Abstract
Did you know that your daily choices, including how much you exercise, what you eat, and even how you think, can change the basic units that are present in your cells? In this article, I will tell you about telomeres, which are the protective tips of DNA molecules—the molecules where the organism’s genes are stored. I will also introduce you to an enzyme that we discovered, called telomerase, which is responsible for the addition and maintenance of telomeres. After diving into the molecular details of telomeres and telomerase, I will reveal to you some very interesting connections between telomeres and human health. I hope, by the end of this article, you will be amazed by the way in which psychological, environmental, and societal factors can influence the fundamentals of our biology.
Professor Elizabeth Blackburn won the Nobel Prize in Physiology or Medicine in 2009, jointly with professor Carol Greider and professor Jack Szostak, for the discovery of how chromosomes are protected by telomeres and the enzyme telomerase.
Protective Tips on The Ends of DNA
Life, in all of its complexity, is a huge puzzle. Curious people like you and me are drawn to ask questions, like “What is this?,” “What is going on here?,” and “Why is this the case?”. I chose to engage this unending curiosity through the study of biology—the science of life. Because biology is so complex, you always have to ask yourself: what are the things that I can understand, and what should I focus on in my research. Otherwise, you get overwhelmed immediately by all the details of the biological phenomenon you are studying. One avenue you may take in the study of biology is called molecular biology, which examines biological processes through the landscape of molecules and their interactions. Molecular biology is a very satisfying scientific field for me because, in many ways, it lets you answer specific and basic questions about the mysteries of life. In this article, I will tell you about the discoveries we have made, using molecular biology, about an important protection mechanism at the ends of the DNA molecules—the molecules that contain the genetic instructions (the code) that pass from parents to children. But first, we need to lay out the groundwork.
DNA, Chromosomes, And Replication
Every living cell contains structures called chromosomes. Each chromosome contains a polymer (a very large molecule) called deoxyribonucleic acid, or DNA. Together, all this DNA is like a library of instructions that tell the cell itself, and any organism built from such cells, how to function. The DNA molecule comes in the form of a double helix which is composed of two linear strands that run opposite to each other and twist together forming the double helix (Figure 1). In chromosomes, DNA is tightly coiled and condensed inside the cells (Figure 1). In human cells, for example, there are 23 pairs of chromosomes that contain all of our DNA. These chromosomes are located in the cell’s nucleus and are called linear chromosomes, as each of them has two ends. This holds true for all cells of eukaryotes, which are cells that have a nucleus. Organisms with cells containing a nucleus include all animals, plants, fungi, as well as most algae. In contrast, organisms which do not have nucleus in their cells, called prokaryotes (such as bacteria), have circular chromosomes, which have no end (like a circle).
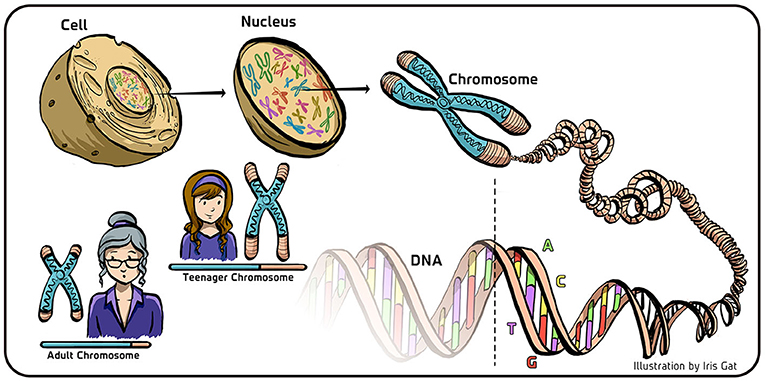
- Figure 1 - DNA and chromosomes in eukaryotic cells.
- In eukaryotes, the DNA is stored in the cell’s nucleus, where it is coiled and condensed in structures called chromosomes. The DNA appears in the form of a double helix, made of four bases (A, T, G, and C) schematically denoted here by the four colors composing the DNA. When cells replicate, the DNA is copied to create two new double helices from the original double helix. The molecular machinery that copies the DNA does not copy the sequence of the DNA at the end of the chromosome (transparent part of the DNA; bottom). As a result, the DNA becomes shorter with aging (lower left). To ensure a stable inheritance of the genetic material, a special mechanism is required to protect the end-part of the DNA sequence during replication.
When cells divide, their DNA must be replicated so that the new cells will also contain all the necessary instructions stored in the DNA. As you might know, the DNA polymer is a string of four basic chemical building blocks, called bases, that are symbolized by the letters A, T, G, and C (A stands for adenine, T for thymine, G for guanine, and C for cytosine). The sequence of these bases along the DNA polymer is the code that is used as the “instruction manual” for the cell. In the two strands composing the double helix, these bases always come in pairs, A-T and G-C, so that opposite to every A in one strand of the double helix there is a T in the other strand, and opposite to G there is a C and vice versa (Figure 1).
When DNA replication takes place in the nucleus, complex cellular machinery first separates the double helix into two single DNA strands. Then, each original strand serves as a template for the synthesis of its complementary strand. In this process, each base in each (by now separated) strand is matched by its respective pair base (T is complemented by A; A by T; G by C and C by G), until a whole new complementary strand is synthesized for each of the strands of the original DNA. At the end of this process, we obtain two new double helices that are identical to the original DNA.
So far, so good—we have a new double helix DNA ready to be used in a new eukaryotic cell following cell division. But it turns out that this complex machinery cannot copy the DNA strands up to their very ends (Figure 1). The sequence of base pairs at the ends of each DNA strand are not copied during cell division. This means that, following each replication, the original DNA will become shorter and shorter.
This is probably where your curious minds stop and ask—well, why does this happen? Why is the DNA not fully replicated during cell division? The truth is that we do not really know. This is one of those instances where we Biologists feel like archeologists—we try to decipher a historical turn of events using the fragmentary information of the artifacts that survived up until today. All that we do know is that the DNA is not fully copied during cell division (the “artifact”). We also surmise that the first cells in the evolution of life were prokaryotic (rather than eukaryotic) cells with circular chromosomes, where there are no free end to the DNA, and that eukaryotic cells, with their linear DNA—and thus DNA ends—developed later during evolution.
From such reasoning we think that the DNA replication machinery that operates in eukaryotes was originally developed in prokaryotes, where it worked well enough and did not have problems with the DNA ending, since there is no “ending” to a circular DNA? Does this then mean that a piece of the important coding genetic material contained in the DNA gets lost in case of the linear chromosomes of eukaryotic cells each time the cell replicates its genetic material—the DNA? Luckily, this is not the case, thanks to a protection mechanism that makes sure that none of the DNA important for its proper function in eukaryotes’ cells will become lost.
Telomeres—DNA Protectors
As we saw in the previous section (Figure 1), in eukaryotes, DNA replication stops before the end of the DNA strands. If you were asked how to protect the important material encoded at the end part of the DNA, can you think of a solution to that puzzle? It turns out that, at the ends of linear chromosomes, there are special DNA sequences called telomeres (in Greek, “telo” means end and “mere” means part). The DNA at the telomeres is called a “non-coding DNA” and it ensures that the coding part of the DNA (which includes the instructions that are transferred from parents to offspring) will be properly transferred to the next generation.
You can think of these telomeres like protective plastic tips at the ends of shoelaces. What actually happens is that the telomeres make up for the lack of DNA copying at the ends of chromosomes. Meaning that, to avoid the case whereby important (coding) parts at the end of the DNA will not be copied during cell division, now only parts of the telomeres (that are located at the very end of the chromosomes) are not being copied, but this does not result in any loss of meaningful (coding) parts of the DNA material. This is similar to the case where the plastic tips of shoelaces get partially worn out when you use them many times, but the shoelaces themselves remain protected. Even more excitingly, we discovered that, when a telomere shortens, it attracts a special enzyme that can add more telomeric DNA to the chromosome. Thus, the telomeres can be replenished. This is the beautiful solution that nature found to the problem of incomplete DNA replication at the ends of linear chromosomes.
In the 1930, telomeres were identified by Muller [1] and McClintock [2] as “something” special at the ends of chromosomes that protected their ends. Later on, with the development of Molecular Biology, it was possible to characterize the molecular nature of the telomeres. In 1978, Joseph Gall and I identified the structure of telomeres at the end of the linear chromosomes in an interesting organism called Tetrahymena thermophila, commonly found in pond scum [3]. We found that telomeres of this pond scum organism are made of a specific pattern of DNA bases, namely the sequence TTGGGG (Figure 2), which was repeated a different number of times (about 20–50) in different chromosomes within the population of cells. Soon after, similar repeating patterns were discovered in the telomeres of other organisms, such as ciliate Oxytricha (TTTTGGGG) and slime molds (TTAGGG) [4]. Human telomeres consist of a TTAGGG sequence, which repeats thousands of times at the ends of all of our chromosomes [4].
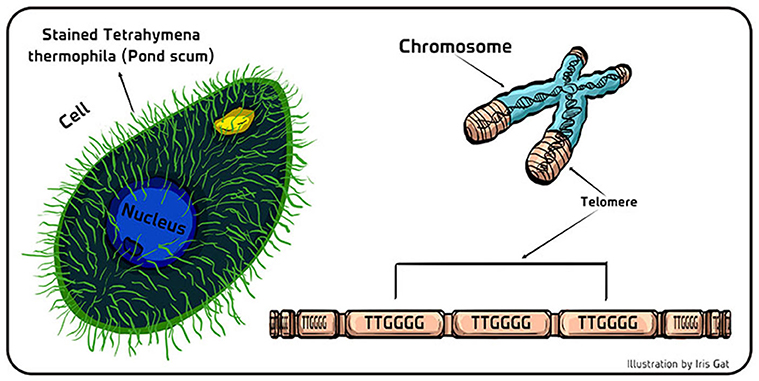
- Figure 2 - Telomeres in pond scum.
- (Left) Schematic of the single-celled eukaryotic organism Tetrahymena thermophila that lives in fresh water. The hundreds of hair-like projections (cilia) on the cell’s surface act as tiny oars so it can swim around to hunt for food, or for a mate. (Right) Telomeres are like the protective tips of shoelaces that protect the genetic material stored in the coding part of the DNA (blue part of the chromosome). The telomeric (non-coding part) of the DNA (brown part of the chromosome) is comprised of repeating units of bases (TTGGGG in Tetrahymena; TTAGGG in us).
There are many interesting questions that could be posed at this point about telomeres and their repeating patterns. What I would like to focus on now is on the following question: how are telomeres added to the ends of chromosomes? And does this machinery supply protection in other situations, in addition to cell’s multiplication? In the next sections, we will have a look at the wonderous mechanism that builds telomeres, and see what telomeres can teach us about human health and wellbeing.
Extending the Life of Tetrahymena—The Discovery of Telomerase
When I was studying Tetrahymena and its related species in the early 1980s, it was known that during their life-cycles, there is a stage where the original chromosomes are cut into smaller linear chromosomes, called minichromosomes. As we saw in the previous section for the whole chromosome, these minichromosomes also end with telomeric repeats of TTGGGG. When we found the structure of these DNA repeats in these pond scum cells, we tried to see if we could fit it into the known body of information at that time regarding how DNA bases are added to chromosomes. The only known mechanisms for DNA base addition at that time were (i) DNA replication and (ii) DNA recombination. Each of these mechanisms had very specific sets of rules for DNA addition, which were violated by the telomeric repeats that we had found (e.g., by the fact that telomeres of the pond scum were heterogeneous in length, sometimes few and sometimes many repeats, and sometimes all the telomeres grew longer at once). These surprising behaviors meant that we could not fit our new findings about the structure of the telomeres into what, back then, were the only well-established principles and knowledge about DNA.
In these kinds of special crossroads in science, you have to think outside the box and entertain other, creative, possibilities in order to find the answer you are looking for. In this case, I tried to think which mechanism could be responsible for the addition of the telomeric repeats to the minichromosomes at the stage after they are cut from the long chromosomes. One possibility was the activity of an enzyme. To check this hypothesis, I put an extract of pond scum cells into a test tube and added various chemical substances to see whether any of the substances added telomeres to the minichromosomes. After some trial and error, I found that this extract did promote synthesis of telomeric repeats.
At this point, Carol Greider joined my lab as a Ph.D. student. Her challenge was to simplify the reaction in the test tube, so that we could find the specific enzyme activity responsible for the addition of the telomeric ends. After some more trials and errors, Carol simplified the assay to its bare essentials. We added synthetic repeats of TTGGGG, right after the chromosomes were chopped up into minichromosomes. At this stage, the telomeres were expected to be added. We added DNA building blocks into the test tube (two molecules called dGTP and TTP), along with some magnesium salt. We saw that TTGGGG repeats were indeed added to the ends of our synthetic DNA [5], which meant that we found a possible mechanism for the elongation of telomeres! Carol’s fellow graduate student Claire Wyman came up with a name for the new enzyme that seemed to be responsible for the addition of telomeric repeats: telomerase [6].
To validate our hypothesis regarding the function of telomerase in the elongation of the DNA in living cells (not just in the test tube), we performed some additional experiments. I will not go into the details here, but we found that telomerase had an RNA part, containing a short sequence that is the complement of the DNA sequence of the telomeres. This RNA acts as a template for the addition of the complementary telomeric sequence. In some of these experiments, we changed (or “mutated”) the structure of telomerase to see whether mutations affected its capability to synthesize telomeric DNA and elongate telomeres. In mutations that allowed the telomerase to keep working but which had a mutation in the RNA template for telomeric DNA addition, we found that pond scum cells had alternative telomeric repeats—different than the original TTGGGG repeats and complementary to the base change we had put in the template [7].
Importantly, when we mutated telomerase so it did not function anymore, the telomeres of pond scum cells got shorter in each cell division, and finally after 20–25 divisions the cells stopped dividing and died [7] (Figure 3, left). This meant that the normally immortal pond scum cells (i.e., cells that multiply seemingly forever) became mortal (i.e., stop multiplying after a certain numbers of multiplications) when the activity of telomerase was damaged. All of these findings have led to the conclusion that telomerase is indeed responsible for the addition of telomeric DNA to the ends of linear chromosomes. It was that discovery for which Carol and I were awarded the Nobel Prize in Physiology or Medicine in 2009 (together with another collaborator, Jack Szostak, with whom I had explored telomere function in baker’s yeast cells). It is important to note that later on, after the discovery of telomerase, it was found that its activity is important not only in the case of cell multiplication. It turned out that telomeric DNA is chemically quite susceptible to damage inside cells, and so there are different situations where it should be fixed—especially over long periods, such as the time frames of human lives. Therefore, telomerase is important even in cells which are not multiplying (Figure 3, right).
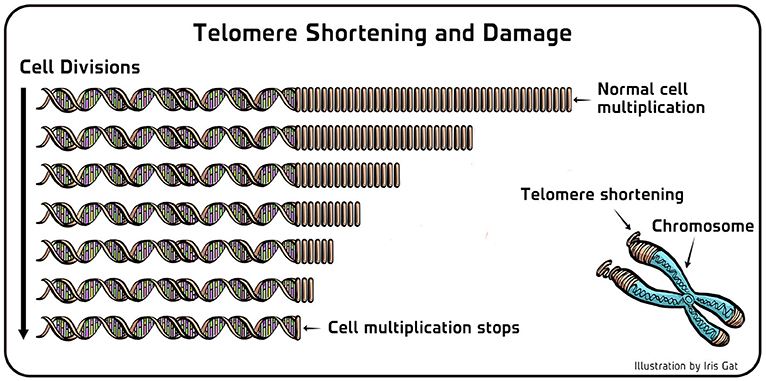
- Figure 3 - Telomere shortening and damage.
- (Left) In cells of the pond scum where telomerase does not work properly, the telomeric DNA (right repeating part of the DNA in this figure) gets shorter with every cell multiplication. When the telomeres become too short, the cell stops multiplying. The same holds true for human cells. (Right) Telomeric ends are chemically susceptible to damage inside cells. Therefore, the activity of telomerase is important also for the maintenance of telomeres in cells which are not dividing.
Now that you are acquainted with my work on telomeres and telomerase, I would like to present to you another line of research that developed later on along my scientific path. This line of research connected telomeres to human health in some very surprising ways.
Lessons From Telomeres—How to Improve Human Health
When I was working on telomere maintenance and telomerase in human cells at the University of California, San Francisco (UCSF), I was approached by a bright psychology researcher, Elissa Epel. Elissa, now a professor in the Department of Psychiatry at UCSF, was then doing her post-doctoral studies on severe chronic stress. At that time, in the beginning of 2000s, it was known that people who are under severe chronic stress often exhibit physiological changes that mimic what we see when people get older naturally, only at a faster pace. In other words, chronic stress was related to accelerated human aging. From another viewpoint, by studying telomeres we knew that if you make a genetic mutation in the telomerase of pond scum or yeast cells that would make it stop working, the telomeres would become shorter and shorter in every multiplication until eventually they would get too short and that would send signals to the cell to stop multiplying (Figure 3). This brought up a new idea that was starting to get some traction in the field: that maybe telomere shortening is related to aging of mammals like us. It was not clear then what was happening in human cells during aging.
When Elissa came to me and asked whether chronic stress could possibly be related to telomere shortening, I thought that this was a very interesting question. She told me about a fascinating study that she was doing on mothers with children that had either developmental disorders or chronic illnesses. This group of mothers was under severe chronic stress, partly because there is often a lack of support for this kind of situation in the United States. In addition to the scientific interest that was sparked in me after hearing about Elissa’s research and wondering about the possible relation of her findings to telomere shortening, I also had another, more personal reason for engaging in this research. At that time, my son was growing up and I found myself fairly often worried about him. That made me feel very empathic toward the women in Elissa’s study, realizing how stressed out they could feel in their challenging caregiving situations.
So, we decided to launch a pilot study in which my group measured telomerase activity and another group measured telomere length in cells of mothers who were the main caregivers of children with chronic illnesses. We compared the findings to control parents, matched in all respects except that they did not have a child with a chronic illness. Our work was led by a post-doctoral researcher named Jue Lin, who was previously working on yeast telomeres and telomerase and was intrigued by this new line of research. Right away, in this first study, we received a surprising result—there was a significant, quantitative correlation between telomere length—in this case, telomere shortness—and both the chronicity of the caregiving and perceived psychological stress! (Figure 4). This was an amazing result, and it was the first indication that a psychological situation like chronic stress could be correlated with this very clear, physical change at the most basic molecular level!
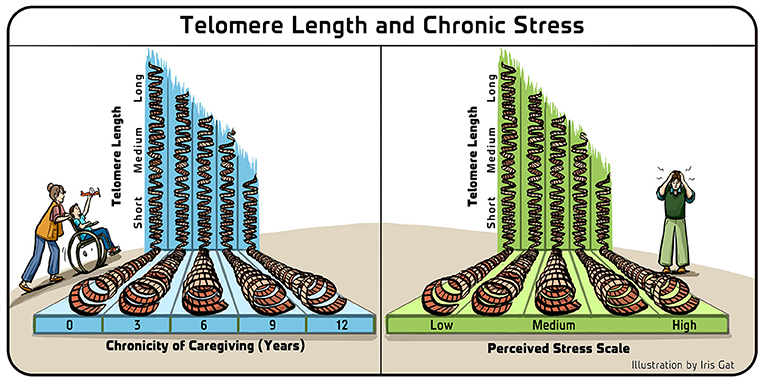
- Figure 4 - Telomere length and chronic stress.
- In the study that we first performed with mothers who were the main caregivers of children with chronic illnesses, we found that there was a statistically significant correlation between the shortness of the mothers’ telomeres and both the number of years that they had been in that situation (Left), and their perceived level of stress (Right). Adapted from Epel et al. [8].
As scientists, we do not rush to conclusions because we are aware of the human tendency of imposing what we want to see on reality and on our data. This tendency means we should be especially suspicious when the initial results we get match our “desired” hypothesis. Therefore, we decided to take additional actions to verify our initial results. One route that we took was to use different groups of people with similar chronic psychological stress of caregiving (such as caring for a family member with dementia) and see if the results on their telomere length would replicate. Additional research has involved checking for possible relations between telomere length and other parameters, such as environmental factors, educational parameters, and additional mental or psychological factors. Many such connections were found, and my group and others eventually concluded that that there are very significant relationships between telomere length and human health [9].
In 2017, Elissa and I co-authored a book for general readers that presents the heart and soul of our continuous work on telomeres and human health, called: The Telomere Effect: A Revolutionary Approach to Living Younger, Healthier, Longer [10] (see Additional Materials). Although I should mention that it was our publishers who insisted that we add “revolutionary” to this book’s title, our book does in fact collect and present many interesting facts and conclusions. I would like to share two significant points. First, lifestyle and attitude matter! Meaning, the ways we sleep, eat, and exercise on a regular basis affect the lengths of our telomeres and, because of that, these activities can either promote or harm our long-term health and longevity. The same is true for habits of negative thinking, which are correlated with telomere shortening; as opposed to positive and resilient thinking, which enhance good telomere maintenance. Second, I want to emphasize the broader, social aspect of telomere length. Telomeres are affected by our environment! That means that when we make sure to support one another, on an individual and societal levels, we promote good telomere maintenance and overall human health. Let us remember, then, the important role we have in making sure that our telomeres, and the telomeres of our fellow humans, will stay long and healthy. The choices that we make on a daily basis, as well as societal influences that we must all keep striving to improve, do matter, because they are reflected even in the most fundamental molecular biology of our cells.
Recommendation for Young Minds
There is a lot of advice that I can give you when it comes to doing science and being a scientist (Figure 5). I would like to start by emphasizing the power of persistence. If you find yourself interested in science, then you should know that you will have to persist because sometimes it will look quite daunting and complex. But, do not give up and you will find at some point that there is a wonderful time where you break through the challenge. Then you will feel very satisfied and know that your persistence was worthwhile. Also, for your long-term satisfaction, you have to be convinced that whatever you are doing is something of value. So, when you choose your way in science (or in any other profession), make sure to be engaged in things you believe are worthwhile doing. This way, there will be an inherent value in your work, and that will encourage you to persist even at times where it would be easier to give up. When you chose your particular path and persist in it, remember that everything, and especially knowledge and technology, can be used in different ways. Make sure to always keep in mind to use whatever you are engaged in for the good of humanity. And while you do so, also remember to always check your results rigorously and not let your personal preferences and wishes interfere with the way you collect and analyze data.
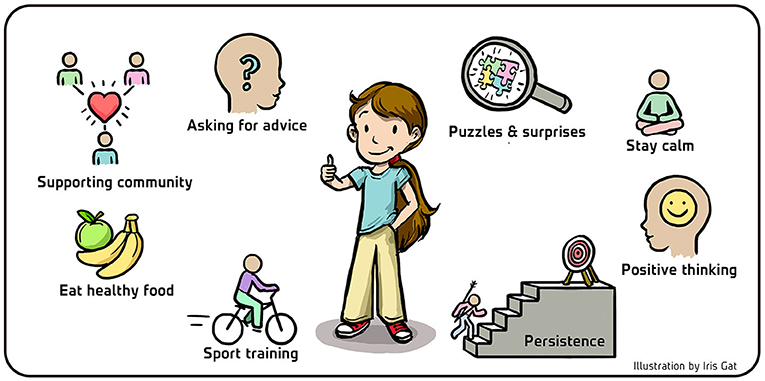
- Figure 5 - Recommendations for young minds.
Another aspect that I want to stress is the importance of asking for advice. I learned that the hard way: early in my career, I was hesitant to ask for advice. I worried that people might think less of me if I seemed to be needing help, so I would refrain from asking for it. In hindsight, I see that this sometimes made my path more difficult than it had to be. I say that, because as time progressed and specifically when my son was born and I had to find my way around being a young mother while being a full professor at the university, I opened up to the possibility of asking for advice. It turned out to be tremendously helpful—I was given all sorts of solutions I would never had thought about from people who already hacked the challenge I was facing, both personally and professionally. I realized that, in most cases, people like helping each other and actually respect you for your willingness to ask for advice. So I encourage you to ask for advice along your way instead of making your life unnecessarily hard. Also, please remember that there are many people who want you to succeed, so find these ones and keep them close, while keeping in perspective other people who are not helpful.
I also want to speak with you about puzzles and surprises. For me, science is full of puzzles—some are big puzzles, that you invest your whole career trying to solve, and some are smaller puzzles that you are faced with every day. My big puzzle is trying to understand how life itself works. That big puzzle breaks down into smaller puzzles each day when I analyze data and try to solve a particular question about a particular phenomenon. In the broad picture, science supplies for me both safety and stability, through an impersonal, rigorous way to get to a truth, and also surprises and thrills, through the unexpected discoveries that you make along the way. I call these surprises birthday present surprises, and I enjoy them very much. My advice to you, in that regard, is to fully enjoy the birthday present surprises that will cross your path. I know that some of you may be somewhat intimidated by surprises, but I can assure you that the kind of surprises you will meet in science are good surprises, if you approach them that way. The better the grasp you will have at your area of expertise, the freer you will feel to step out of your known territory and explore scientific birthday surprises.
Finally, I would like to direct some special words of encouragement to the future women scientists among you. As you can see from my example, women can have a successful, fulfilling, often joyful career in science. I do not know if you know, but in 2009 when Carol Greider and I were awarded the Nobel Prize in Physiology or Medicine, three other women won Nobel Prizes: in Chemistry, Economics, and Literature. I felt that this was sending an important signal to younger scientists, saying that a Nobel Laureate could just as well be a woman as it can be a man. Therefore, it is an honor for me to be a symbol for the future generation of women, and indeed all, scientists.
Additional Materials
1. The Telomere Effect: A Revolutionary Approach to Living Younger, Healthier, Longer—Amazon.
2. Women Who Changed Science—Elizabeth Blackburn.
3. Elizabeth Blackburn on the telomere effect—The Guardian.
Glossary
Chromosome: ↑ A structure of tightly packaged DNA inside cells.
DNA: ↑ The molecule that carries information about the appearance and function of living organisms.
Eukaryotes: ↑ Organisms whose cells contain a nucleus.
Prokaryotes: ↑ Organisms whose cells do not contain a nucleus.
Telomere: ↑ Protective DNA sequence (or a “cap”) at the ends of linear chromosomes.
Enzymes: ↑ Protein structures that enhance chemical activity in living cells.
Telomerase: ↑ An enzyme that adds telomeric DNA to chromosomes.
RNA: ↑ A moveable copy of DNA which the cell uses to perform different actions, such as copying DNA.
Conflict of Interest
The author declares that the research was conducted in the absence of any commercial or financial relationships that could be construed as a potential conflict of interest.
Acknowledgments
I wish to thank Noa Segev for conducting the interview which served as the basis for this paper and for co-authoring the paper, to Iris Gat for providing the illustrations, and to Susan Debad for copyediting the manuscript.
References
[1] ↑ Muller, H. J. 1938. The remaking of chromosomes. Collect. Net 13:181–98.
[2] ↑ McClintock, B. 1939. The behavior in successive nuclear divisions of a chromosome broken at meiosis. Proc. Natl. Acad. Sci. U.S.A. 25:405–16. doi: 10.1073/pnas.25.8.405
[3] ↑ Blackburn, E. H., and Gall, J. G. 1978. A tandemly repeated sequence at the termini of the extrachromosomal ribosomal RNA genes in Tetrahymena. J. Mol. Biol. 120:33–53.
[4] ↑ Blackburn, E. H. 2010. Telomeres and telomerase: the means to the end (Nobel lecture). Angew. Chem. Int. Ed. 49:7405–21. doi: 10.1002/anie.201002387
[5] ↑ Greider, C. W., and Blackburn, E. H. 1985. Identification of a specific telomere terminal transferase activity in Tetrahymena extracts. Cell. 43:405–13.
[6] ↑ Greider, C. W., and Blackburn, E. H. 1987. The telomere terminal transferase of Tetrahymena is a ribonucleoprotein enzyme with two kinds of primer specificity. Cell. 51:887–98.
[7] ↑ Yu, G. L., Bradley, J. D., Attardi, L. D., and Blackburn, E. H. 1990. In vivo. alteration of telomere sequences and senescence caused by mutated Tetrahymena telomerase RNAs. Nature. 344:126–32.
[8] ↑ Epel, E. S., Blackburn, E. H., Lin, J., Dhabhar, F. S., Adler, N. E., Morrow, J. D., et al. 2004. Accelerated telomere shortening in response to life stress. Proc. Natl. Acad. Sci. U.S.A. 101:17312–5. doi: 10.1073/pnas.0407162101
[9] ↑ Blackburn, E. H., Epel, E. S., and Lin, J. 2015. Human telomere biology: a contributory and interactive factor in aging, disease risks, and protection. Science. 350:1193–8. doi: 10.1126/science.aab3389
[10] ↑ Blackburn, E., and Epel, E. 2017. The Telomere Effect: A Revolutionary Approach to Living Younger, Healthier, Longer. New York, NY: Grand Central Publishing.