Abstract
Most of the world’s rich variety of life, called biodiversity, cannot be seen by the naked eye. But just because it is small does not mean it is boring or unimportant! Tiny organisms known as microbes, which are often made up of only a single cell, have many impressive abilities and weird qualities. A lot of the microbial diversity we observe today was made possible by the sharing of DNA between different species through a process called endosymbiosis—in which one organism (the endosymbiont) becomes trapped inside another (called the host). Indeed, an ancient endosymbiotic event resulted in the chloroplasts that trees and green algae use to convert sunlight into energy, for example. Chloroplasts were then passed along, like Pokémon cards, to other organisms through more recent endosymbiotic events, causing an explosion of photosynthesis across the microbial world.
Microbes Rule the World
There are millions of species on Earth, most of which are microbes and cannot be seen by the naked eye. All of this diversity comes in two main flavors: prokaryotes and eukaryotes (Figure 1). Animals, plants, and fungi, for example, are eukaryotes, which means their cells contain sub-parts known as organelles. Organelles are surrounded by membranes (barriers that let only certain things in and out) and they perform specific tasks that keep cells alive and running. Organelles set eukaryotes apart from “simpler” cells known as prokaryotes. Whereas, eukaryotes can be made of one cell or many cells, prokaryotes are almost exclusively one-celled (unicellular) organisms. Bacteria are classic examples of prokaryotes. Another key difference between eukaryotes and prokaryotes is that eukaryotes have an organelle called the nucleus, which encloses their DNA, whereas prokaryotes do not have a nucleus, meaning their DNA is more “free floating” inside the cell. Through a microscope, eukaryotic cells are generally much larger and more complex than prokaryotic cells (Figure 1).
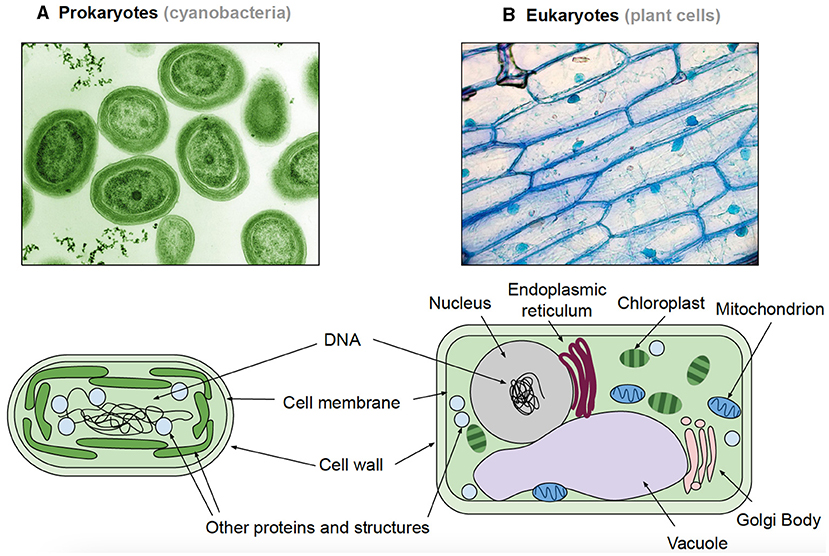
- Figure 1 - (A) Prokaryotes, like cyanobacteria, are generally smaller and less complex than eukaryotic cells.
- They do not have a nucleus enclosing their DNA. (B) Eukaryotes, like these plant cells (stained blue), have various membrane-bound structures called organelles that perform important functions inside the cell. They contain a nucleus where the DNA is kept. Eukaryotic cells are typically around 100 times bigger than prokaryotic cells—about the size difference between a grain of rice and a beach ball (Photo credits: Wikipedia Commons).
There are millions of eukaryotic species on Earth [1], and most of them are not animals or plants—they are microbes. Much of this microscopic biodiversity exists because eukaryotic microbes love eating each other. And in the case of microbes, sometimes you really are what you eat!
In this article, we focus on plastids—a type of organelle found in some eukaryotic cells. Plastids produce and store chemicals required for survival. The chloroplasts found in plants and algae are a kind of plastid that converts sunlight into sugar to be used as energy, a process known as photosynthesis. Plastids are also responsible for much of the biodiversity seen on Earth today, especially among eukaryotic microbes.
Before Plants and Animals
Long ago, before animals and plants existed, microbes dominated Earth’s oceans, lakes and ponds (Figure 2A). This was the case for most of Earth’s history. Much like animals today, eukaryotic microbes needed to eat to gain energy for survival. No eukaryote could use sunlight to produce its own energy—only some bacteria, including a type called cyanobacteria (Figure 1A), could perform photosynthesis.
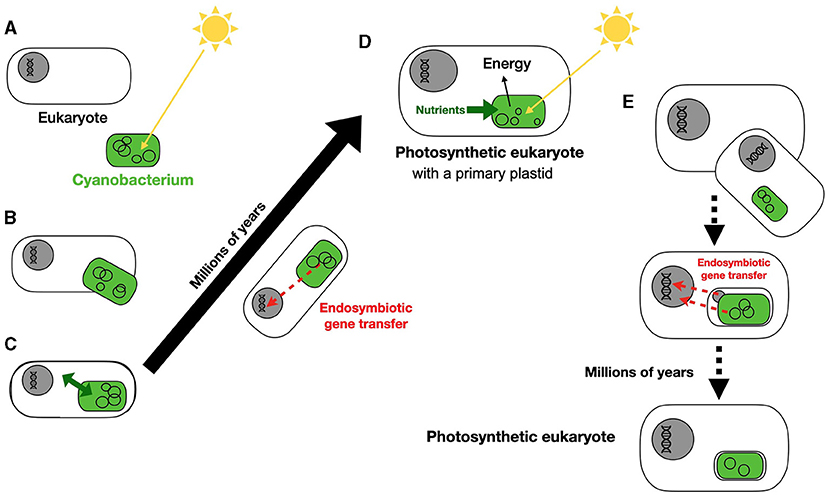
- Figure 2 - (A) A eukaryote and a cyanobacterium living independently billions of years ago.
- Cyanobacteria convert sunlight into energy using photosynthesis. (B) A eukaryote engulfs a cyanobacterium. (C) Primary endosymbiosis, in which the bacterium receives protection and nutrients in return for providing energy from photosynthesis. Over millions of years, endosymbiotic gene transfer (and gene loss) causes the transfer of endosymbiont genes to the host’s genome. (D) The endosymbiont is eventually an organelle—a primary plastid. (E) Higher-order endosymbiosis involves a eukaryote without a plastid engulfing another eukaryote with a primary plastid, in this case a chloroplast that can perform photosynthesis.
More than a billion years ago, a eukaryote engulfed a photosynthetic cyanobacterium (Figure 2B) [2]. Usually, this is no big deal—many eukaryotes hunt and engulf their prey, digest it, extract nutrients, and go on with their day. But not this time! For various reasons, this particular cyanobacterium was not digested. In fact, over time it continued to live inside the eukaryote. This type of relationship is called endosymbiosis because one organism (called the endosymbiont) lives inside another (called the host) and can result in each one benefiting from the other. In this case, the eukaryote protected and fed the cyanobacterium and, in return, the cyanobacterium provided the eukaryote with energy produced via photosynthesis (Figure 2C). As the host reproduced (dividing into new cells), the endosymbiont remained inside the host and divided too. All the cells that were descendants of the host contained an endosymbiont that produced energy for them. Eventually, over millions of years, the descendants of this cyanobacterium slowly lost all independence and gradually changed from an endosymbiont (a separate organism) to just another part of the eukaryotic cell—an organelle we now call a plastid (Figure 2D).
This event is known as primary endosymbiosis because it involved a prokaryote (the cyanobacterium) and gave rise to a primary plastid. Scientists believe mitochondria evolved by a similar process, where an ancestor of eukaryotes engulfed a bacterium, which eventually became the organelle we call a mitochondrion. However, this mitochondria primary endosymbiosis happened much longer ago than plastid primary endosymbiosis. Three main groups of life arose from this primary plastid endosymbiotic event: red algae, green algae plus land plants, and a group of freshwater algae called glaucophytes (Figures 3A–F) [2, 3]. Organisms in these three groups all contain a primary plastid. In other words, without primary endosymbiosis, plants and algae would not exist!
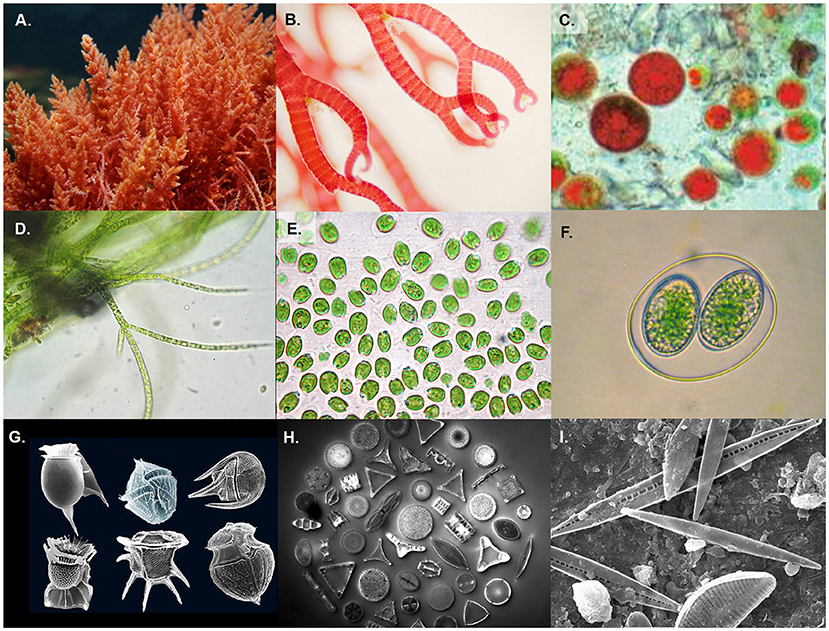
- Figure 3 - (A–C) Examples of multicellular and unicellular red algae (Photo credits: Encyclopædia Britannica; Wikipedia Commons; Instruments Direct Services Limited).
- (D, E) Examples of multicellular and unicellular green algae (Photo credit: Encyclopædia Britannica; Stevens Point Department of Biology, University of Wisconsin). (F) A glaucophyte, freshwater algae with primary plastids (Photo credit: Wikipedia Commons). (G) Dinoflagellates seen through an electron microscope (Photo credit: Flickr). (H, I) Diatoms seen through an electron microscope (Photo credit: Randolph Femmer, USGS Library of Images From Life; Wikipedia Commons).
No Eukaryote Is Safe
Plastid endosymbiosis can also occur between eukaryotes. For example, red and green algae which contain plastids, are often gobbled up as food by other kinds of eukaryotes. So—you guessed it—certain red and green algae have evolved (via endosymbiosis) from being food to actual working chloroplasts housed inside of other diverse organisms.
This process is known as higher-order plastid endosymbiosis (Figure 2E). When eukaryotes ultimately become plastids in another eukaryote, these plastids are known as complex plastids. One of the main features of complex plastids is that they have more than two membranes, unlike primary plastids. But the most important takeaway is that higher-order endosymbiosis has allowed photosynthesis to spread across the eukaryotic Tree of Life into some really interesting organisms, ones that you would not necessarily think have plastids [3] (Figure 3).
You Are What You Engulf
Among the many benefits of endosymbiosis can be the reciprocal exchange of energy and nutrients between the host and the endosymbiont. But this close relationship can also end up mixing the DNA of both organisms, in a process known as endosymbiotic gene transfer [4]. Simply put, this involves the movement of the endosymbiont’s DNA to the host’s genome, much like transferring information from an external drive to a desktop computer.
Endosymbiotic gene transfer is perhaps the key ingredient in the journey from endosymbiont to organelle, as it allows the host to gain more and more control over its cellular resident. Another important ingredient is the outright loss of genes from the endosymbiont, causing it to become more and more dependent on the host. All of this gene blending and gene loss can sometimes lead to very unusual genetic features. Some diatom algae, for example, have special plastids that allow them to produce chemicals that help them survive in even the harshest conditions, like in the Antarctic Ocean where the water is very poor in iron (an essential ingredient for most microbial life) [5].
Even More Diversity!
Not all plastid endosymbiotic events are successful, and sometimes it takes a few tries to get it right [3, 6]. This means that some algae bear the marks of older higher-order plastid endosymbioses that were either halted before completion or were successful but the plastid was ultimately lost. Think of it like installing a solar panel on a house and the workmen stop the job before completion or the panel gets blown away during a storm. Whatever the outcome, the house will often contain the “scars” of the initial installation in the form of marks on the roof, etc. It is similar with the genomes of certain eukarytoes in that they contain pieces of DNA from old plastid endosymbiotic events [6].
The genomes of some diatom algae (Figure 3) can be a great example of this, containing a random mash-up of dozens of DNA pieces from numerous organisms. Imagine trying to make sushi with a recipe that contains instructions for pasta sauce and tacos! But for diatoms, this mash-up works like a charm. In fact, diatoms are among the most abundant and diverse groups of algae on Earth [6]. Some believe that hundreds, or even thousands of new genes and gene combinations have evolved from the blending of genomes, resulting from endosymbioses, paving the way for new abilities and new species adapted for living in even the harshest environments.
We could go on for another hundred pages describing the many amazing features and outcomes of plastid endosymbiosis. There are millions of species of eukaryotic microbes, each one with unique characteristics and tangled evolutionary histories. In summary, not only is endosymbiosis a fascinating biological process, but without it much of the massive biodiversity we see today would not exist.
Glossary
Eukaryotes: ↑ Organisms with complex cell(s) that contain organelles with membranes, a nucleus to hold DNA, and other intricate characteristics.
Biodiversity: ↑ The variety of living things in the world or in a certain area.
Plastid: ↑ A type of organelle that evolved from an endosymbiont. Plastids produce essential chemicals (such as sugars) used by the cell for energy.
Endosymbiont: ↑ An organism that lives inside another organism, often involving the trading of resources.
Primary Endosymbiosis: ↑ The initial event more than a billion years ago when a prokaryote known as a cyanobacterium was engulfed by a eukaryote and eventually became an organelle known as a plastid.
Primary Plastid: ↑ A plastid that evolved from primary endosymbiosis.
Higher-order Plastid Endosymbiosis: ↑ Endosymbiosis involving eukaryotes, where one eukaryote becomes the plastid of another eukaryote.
Endosymbiotic Gene Transfer: ↑ When genes from an endosymbiont are transferred to the host’s own genome.
Conflict of Interest
The authors declare that the research was conducted in the absence of any commercial or financial relationships that could be construed as a potential conflict of interest.
References
[1] ↑ Mora, C., Tittensor, D. P., Adl, S., Simpson, A. G. B., and Worm, B. 2011. How many species are there on earth and in the ocean? PLoS Biol. 9:e1001127. doi: 10.1371/journal.pbio.1001127
[2] ↑ Burki, F., Roger, A. J., Brown, M. W., and Simpson, A. G. B. 2019. The new tree of eukaryotes. Trends Ecol. Evol. 35:43–55. doi: 10.1016/j.tree.2019.08.008
[3] ↑ Sibbald, S. J., and Archibald, J. M. 2020. Genomic insights into plastid evolution. Genome Biol. Evol. 12:978–90. doi: 10.1093/gbe/evaa096
[4] ↑ Timmis, J. N., Ayliffe, M. A., Huang, C. Y., and Martin, W. 2004. Endosymbiotic gene transfer: organelle genomes forge eukaryotic chromosomes. Nat. Rev. Genet. 5:123–35. doi: 10.1038/nrg1271
[5] ↑ Bowler, C., Vardi, A., and Allen, A. E. 2010. Oceanic and biogeochemical insights from diatom genomes. Annu. Rev. Mar. Sci. 2:333–65. doi: 10.1146/annurev-marine-120308-081051
[6] ↑ Dorrell, R. G., and Bowler, C. 2017. “Secondary plastids of stramenopiles,” in Secondary Endosymbioses. Advances in Botanical Research, Vol 84, ed Y. Hirakawa (London: Academic Press), 57–93.