Abstract
Did you know that the microscopic world has its own superheroes? These tiny heroes are called extremophiles. Extremophiles are microorganisms that can survive in the most dangerous places on Earth, such as hot streams or icebergs. Extremophiles can survive in these harsh environments because they have molecular tools called enzymes, which help them to perform important chemical reactions. While all organisms have enzymes, those of extremophiles are adapted to work under the extreme conditions in which they live, so we call those enzymes extremozymes. Extremozymes can help us produce drugs, food, and other products that we use every day! In the lab, we can make extremozymes even better by increasing their strength and durability through a process called immobilization. Using enzymes in industrial processes often helps to protect the Earth, because enzymes are generally more environmentally friendly than many man-made chemicals.
What Are Enzymes and How Can They Help us?
Humans have used microorganisms to their advantage since the very beginning of civilization. Baking bread depends on yeast cells feeding on the sugars present in the flour, and most alcoholic drinks are made by converting the sugars present in grapes and grains into alcohol. This was the beginning of biocatalysis: the very first examples of using of living organisms (or substances they make) to produce products that are useful for humans. But what if we need a reaction to happen at more extreme conditions, for example, at a high temperature? It would be hard for most microorganisms to survive in those conditions, and the reactions would not happen. This is because microorganisms like baking yeasts are mesophiles: organisms that live in “normal” conditions, such as the presence of water, temperatures between 20 and 45°C, and pH between 7 and 9 (Figure 1A). Mesophiles do not like changes in their environments, so they often cannot be used in situations where conditions are not as mild.
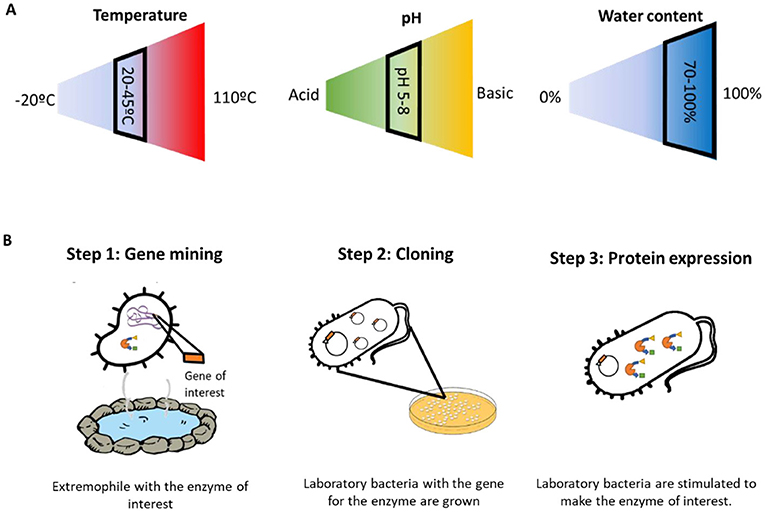
- Figure 1 - (A) Mesophiles are microorganisms that need “normal” conditions of temperature, pH, and water content to survive.
- These conditions are indicated by the black boxes. Extremophiles can live in harsh conditions that fall outside of the black boxes. (B) To perform recombinant expression in the laboratory, three general steps are followed. In the first step, the gene of interest is identified and “mined” from within the DNA of an extremophile. Then, that gene is cloned by placing it into a laboratory bacterial strain. The bacteria with the gene of interest are then cultured and stimulated to make the enzyme.
But not all habitats in the world have such pleasant conditions—imagine a volcano, or Antarctica, for example. Some microorganisms have evolved to survive in these harsh environments where mesophiles cannot: these microorganisms are called extremophiles. Extremophiles can live under frozen lakes, in thermal baths where the temperature is over 70°C, and even in nuclear waste containers! They are like the cartoon superheroes that have special powers. Extremophiles can survive in these environments because they have evolved internal tools that allow them to eat, grow, and thrive in harsh conditions.
When we talk about these internal tools, we are talking mainly about enzymes. Enzymes are proteins that act as a catalyst. They help the biological reactions in all living organisms to happen faster and easier—such as transforming the sugar you eat into energy for your body. Enzymes that come from extremophiles, which can work under harsh conditions, are called extremozymes. Cells produce enzymes by following a set of instructions written in the DNA. Scientists know enough about how this process works that they can manipulate it in the lab to produce any enzyme they desire! This technique is called recombinant expression. Normally, recombinant expression consists of three steps (Figure 1B). The first step is to identify the gene for the enzyme we are interested in, which is called gene mining. We look at the DNA of the extremophile until we find the gene that produces the enzyme that we want. In the second step, we isolate the gene in the lab and clone it, which means to make many copies. To clone a gene, we put the gene into another microorganism—one that we can easily work with in the lab. Finally, we are ready for step three: we use the microorganism with the cloned gene like a little factory, to produce lots of the enzyme. Using these three steps, we can produce many different enzymes in the lab, study them, and select the best ones for our needs.
What Can Extremozymes Do for us?
Although it is a lot of work to create extremozymes, these enzymes are very useful for us! There are many microorganisms that have evolved to live in various types of extreme conditions, so there is virtually always an enzyme that can help make industrial processes better. For example, extremozymes can be used in the food industry to prepare fruit juices, meats, or cheeses, and they can also be used in the pharmaceutical industry to produce medicines. The use of extremozymes in these processes often generates cleaner, safer products because we can for example use higher temperatures than would be allowed with mesophilic enzymes, helping to run the reaction better and faster, while doing everything in water. Using these enzymes can also make manufacturing processes more sustainable (causing very little or no damage to the environment) because enzymes often do a better, more efficient job than man-made chemicals, generating less waste and less pollution. The use of enzymes has some other very cool advantages: enzymes are more precise (selective), generating less waste and less undesired products.
Let us look at the example of extremozymes that can work under extremely cold temperatures. These enzymes come from extremophiles that live in environments with temperatures lower than 15°C. That is cold! When temperatures increase to just 30–40°C, some of these enzymes are inactivated. Imagine being able to switch on or off a process by simply shifting the temperature by a few degrees…this is a major plus in industry where “simple” is always a major advantage. And, you probably have some of these extremozymes in your house right now! Since the 1980s, they have been used in laundry detergents. Enzymes in detergents can help to remove stains from clothes [1]. Normally, stains are more easily removed using hot water, but when cold-tolerant enzymes are added to detergents, we can get the same clean, fresh clothes using cold water, which saves a lot of energy. So extremozymes not only make your laundry more environmentally friendly, but also cheaper!
How Can We Make Extremozymes Even Better?
But extremozymes are not a perfect solution. One of the main obstacles is that they are not reusable. Remember, enzymes are produced by living organisms, so they do not last forever—they become less active over time. This inactivation happens because the enzymes lose their shape. The specific shape of an enzyme is critical for the reaction it assists; like an athlete losing his fitness, it does not perform as well anymore. While this inactivation is normal in nature, it is not ideal for industrial applications. Making more enzymes takes time, energy, and resources, so it would be helpful to have enzymes that last longer. If, in a factory, we could re-use the same enzymes a hundred times, imagine how much time and effort would be saved. But how can we make enzymes more stable and reusable?
The main problem with reusing enzymes is that they are usually dissolved in a liquid and cannot be recovered. Imagine trying to separate the sugar from a cup of fresh orange juice—almost impossible to do! It is the same with an enzyme in solution. But, if we could stick the enzyme onto something that cannot be dissolved in water, we could then separate the enzyme from the liquid! In our orange juice example, if the sugar was stuck to the orange pulp, we could easily separate it from the juice with a strainer. The process of making the enzyme insoluble is called immobilization [2]. Over the years, many techniques have been invented to immobilize enzymes (Figure 2). There are three main types of immobilization: entrapment, which “traps” enzymes inside a bigger container that is insoluble—for example, inside an oil droplet that cannot dissolve in water; cross-linking, in which enzymes are linked to each other to create an insoluble net; and attachment, in which enzymes are stuck directly to the surface of a particle.
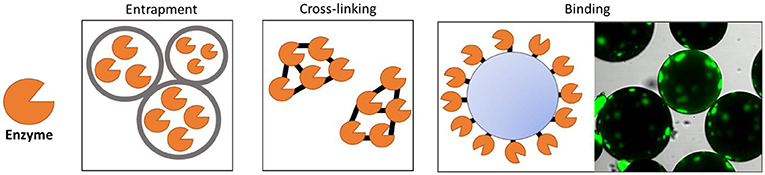
- Figure 2 - When enzymes are immobilized, they keep their shapes longer and can be recovered from industrial reactions and reused.
- There are three main ways to immobilize enzymes. They can be enclosed in a bigger structure, like an oil droplet, in a process called entrapment; they can be hooked to each other, using crosslinking; and they can be stuck to the surface of a particle, called binding. In the last image, you can see a real example of a fluorescent green enzyme immobilized by binding on the surface of a particle.
Each immobilization technique has advantages and disadvantages, so we must find the best strategy for each enzyme. In cross-linking and attachment, since the enzyme is linked to another particle it cannot move as before. This normally improves the resistance of the enzyme to temperature or other harsh conditions because it helps the enzyme keep its shape. But, at the same time, linking the enzyme can also cause it to be less effective at its job because it is less flexible. With entrapment, the enzyme is protected from changes in the environment inside a “bubble,” but it can move freely inside this bubble, so it usually does not lose its activity.
How Are Immobilized Enzymes Used?
A very good example of how immobilized enzymes are applied is the process of producing lactose-free milk. Lactose is a molecule present in cows’ milk that 60–70% of people cannot digest properly, making them lactose intolerant. Since milk is an important source of minerals and vitamins, removing the lactose from milk is a very good option. Lactose-free milk can be made by breaking down lactose with an enzyme, called lactase or β-galactosidase. One way that the milk industry gets rid of lactose is to add enzymes directly into the milk and then inactivate the enzymes by increasing the temperature. However, after inactivation, the enzymes are still in the milk, and this could cause problems such as allergies and of course does not allow the reuse of the enzyme a second time because you cannot separate the enzyme from the milk. But if the enzymes are immobilized, we could simply remove them from the milk using a big strainer! [3]. Recovery of an enzyme allows it to be reused with another batch of milk and also ensures that the lactose-free milk no longer contains the lactase. Even better, if we can use an enzyme that works at low temperatures, we can perform this reaction without affecting the taste and maintaining other important properties of the milk that are affected by the heating step.
But there is more to it as the use of immobilized enzymes goes beyond food! Some can be used in the production of biodiesel, for example. Biodiesel is a type of fuel generated from vegetable oils like palm, sunflower, or soybean oils. To produce biodiesel, we use immobilized lipases, which are enzymes that act on fats. The most famous immobilized lipase is called CALB, which stands for Candida antarctica lipase B [4]. As the name suggests, this is a cold-adapted enzyme but, surprisingly, it can be made resistant to high temperatures. When immobilized, it can withstand temperatures of up to 110°C and it can even work even without any water! This is very useful in the production of biodiesel, which occurs at high temperatures. Producing biodiesel using immobilized enzymes could help us reduce the use of fossil fuels and the pollution those fuels generate, helping to solve a major climate problem threatening our future.
Conclusion
Enzymes are a good example of how humans can use nature to their advantage. They have become extremely important tools for the eco-friendly production of products that fulfill our everyday needs. The ability to produce any enzyme in the lab, the discovery of extremozymes, and the development of technologies for enzyme immobilization have been ground-breaking. These advances could allow us to revolutionize many industrial processes. Nowadays, the processes to produce many of the goods we use every day are producing waste that pollute our planet. We are now facing a crisis and we must find ways to protect the Earth and its inhabitants. To do so, it is more important than ever to move toward more sustainable strategies in all that we can and making our vast system of industrial production more environmentally friendly by using enzymes could play a major role in reducing pollution.
Glossary
Biocatalysis: ↑ The use of living things or their machinery to make products important to humans.
Mesophile: ↑ An organism that develops and grows at mild temperatures (20–45°C), neutral pH (5–8) and in high water contents (more than 70%).
Extremophile: ↑ An organism that lives in extreme conditions such as very high or very low temperatures, for example, imagine volcanoes and glaciers.
Enzyme: ↑ A protein that speeds up reactions in your body but also in a chemistry lab. All living organisms have many kinds of enzymes.
Extremozyme: ↑ An enzyme that comes from an extremophile.
Recombinant Expression: ↑ Process of expressing an enzyme in the lab. In this process, a laboratory bacterium that contains the gene of interest is stimulated to produce the enzyme in the laboratory.
Sustainable: ↑ That causes very little or no damage to the environment.
Insoluble: ↑ That cannot be dissolved in water, like the pulp in an orange juice.
Immobilization: ↑ Process of sticking an enzyme together with an insoluble material.
Conflict of Interest
The authors declare that the research was conducted in the absence of any commercial or financial relationships that could be construed as a potential conflict of interest.
Acknowledgments
This work was supported by the SNSF (200021_192274 to FP).
References
[1] ↑ Sarmiento, F., Peralta, R., and Blamey, J. M. 2015. Cold and hot extremozymes: Industrial relevance and current trends. Front. Bioeng. Biotechnol. 3:148. doi: 10.3389/fbioe.2015.00148
[2] ↑ Romero-Fernández, M., and Paradisi, F. 2020. Protein immobilization technology for flow biocatalysis. Curr. Opin. Chem. Biol. 55:1–8. doi: 10.1016/j.cbpa.2019.11.008
[3] ↑ Basso, A., and Serban, S. 2019. Industrial applications of immobilized enzymes—a review. Mol. Catal. 479:110607. doi: 10.1016/j.mcat.2019.110607
[4] ↑ Ortiz, C., Ferreira, M. L., Barbosa, O., dos Santos, J. C. S., Rodrigues, R. C., Berenguer-Murcia, Á., et al. 2019. Novozym 435: the “perfect” lipase immobilized biocatalyst? Catal. Sci. Technol. 9:2380–420. doi: 10.1039/C9CY00415G