-sensitive to capscaicin. (C) We then turned each of the many mRNA molecules that we screened back into the DNA (genes) that they were initially made from. This DNA library should include a DNA fragment or several fragments encoding the protein capable of reacting to capsaicin. (D) The DNA fragments were introduced into cells that normally did not respond to capsaicin. (E) The cells that did respond to capsaicin after the DNA was introduced to them were then known to contain the capsaicin gene. After a lot of screening, we identified the single gene that codes for the capsaicin receptor.
Toward this goal we created a library of millions of DNA fragments corresponding to genes that are expressed in the sensory neurons which can react to pain, heat, and touch. To find the specific gene we were looking for, we had to screen hundreds of thousands of DNA pieces produced from many different mRNAs collected from the pain-responsive nerve cells. This was a laborious process that involved sophisticated ways of dividing the enormous number of DNA pieces that we created into smaller and smaller groups, until we could finally detect the exact gene we were interested in.
To check whether we found the right gene, we put our DNA pieces that we suspected code for the pain receptor into cells that originally did not respond to capsaicin (Figure 2D). We then checked whether these cells became sensitive to capsaicin by using a method invented by a late Nobel Laureate named Roger Tsien, with which we could actually see a glowing flash of light from the cells every time calcium ions entered into the cell (Figure 2E). We then used the patch clamp technique to record current flow across small patches of membrane from these glowing cells and confirmed that they responded to capsaicin by enabling the flow of ions into the cell—bingo!
Our results told us that there is a capsaicin receptor, and that it is an ion channel. We named it the TRPV1 receptor (Figure 3A), as it is a member of a group of ion channels called the transient receptor potential (TRP) channels. Later, we found that this receptor responds not only to capsaicin (Figure 3B) but also to harmful heat—it becomes activated at temperatures above 43°C (Figure 3C) [4].
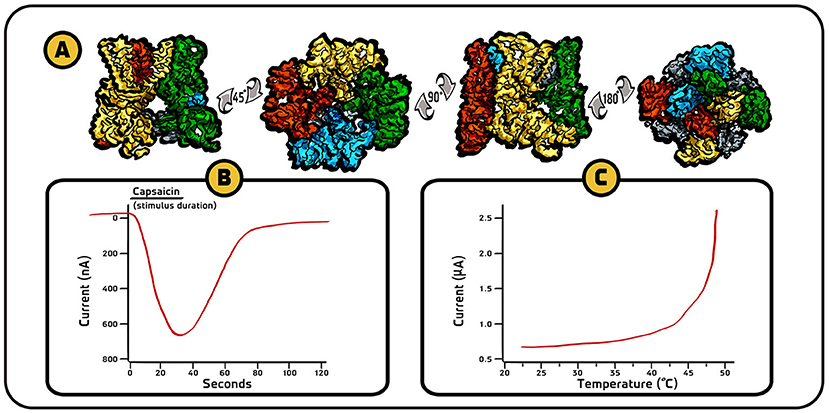
- Figure 3 - Structure and behavior of TRPV1 receptor.
- (A) High-resolution three-dimensional drawings in four different views of TRPV1 receptor channel, created using images obtained from cryogenic electron microscopy (images adapted from [5]). (B) In response to capsaicin, TRPV1 receptors allow calcium ions (which are positively charged) to enter the cell, as expressed by a change in electrical current. When a positive charge flows into the cell, the convention is that the current is displayed in the downward direction (graph adapted from [4]). (C) TRPV1 receptors also respond to high temperatures by allowing calcium and sodium ions (positive charge) to flow into the cell. These results tell us that the same receptors respond both to chemicals (capsaicin in this case) and to hot temperatures which can be harmful for the body.
After finding the TRPV1 receptor, it was easier for us to discover additional receptors from the TRP family, such as TRPM8, which responds to menthol and cold temperatures [6], and TRPA1 (sometimes called the wasabi receptor), which responds to pungent agents from mustard and garlic, and agents produced during inflammation, among other stimuli [7]. TRP channels are found in a lot of sensory tissues, including the eyes, tongue, and skin. Many of them are involved in detecting external signals that are related to our senses, and some of them detect signals from within the body (such as signals generated by internal organs). Our understanding of their structures and functions have greatly advanced, but there are still some riddles yet to be solved.
The Future of Pain Research
One of the main riddles we are still trying to solve is how heat and cold activate TRP receptors. Currently, we do not understand how TRP receptors “sense” temperature. We think that, as opposed to chemicals like capsaicin, temperature does not act on one specific part of the TRP receptor but instead acts on multiple parts simultaneously. To study this, we are trying to freeze and take images of receptors (using cryogenic electron microscopy) as they respond to hot or cold temperatures, and then try to understand what those temperatures are doing to the receptors.
To develop better drugs for acute and chronic pain, which could begin to address the opioid crisis, I believe we need to understand the various types of pain, including the molecules, cell types, and pathways that are most involved in each type (for example, we know that pain that is perceived in the skin, called cutaneous pain, is very different from pain that is generated by internal organs, called visceral pain). I do not think we can solve every type of pain using the same approach, so we will probably have to develop specific approaches for each type of pain. We must also develop better ways to measure pain, since everyone feels pain differently. Accurate pain measurements will allow us to better assess the performance of new drugs.
I am also interested in diving deeper into the atomic structure of TRP receptors, and in using this knowledge to better understand how they operate. An enhanced understanding of how TRP receptors work will allow us to develop new drugs that target only specific parts or mechanisms of these receptors. Many analgesics used today block pain receptors completely and therefore interfere with the receptors’ ability to warn people against harm. For example, some TRPV1 drugs diminish people’s ability to detect painful heat, and as a result patients could unknowingly burn themselves when touching something hot or drinking hot liquids [8].
Is it possible to develop drugs that do not shut TRP receptors down, but instead change the way the receptors interact with a specific stimulus? In the case of TRPV1, we are looking for ways to block the ability of inflammation-causing substances to increase the sensitivity of the receptor—without blocking its ability detect heat under normal conditions. This is a challenging task, and a lot of work is still ahead of us—but the great potential of helping many people that suffer from chronic pain makes the effort worthwhile.
Recommendations for Young Minds
Science is like any kind of creative endeavor—you have the freedom to follow your curiosity, but there is also a lot of unpredictability along the way. You can forge your own path and get great enjoyment from your work, but there are also times of frustration and anxiety, when you do not understand your results or what you should do next. If you choose a career in science, you must be driven by curiosity and passion, enjoy understanding how things work, and have real motivation for solving puzzles. Often you will have to simply put one foot in front of the other and pay attention to the short term, enjoying coming into the lab and doing your experiments.
I often tell my students that persistence pays off. You must find a way to keep advancing toward a scientific goal that you find exciting, interesting, and significant. In my career, I focused on big questions that had been unanswered for many years, that would be very exciting to solve, and which could open up new possibilities in my field of research. When things were not working for me, I tried to figure out why and I searched for a new approach. As long as I could think of something new to try, I had the energy to go back to the lab and look at the problem differently. This path was challenging, but my persistence paid off.
Finally, I want to highlight another unusual and exciting aspect of science: scientists are part of an international community (Figure 4). I know people from all over the world and I visit them frequently. I think this is something very special and unique and it has really broadened my horizons, making my life even more interesting.
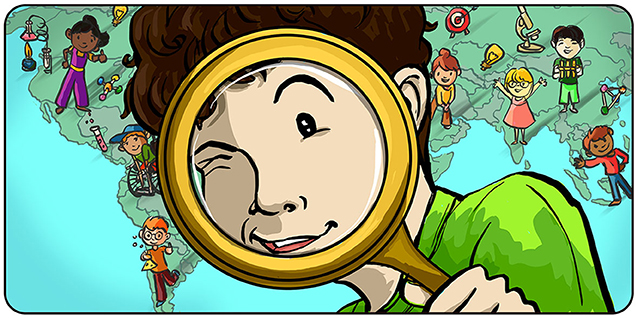
- Figure 4 - Scientists are part of an international community.
- One of the things I love about being a scientist is that I am part of an international community of many scientists. Knowing people from all over the world enriches me and makes my life more interesting.
Glossary
Somatosensation: ↑ The ability to sense touch, temperature, pain, and the position and movement of our bodies in space.
Receptors: ↑ Small sensing units, typically proteins in the cell’s membrane, that respond to stimuli (such as specific chemicals or temperature).
Ion Channel Receptors: ↑ Receptors that create electrical signals in response to stimuli, by allowing ions (charged particles) to flow into and out of nerve cells.
Chronic Pain: ↑ Pain that lasts for long periods of time (more than 12 weeks), even after the original cause is gone. Examples: chronic abdominal pain and chronic pain in the joints.
Analgesics: ↑ Drugs that are designed to relief pain (“painkillers”).
Acute Pain: ↑ Sudden pain that lasts for a relatively short time and passes when its original cause is gone (for example, the pain you feel when you cut your finger).
Nociception: ↑ The process in the sensory system that enables to detect harmful (noxious) stimuli.
Capsaicin: ↑ The spicy substance in hot chili peppers that causes the burning sensation.
Conflict of Interest
The author declares that the research was conducted in the absence of any commercial or financial relationships that could be construed as a potential conflict of interest.
Acknowledgments
I wish to thank Noa Segev for conducting the interview which served as the basis for this paper and for co-authoring the paper; Iris Gat for providing the figures; and Susan Debad for copyediting the manuscript.
References
[1] ↑ Benyamin, R., Trescot, A. M., Datta, S., Buenaventura, R. M., Adlaka, R., Sehgal, N., et al. 2008. Opioid complications and side effects. Pain Phys. 11:S105. doi: 10.36076/ppj.2008/11/S105
[2] ↑ Szolcsányi, J., and Jancso-Gabor, A. 1975. Sensory effects of capsaicin congeners I. Relationship between chemical structure and pain-producing potency of pungent agents. Arzneimittel Forschung. 25:1877–81.
[3] ↑ Wood, J. N., Winter, J., James, I. F., Rang, H., Yeats, J., and Bevan, S. 1988. Capsaicin-induced ion fluxes in dorsal root ganglion cells in culture. J. Neurosci. 8:3208–20. doi: 10.1523/JNEUROSCI.08-09-03208.1988
[4] ↑ Caterina, M. J., Schumacher, M. A., Tominaga, M., Rosen, T. A., Levine, J. D., and Julius, D. 1997. The capsaicin receptor: a heat-activated ion channel in the pain pathway. Nature. 389:816–24. doi: 10.1038/39807
[5] ↑ Liao, M., Cao, E., Julius, D., and Cheng, Y. 2013. Structure of the TRPV1 ion channel determined by electron cryo-microscopy. Nature. 504:107–12. doi: 10.1038/nature12822
[6] ↑ Bautista, D. M., Siemens, J., Glazer, J. M., Tsuruda, P. R., Basbaum, A. I., Stucky, C. L., et al. (2007). The menthol receptor TRPM8 is the principal detector of environmental cold. Nature. 448:204–8. doi: 10.1038/nature05910
[7] ↑ Bautista, D. M., Jordt, S. E., Nikai, T., Tsuruda, P. R., Read, A. J., Poblete, J., et al. 2006. TRPA1 mediates the inflammatory actions of environmental irritants and proalgesic agents. Cell. 124:1269–82. doi: 10.1016/j.cell.2006.02.023
[8] ↑ Moran, M. M., and Szallasi, A. 2018. Targeting nociceptive transient receptor potential channels to treat chronic pain: current state of the field. Br. J. Pharmacol. 175:2185–203. doi: 10.1111/bph.14044