Abstract
Chemists use atoms as the building blocks in reactions to design new molecules for materials, medicines, and much more. However, the chemical elements on our planet are a finite resource, so we need to prevent them from running out or being wasted. How do chemists do this? The principles of Green Chemistry guide chemists, helping them to design new products both efficiently and safely, while limiting or preventing pollution along the way. Scientists consider them through every step of creating a new product—from early experiments in the laboratory, all the way up to large-scale industrial production. Chemists can compare the materials and processes used to create their products using a Life Cycle Assessment, which can calculate factors such as water, energy, and resource inputs as well as production of pollutants like carbon dioxide. All of this means we can do exciting and important science while protecting our planet, too!
Sustainability: Thinking Of The Future
We are all humans living on the Earth, and it is important we understand how our actions affect the environment and other people across the planet. But can the Earth produce the resources we need as quickly as we are using them up? And how can we make sure those resources are used fairly, safely, and sustainably? The goal of sustainability is to avoid wasting resources that cannot be replenished as quickly as they are used up. Sustainability is essential in society to preserve and protect life on Earth for future generations. There is a long list of “R” words that describe possible strategies to improve sustainability: reduce, reuse, repair, rot, recycle… and many more. But how do scientists achieve sustainability in their experiments? Chemists make and break bonds in chemical reactions, to create new products with specific properties, like brighter colored paints or important medicinal properties. You might have heard of chemical elements like carbon, nitrogen, magnesium, and iron. The Earth’s crust and atmosphere contain varying amounts of each element, and these elements are a finite resource: they generally cannot be created or destroyed. If a product ends up in landfill, the elements it is made from are trapped and wasted. Some elements are also difficult to access in the first place: they may be trapped underground or in areas where there are wars or conflicts. This poses a huge challenge, for example, for obtaining the precious metals that smartphones, laptops, and other gadgets rely on.
Principles Of Green Chemistry
Chemistry that aims to be as sustainable as possible, to protect the Earth and its resources for future generations, and avoid wasting atoms, is called green chemistry. A set of guidelines called the 12 Principles of Green Chemistry was published in 1998. These guidelines help chemists develop sustainable processes and environmentally friendly products [1]. Figure 1 shows the questions scientists might ask as they design a new process, starting from the raw materials and moving toward the new product. The principles fit into two main categories: design for efficiency and design for environmental safety.
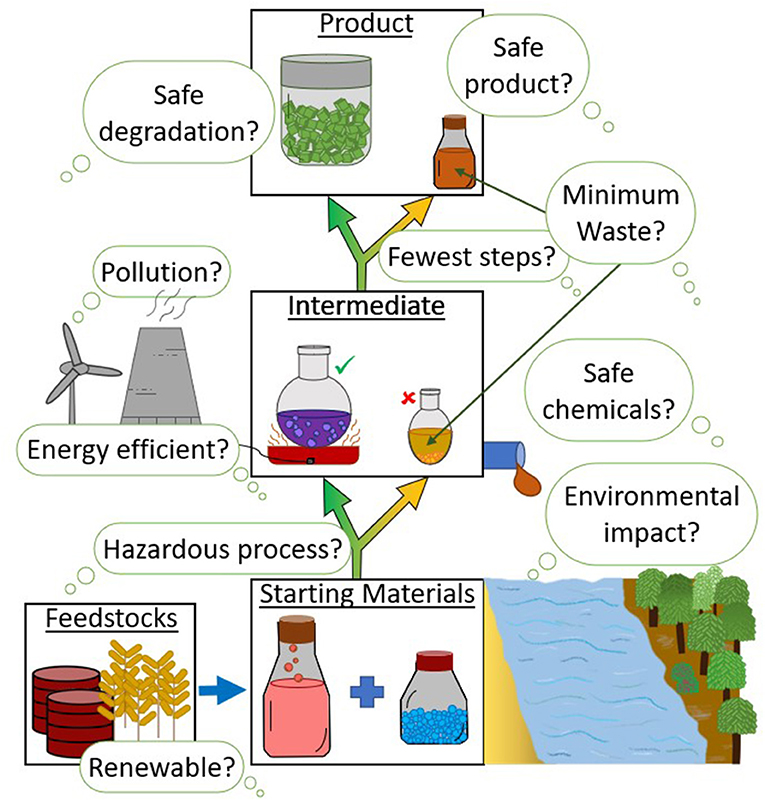
- Figure 1 - When creating a product from raw materials, scientists must ask multiple questions throughout the process to make sure they are following the 12 Principles of Green Chemistry.
- It is not always possible to make the desired product straight away. Sometimes other substances, called intermediates, must be made first, and their impact must be measured as well. Waste products (yellow arrows) can also be created.
Design For Efficiency
Firstly, what is efficiency and how do scientists measure it? For a process to be efficient, it must generate the desired product while limiting waste of atoms, materials, energy, and money. The first thing to consider is how much product is made (known as the reaction yield). Not all reactions form 100% of the intended product. For example, unwanted products (called by-products) may also be made, or the reaction may not run to completion, so not reaching a 100 % yield. Efficiency can be measured in many ways. The best way to measure it in terms of sustainability in chemistry is to consider what happens to each of the atoms in a process. Atom efficiency compares the number of atoms involved in a process with the number present in the final product. This allows scientists to determine how many of the atoms from the starting material have been incorporated into the desired product. During a chemical reaction, atoms may be lost as by-products or as waste (yellow arrows in Figure 1). By improving the efficiency of a process and reducing the total number of steps, scientists can limit the formation of unnecessary by-products and waste. However, sometimes by-products may be useful in another reaction, or for a separate purpose. For example, steam (gaseous water) produced as the by-product of a reaction could be used to turn a turbine to generate electricity, or the steam could be condensed into water, which could be used for another purpose.
Efficient energy use—and using energy from renewable sources, like solar or wind energy—is also important. A reaction that works at room temperature and does not require high pressure will require less energy. One approach for reducing the amount of energy needed by a chemical process is by using a chemical called a catalyst, which helps the reaction happen more easily. A catalyst allows the temperature and/or time of the reaction to be lowered without impacting the final product, but the atoms of the catalyst are not present in the structure of the final product. However, the catalyst must be separated from the final product so that it can be recycled for repeated use, which can use lots of energy and resources. Additionally, many common catalysts are made from rare elements (often metals), which may be difficult and expensive to access, so catalysts should be used in small amounts and recovered for repeated use where possible.
Finally, scientists aim to use renewable raw materials and generate recyclable products, to keep atoms in a circular system. When materials are thrown away after being used, the elements are wasted and cannot be reused, so new resources must be extracted. This is known as a linear economy. Recycling materials allows elements to remain available for future use. This is a key part of a circular economy. Moving from a traditional linear economy to a circular economy is important to make sure that finite elements are available in the future. Plant-based raw materials can be regenerated much faster than raw materials like oil or natural gas. They may even remove carbon dioxide (CO2) from the atmosphere as they grow. However, to be a sustainable choice, land for food production must not be sacrificed to grow crops for raw materials, and extracting the necessary raw materials from plant matter must also be fairly simple.
Design For Environmental Safety
The safety-related principles of green chemistry allow the impact of all stages of a chemical process on humans, wildlife, and the environment to be evaluated and minimized. This includes how products will act over longer periods of time, too. Designing products with their future degradation (breakdown) in mind is also critical. Degradation can be physical (breakdown into to smaller pieces) or chemical (breakdown into different molecules). Degradation can occur naturally, like composting, or with external help, such as melting, grinding, or chemical reactions.
Importantly, the product and its degradation products should be non-toxic, and should not be environmentally persistent. Ideally, all steps of the process, as well as the raw materials, products and by-products, should be as safe and non-toxic as possible. By considering the safety implications of a process while designing it, we can prevent harm to people and the environment.
Safety considerations also include hazards due to the use of high temperatures or pressures during the reaction process. Risk of fire as well as worker injury or illness must all be minimized to keep everyone safe and to limit harm to the environment due to the formation or release of pollutants. By thinking about the possible consequences of the products and processes during the design stages, scientists can remove or minimize hazards even before they begin. By using the 12 Principles of Green Chemistry [1], scientists are working to reverse the impacts of past pollution and avoid causing more pollution in the future too.
Measuring The Impact Of Chemical Processes On People And The Planet
Scientists need to be able to compare different chemicals and processes to understand and rank their impact and usefulness. Understanding how different chemicals may affect the people using them is very important. Some chemicals may be harmful to humans or the environment. The information in a chemical’s safety documents allows scientists to decide which chemicals may be the most sustainable and safe option for use in their products. Scientists will consider:
- What harm a chemical could cause to people or the environment,
- How much chemical would need to be present to cause that harm, and
- How to reduce the risk of using that chemical, possibly by monitoring exposure or using personal protective equipment.
Chemists often calculate the ratio of the amount of waste or “unwanted” by-product a process generates vs. the amount of desired product made, as a way of evaluating the product’s environmental impact. This is called the E-factor [2]. However, the E-factor does not consider the toxicity of any by-products, just the quantity generated. But what about how much CO2, a greenhouse gas that contributes to climate change, is created during production, or how long a product takes to break down in the environment? Figure 2 illustrates the stages in the lifecycle of a product that scientists must think about, beyond just the chemical reactions used to create it.
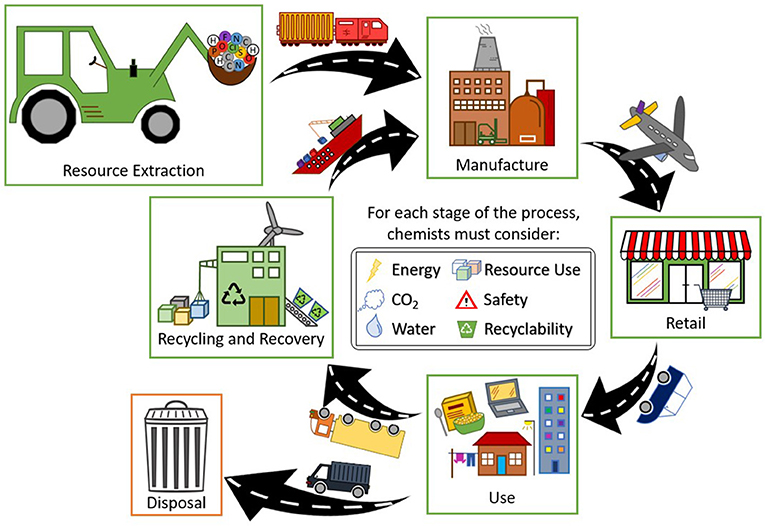
- Figure 2 - The lifecycle of a product has several stages, and transportation must occur between each stage.
- In the first stage, resource extraction, raw materials are collected. The manufacturing stage involves creating the product, and retail involves selling it. After products are used, they are either thrown away (disposal) or recycled so that their resources can be used again—this is preferable, as it makes the economy circular instead of linear [2].
Scientists can compare products more fully by completing a lifecycle assessment (LCA) [2]. Factors assessed for each stage of a product’s lifecycle can include the amount of energy required, the amount of water used or, most commonly, the amount of CO2 produced. The safety profiles of all the chemicals used to make the product are evaluated, as well as the sources of those chemicals. LCAs enable scientists to consider and compare all stages in a process, to ensure that the most sustainable process is chosen, which is especially important when developing large-scale industrial processes.
Conclusions
Earth’s elements are a finite resource. We all have a shared responsibility to use them as efficiently as possible, and to generate minimal environmental damage or waste in the process. The 12 Principles of Green Chemistry and methods of measuring impact such as lifecycle assessments provide scientists with tools to help them make informed decisions about sustainability when they are designing and conducting chemical reactions. Focusing on sustainability in current and future processes is vital to society. We must develop beneficial technologies without harming future generations and the planet.
Glossary
Sustainability: ↑ This is the practice of protecting the Earth’s resources, so they are still available to future generations. If something is sustainable, it means it will be around for a long time, without causing harm to the environment.
By-Product: ↑ An additional product made alongside the desired product during a chemical reaction. Historically seen as a waste product, but some by-products have their own uses.
Catalyst: ↑ A substance used in a chemical reaction that makes the reaction happen more easily without being used up itself.
Circular Economy: ↑ An system that tries to limit waste or pollution and keep materials in use; an alternative to the traditional linear economy in which materials are thrown away once used.
Degradation: ↑ When a product is broken down into smaller simpler parts.
Environmentally Persistent: ↑ Describes chemicals that are toxic or harmful and resistant to degradation. Once produced, they are difficult to remove from the environment and can continue to cause harm for a long time.
E-Factor: ↑ A ratio of the total waste of a process compared to how much useful product is made. The ideal E-factor is 0, and higher E-factors indicate a more wasteful process.
Lifecycle Assessment: ↑ A detailed analysis of the impact of a product on the environment throughout its lifespan, including extracting the necessary raw materials, making and using the product, and its disposal.
Acknowledgments
We would like to thank the EPSRC and SFI Center for Doctoral Training in Sustainable Chemistry (EP/S022236/1) for studentship funding. JS is also associated with the EPSRC Prosperity Partnership between Lubrizol and the Universities of Nottingham and Warwick (EP/V037943/1).
Conflict of Interest
The authors declare that the research was conducted in the absence of any commercial or financial relationships that could be construed as a potential conflict of interest.
References
[1] ↑ Anastas, P. T., and Warner, J. C. 1998. Green Chemistry: Theory and Practice. (Oxford: Oxford University Press). p. 30.
[2] ↑ Sheldon, R. A. 2017. The E factor 25 years on: the rise of green chemistry and sustainability. Green Chem. 19, 18–43. doi: 10.1039/C6GC02157C