Abstract
Throughout human history, we have witnessed several major breakthroughs in science and technology that have transformed our society, such as the Industrial Revolution and the Internet Revolution. The next revolution is already underway, made possible by a technology I co-discovered that allows scientists to change the genes of many organisms and plants. This powerful technology, called CRISPR, could improve human health, help crops grow better, and fight climate change. CRISPR could even influence human evolution. In this article, I will explain how CRISPR works, my discovery of a specific CRISPR system called CRISPR-Cas9, and the current and future uses of this technology. Finally, I will discuss some of the ethical concerns around using CRISPR tools and the actions scientists and societies should take to ensure responsible and impactful application of CRISPR technology.
Professor Jennifer Doudna won the Nobel Prize in Chemistry in 2020, jointly with Emmanuelle Charpentier, for the development of a method for genome editing.
Introduction
Living cells contain molecules called DNA, which serve as a detailed “instruction manual” that tells each cell how to operate. The entire instruction manual is called the organism’s genome. Small sets of instructions in the manual, called genes, code for individual proteins that determine the specific functions of each cell (like the ability of muscle cells to contract) and the traits of the organism (like skin and eye color). There is a “middleman” between genes and proteins, however—a molecule called RNA that carries the information from DNA to the cellular machinery that makes proteins. Unlike DNA, RNA is found throughout the cell, not just in the nucleus.
Tiny organisms called viruses can interfere with this process and cause disease—like the SARS-CoV-2 virus responsible for the COVID-19 pandemic. Viruses infect cells by inserting their genetic material into the cell and then directing the cell to create copies of the virus, which can then spread throughout the organism. Viruses do not infect only people and animals—they can also infect bacteria. However, certain bacteria have a type of immune system that helps them protect themselves against viruses. When a virus infects a bacterial cell, the bacterium keeps a copy of the viral DNA in a special site in its genome called CRISPR [1]. This part of the bacterial genome contains between a few to hundreds of pieces of DNA from different viruses, separated by repeating pieces of bacterial DNA (Figure 1). These CRISPR pieces act as a memory system, allowing the bacterium to quickly detect that specific viral DNA if it is ever inserted into the bacterium again. Using special proteins, called Cas proteins, the bacterium manages to not only detect, but also get rid of the foreign viral DNA. The combined system of RNA and Cas proteins is called a CRISPR-Cas system [2].
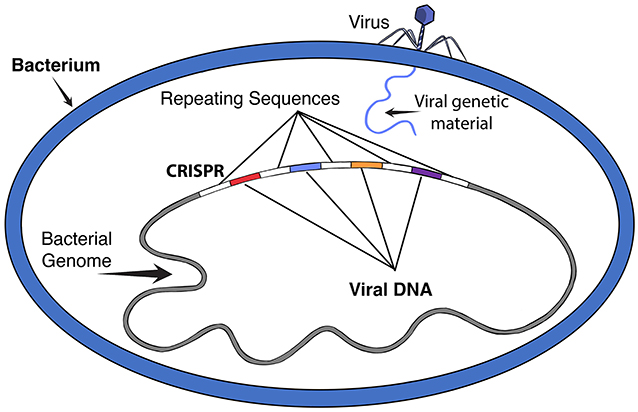
- Figure 1 - CRISPR in a bacterial cell.
- When viruses infect a bacterial cell, the bacterium keeps a copy of the viral DNA in a site in its genome called CRISPR. The viral DNA pieces are separated by repeating pieces of equally spaced bacterial DNA. This CRISPR segment in the bacterial genome acts as a memory system, allowing the bacterium to quickly recognize and respond to future infections by similar viruses.
CRISPR-Cas9: A Powerful Technology for Gene Editing
I first heard about CRISPR around 2006. At that time, it was starting to be understood that CRISPR provides bacteria with immunity against viruses [3], but scientists did not know how. When I started working with Emmanuelle Charpentier, we hypothesized that this mechanism involved a protein that would chop up, or “cleave”, the viral DNA. We knew about a protein called Cas9 that was known to cooperate with the CRISPR system, but its specific function was still unknown [4]. A collaboration began between our labs, led by two talented students—Martin Jinek and Krzysztof Chylinski—to study this mysterious Cas9 protein.
We knew that, somehow, the Cas9 protein had to find the specific viral DNA. Our theory was that if the Cas9 protein were attached to a piece of RNA that matched the viral DNA, they might pair up and this system would bring Cas9 to the correct place in the genome. So, we combined the Cas9 protein with RNA that matched part of the CRISPR sequence (called crRNA)—to see if it would cut up a target DNA sequence that we wanted to alter, but nothing happened. We did not give up. Instead, we considered the possibility that a piece might be missing from the puzzle. The answer came in the form of an additional piece of RNA that Emmanuelle’s lab was working on, called a tracrRNA [5]. We combined the tracrRNA with the crRNA and the Cas9 protein and repeated the experiment. This time the complex successfully targeted and cleaved a specific piece of DNA (Figure 2). We had discovered that the natural CRISPR-Cas9 system is a dual RNA-guided DNA cutting system—where two different pieces of RNA are required to cut the viral DNA.
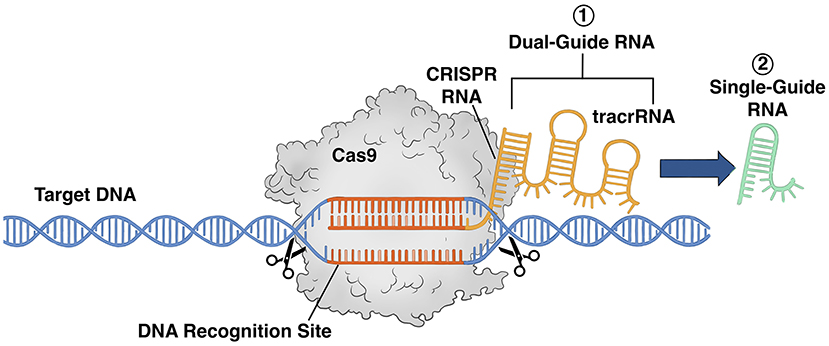
- Figure 2 - CRISPR-Cas9 system.
- (1) Our original CRISPR-Cas9 cleaving system contained a protein called Cas9 carrying two pieces of RNA—crRNA and tracrRNA. This dual-guide RNA system could recognize and cleave a specific part of a target DNA. (2) We then engineered and simplified the two RNA pieces of the dual-guide RNA into a single piece of RNA called single-guide RNA. This transformed the CRISPR-Cas9 system into one that could recognize and cleave any piece of any DNA scientists are interested in.
Next, we set our sights on simplifying this process. This entailed determining which parts of both the crRNA and tracrRNA were essential and developing a more streamlined system. We fused the essential parts into one engineered piece of RNA, transforming the system into a single RNA-guided DNA cutting system (Figure 2) [2, 6]. This was extremely important because it is much easier to produce one desired piece of RNA in the lab than to reliably combine two different pieces. To learn more about the discovery of the CRISPR-Cas9 system, view this video.
Additional studies from several research groups helped figure out the structure of the Cas9 protein and further details of the cleavage process. It was found that when the CRISPR-Cas9 complex arrives at the correct location on the DNA molecule, which is made of two strands wound together, it first separates the strands. Then the RNA portion of CRISPR sticks to the matching sequence in the DNA. The complex then uses small “molecular scissors” to cut both strands of the DNA (to learn more about the structure of Cas9 and the cleavage process, see here). Once the DNA is cleaved, DNA-repair mechanisms are activated in the cell. Scientists can take advantage of CRISPR’s ability to target a specific site in the DNA for cleavage and repair to essentially delete, change, or replace any part of the DNA. This is called gene editing or genome editing.
After our initial discovery and simplification of the CRISPR-Cas9 system for gene editing, many more systems with different types of Cas protein have been developed and the field of CRISPR-Cas technology has expanded. Gene editing using CRISPR-Cas systems can be performed in all living cells, including humans, animals, microbes, and plants. This makes it a “cross-cutting” technology that influences not only basic research, but also has great potential implications in the fields of medicine, agriculture, and even climate change.
CRISPR and Human Health
CRISPR-based gene editing could benefit human health in several ways (Figure 3), such as to treat diseases. There are more than 7,000 human genetic diseases, which are caused by errors in particular genes and can be passed from parents to children. Genetic diseases include sickle cell anemia, in which the shape of red blood cells is impaired so they cannot carry oxygen properly; cystic fibrosis, in which unusual production of mucous damages the lungs; and Huntington’s disease, in which buildup of an abnormal protein damages brain cells. If we edit the mutated genes in people with these diseases, we might be able to correct the errors and prevent the diseases. There has already been remarkable success in addressing genetic disorders using CRISPR technology—from treating a patient with sickle cell anemia (learn more here) or an individual with a vision disease called Leber congenital amaurosis, or LCA (read more here). It is also possible to use CRISPR to treat diseases that are not inherited, such as AIDS, in which a person’s immune system is damaged. AIDS is caused by a virus called HIV, which CRISPR can target to remove the HIV DNA from the patient’s cells [7]. Cancer is another important example. CRISPR can be used to improve the ability of the body’s immune cells to target and eliminate cancer cells [8].
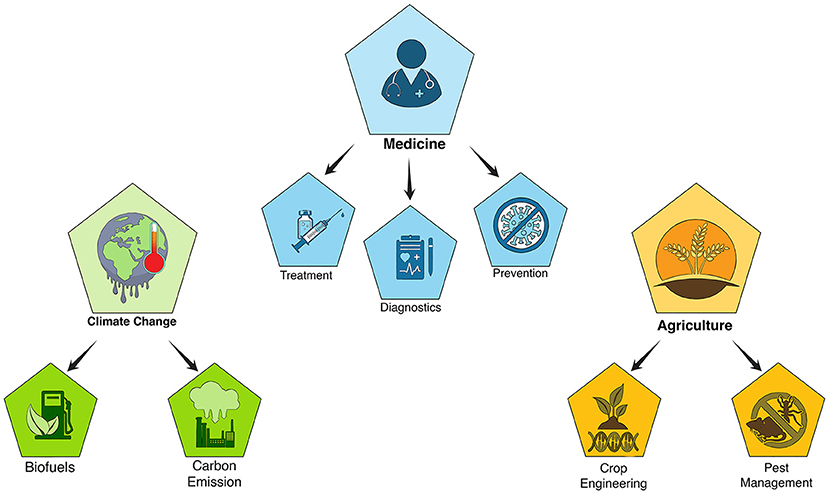
- Figure 3 - Examples of CRISPR applications.
- CRISPR-based gene editing technologies have applications in many different fields, including medicine, agriculture, and climate change.
Another application of CRISPR is to prevent diseases before they emerge—a field of medical practice called preventive medicine. Preventive medicine can curb a great deal of human suffering and conserve valuable resources, such as funding and materials, that would otherwise be used to treat diseases. CRISPR could be used to change certain disease-related genes for the better by introducing “good” mutations that prevent the development of a disease in the future. CRISPR-based preventive medicine could be applied, for example, to prevent heart diseases [9], Alzheimer’s disease [10], and the spread of infectious diseases. CRISPR can be used to diagnose and treat patients quickly by detecting the DNA of viruses (like SARS-CoV-2) in patients or in animals [11, 12]. Early identification can help doctors take the necessary measures to prevent the disease from spreading, such as early quarantine guidelines. In addition, using CRISPR to eliminate disease-causing organisms from people who are infected could also help stop the spread of infection [13].
CRISPR gene editing might also be used to fight diseases that are spread from animals to humans. For example, some diseases, like malaria, are passed to humans by mosquitoes. Using CRISPR, scientists can edit the genes of egg and sperm cells in mosquitoes so that their offspring will be unable to infect humans with malaria [14]. This means that it is possible to modify whole populations of mosquitoes to become harmless to humans. This approach is called germline genome editing and goes beyond editing the genes of just one individual as we described above—it means that we can use CRISPR to edit genes across many generations of individuals.
Other Uses of CRISPR
CRISPR-based gene editing technologies can also be used to modify the genes of plants, making crops more nutritious [15] or altering foods like peanuts to reduce allergens. It is possible to selectively enhance specific traits of crops to improve the amount of food harvested or to make crop plants more resistant to diseases, insect pests, drought, or extreme temperatures. To manage crop pests directly, we could use CRISPR to edit insects’ genes to limit their ability to damage plants [16]. One strategy aims to spread infertility through a pest population, so that it would be unable to reproduce.
Beyond agriculture, CRISPR technologies can be applied to addressing climate change. One potential direction is engineering organisms such as algae to produce biofuels that could be used to power our homes and cars, or to remove carbon dioxide from the air through absorption. It might also be possible to modify the genomes of microbes to direct their interaction with plants such that they would store carbon dioxide instead of releasing it to the air.
More Work is Needed
Significant progress continues to be made in applying CRISPR to many areas, but there is still work to be done to address its limitations. For example, one major issue with current CRISPR technologies involves their delivery, particularly when they are given to humans [17]. It is difficult to make sure that CRISPR-Cas complexes get to the correct cells, and not to other cells that were not the target of treatment. To improve delivery, researchers are investigating other known CRISPR systems besides the CRISPR-Cas9 system described in this article. For example, some viruses also have CRISPR-Cas systems [18] containing smaller Cas proteins that might be easier to target to specific cells. We are also discovering new Cas proteins and engineering known Cas proteins to improve their function, such as making them more efficient at locating matching DNA sequences and more accurate at cutting them. We could also create Cas proteins that can detect and chop up RNA instead of DNA [19], which would be useful for dealing with viruses that have RNA as their genetic material.
Additionally, we could optimize the crRNA guide molecules that are carried by Cas proteins—approaching these challenges from a different angle by identifying the best crRNA sequences for each use. These are all very exciting areas for future improvement and application that could potentially revolutionize important aspects of our lives, such as healthcare and food production.
Ethical Concerns
CRISPR technology has proven extremely powerful and efficient, and it could change the way we relate to nature and to each other. However, we must always be careful and responsible when dealing with such impactful technologies. Imagine, for example, if we had the power to engineer “improved humans” with specific traits and capabilities or to efficiently engineer many of the animals and plants around us. We might dramatically change the course of evolution. To read more about the ethical responsibility around CRISPR, see the last section in this paper.
In 2015, I first called for a worldwide ban on all CRISPR activity related to human germline genome editing, after a Chinese scientist edited the genomes of twin embryos. My call for a ban was a personal way to vote against irresponsible use of CRISPR technology. Since then, many other important efforts have been made to ensure responsible use of CRISPR [20].
As a society, we must ensure that our application of CRISPR technology will be safe and beneficial for everyone. Scientists should help the public understand and accept the use of CRISPR, as well as the need for ethical guidelines, including detailed descriptions of when we consider CRISPR technologies to be safe enough to use in humans. Additionally, we should establish policies and laws that ensure these guidelines are followed [21]. It is imperative that we are careful not to use this powerful technology before the necessary ethical guidelines are in place. If we continue to act thoughtfully, CRISPR will help usher in a new era of positive, long-term benefits for our species.
Recommendation for Young Minds
All my life, science has been about discovery and figuring out how to answer questions for which we do not yet have answers. I always approach scientific questions with a great sense of wonder, as even a seemingly trivial experiment in the lab could produce a new piece of information—one that I am the first person to learn. This act is like peeling an onion, discovering layers upon layers of truth about how our world works. I find that very exciting and satisfying.
I came from a very humble background—no one in my family was a scientist. During my childhood and throughout my education, I kept following my curiosity and pursuing what I found interesting, even through challenging times. That is what I advise to the next generation of scientists. You may not know where your curiosity will lead you, but be persistent and follow it anyway! I certainly did not know that my early fascination with CRISPR would become a world-changing technology with implications across all of science.
Persistence is key in fear management as well. At one time or another, we all have doubts about whether the things we pursue will work out, or whether we might embarrass ourselves in the process. Those fears are perfectly normal. We cannot dismiss them, but we can certainly manage them by finding sources of support and simply going for it. When you find yourself having to make hard decisions or are worried about taking the next step, remember to embrace both the possibility of success and of failure—your passion will lead you in the right direction. Anyone can find the courage to take that crucial step, even if it is scary.
Perhaps you are similarly passionate about answering questions related to the natural world. If that sounds interesting to you, I encourage you to pursue a career in science. Nowadays, young scientists have very powerful tools at their disposal, like CRISPR. That makes our work as scientists very exciting yet also quite challenging (Figure 4).
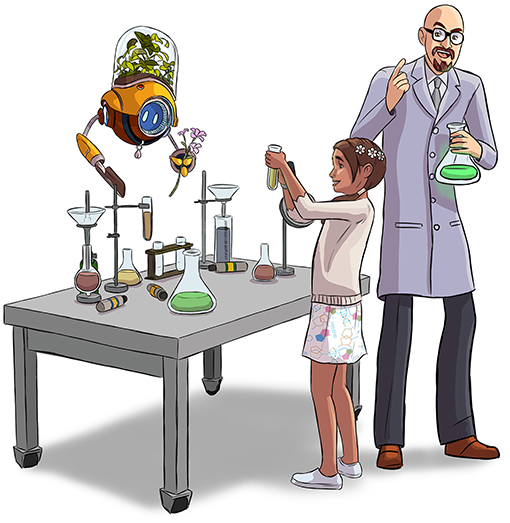
- Figure 4 - The future of science.
- Today and in years to come, scientific technologies such as CRISPR will continue to improve and become more accessible to scientists around the world. The possibilities that lie ahead make a young scientist’s path very exciting, but also one that carries great responsibility. Researchers must commit to ensuring that their work creates a positive impact on humans and on the greater natural world we are a part of.
Our responsibility as a field is to make sure we use these tools wisely, so that our work will have a positive impact on the human condition and on the environment in which we live.
Additional Materials
- To learn more about CRISPR, and its ethical implications, you can visit Prof. Doudna’s Innovative Genomics Institute webpage, with multiple resources and videos: https://innovativegenomics.org/what-is-crispr/.
- To learn more about how CRISPR could be used for gene editing, see this video.
Glossary
DNA: ↑ The instruction manual of living cells.
Genome: ↑ All the genetic information of an organism.
Gene: ↑ A section of DNA that determines a specific function or trait in an organism.
RNA: ↑ A moveable copy of DNA that serves as the “middleman” between DNA and proteins.
Immune System: ↑ The system that helps organisms stay healthy and fight diseases.
CRISPR: ↑ A system in the bacterial genome that helps protect bacteria against viral infections. It stands for Clustered Regularly Interspaced Short Palindromic Repeats.
CRISPR-Cas System: ↑ A system within bacteria that uses CRISPR RNA and Cas (CRISPR-associated) proteins to detect and remove viral DNA.
Germline Genome Editing: ↑ Changing genes in egg and sperm cells of parents so that the changed genes will pass on to their offspring.
Biofuels: ↑ Fuels that are made from plants, algae, or animal waste.
Conflict of Interest
The author declares that the research was conducted in the absence of any commercial or financial relationships that could be construed as a potential conflict of interest.
Acknowledgments
I wish to thank Or Raphael for conducting the interview that served as the basis for this paper and for co-authoring the paper, and Aviad Sajevitch for providing the figures.
References
[1] ↑ Sorek, R., Kunin, V., and Hugenholtz, P. 2008. CRISPR—a widespread system that provides acquired resistance against phages in bacteria and archaea. Nat. Rev. Microbiol. 6:181–186. doi: 10.1038/nrmicro1793
[2] ↑ Makarova, K. S., Haft, D. H., Barrangou, R., Brouns, S. J., Charpentier, E., Horvath, P., et al. 2011. Evolution and classification of the CRISPR–Cas systems. Nat. Rev. Microbiol. 9:467–477. doi: 10.1038/nrmicro2577
[3] ↑ Barrangou, R., Fremaux, C., Deveau, H., Richards, M., Boyaval, P., Moineau, S., et al. 2007. CRISPR provides acquired resistance against viruses in prokaryotes. Science 315:1709–1712. doi: 10.1126/science.1138140
[4] ↑ Haft, D. H., Selengut, J., Mongodin, E. F., and Nelson, K. E. 2005. A guild of 45 CRISPR-associated Cas protein families and multiple CRISPR/Cas subtypes exist in prokaryotic genomes. PLoS Comput. Biol. 1:e60. doi: 10.1371/journal.pcbi.0010060
[5] ↑ Deltcheva, E., Chylinski, K., Sharma, C. M., Gonzales, K., Chao, Y., Pirzada, Z. A., et al. 2011. CRISPR RNA maturation by trans-encoded small RNA and host factor RNase III. Nature 471:602–607. doi: 10.1038/nature09886
[6] ↑ Jinek, M., Chylinski, K., Fonfara, I., Hauer, M., Doudna, J. A., and Charpentier, E. 2012. A programmable dual-RNA–guided DNA endonuclease in adaptive bacterial immunity. Science 337:816–821. doi: 10.1126/science.1225829
[7] ↑ Ebina, H., Misawa, N., Kanemura, Y., and Koyanagi, Y. 2013. Harnessing the CRISPR/Cas9 system to disrupt latent HIV-1 provirus. Sci. Rep. 3:1–7. doi: 10.1038/srep02510
[8] ↑ Mollanoori, H., Shahraki, H., Rahmati, Y., and Teimourian, S. 2018. CRISPR/Cas9 and CAR-T cell, collaboration of two revolutionary technologies in cancer immunotherapy, an instruction for successful cancer treatment. Hum. Immunol. 79:876–882. doi: 10.1016/j.humimm.2018.09.007
[9] ↑ Rezaei, H., Farahani, N., Hosseingholi, E. Z., Sathyapalan, T., and hossein Sahebkar, A. 2020. Harnessing CRISPR/Cas9 technology in cardiovascular disease. Trends Cardiovasc. Med. 30:93–101. doi: 10.1016/j.tcm.2019.03.005
[10] ↑ Bhardwaj, S., Kesari, K. K., Rachamalla, M., Mani, S., Ashraf, G. M., Jha, S. K., et al. 2021. CRISPR/Cas9 gene editing: New hope for Alzheimer’s disease therapeutics. J. Adv. Res. 40:207–21. doi: 10.1016/j.jare.2021.07.001
[11] ↑ Kaminski, M. M., Abudayyeh, O. O., Gootenberg, J. S., Zhang, F., and Collins, J. J. 2021. CRISPR-based diagnostics. Nat. Biomed. Eng. 5:643–656. doi: 10.1038/s41551-021-00760-7
[12] ↑ Hou, T., Zeng, W., Yang, M., Chen, W., Ren, L., Ai, J., et al. 2020. Development and evaluation of a rapid CRISPR-based diagnostic for COVID-19. PLoS Pathog. 16:e1008705. doi: 10.1371/journal.ppat.1008705
[13] ↑ Ding, R., Long, J., Yuan, M., Jin, Y., Yang, H., Chen, M., et al. 2021. CRISPR/Cas system: a potential technology for the prevention and control of COVID-19 and emerging infectious diseases. Front. Cell. Infect. Microbiol. 11:639108. doi: 10.3389/fcimb.2021.639108
[14] ↑ Hammond, A., Galizi, R., Kyrou, K., Simoni, A., Siniscalchi, C., Katsanos, D., et al. 2016. A CRISPR-Cas9 gene drive system targeting female reproduction in the malaria mosquito vector Anopheles gambiae. Nat. Biotechnol. 34:78–83. doi: 10.1038/nbt.3439
[15] ↑ Jaganathan, D., Ramasamy, K., Sellamuthu, G., Jayabalan, S., and Venkataraman, G. 2018. CRISPR for crop improvement: an update review. Front. Plant Sci. 9:985. doi: 10.3389/fpls.2018.00985
[16] ↑ McFarlane, G. R., Whitelaw, C. B. A., and Lillico, S. G. 2018. CRISPR-based gene drives for pest control. Trends Biotechnol. 36:130–133. doi: 10.1016/j.tibtech.2017.10.001
[17] ↑ Lino, C. A., Harper, J. C., Carney, J. P., and Timlin, J. A. 2018. Delivering CRISPR: a review of the challenges and approaches. Drug Deliv. 25:1234–1257. doi: 10.1080/10717544.2018.1474964
[18] ↑ Pausch, P., Al-Shayeb, B., Bisom-Rapp, E., Tsuchida, C. A., Li, Z., Cress, B. F., et al. 2020. CRISPR-CasΦ from huge phages is a hypercompact genome editor. Science 369:333–337. doi: 10.1126/science.abb1400
[19] ↑ East-Seletsky, A., O'Connell, M. R., Knight, S. C., Burstein, D., Cate, J. H., Tjian, R., et al. 2016. Two distinct RNase activities of CRISPR-C2c2 enable guide-RNA processing and RNA detection. Nature 538:270–273. doi: 10.1038/nature19802
[20] ↑ The Royal Society, National Academy of Medicine, & International Commission on the Clinical Use of Human Germline Genome Editing. 2020. Heritable Human Genome Editing. Washington, DC: National Academy Press.
[21] ↑ Baylis, F., Darnovsky, M., Hasson, K., and Krahn, T. M. 2020. Human germline and heritable genome editing: the global policy landscape. CRISPR J. 3:365–377. doi: 10.1089/crispr.2020.0082