Abstract
Have you ever heard of black holes? Black holes sound like objects from a science fiction story. These objects are dark, dense regions in the universe, and their gravitational pull is so strong that nothing can escape them—not even light! This is why black holes are so black: without light, we cannot see them. Physicists think that black holes are some of the universe’s most exciting objects to study. Why? Because once something has fallen into a black hole, it can never return. And more fantastic still: the laws of physics do not tell us what happens when something falls into a black hole and reaches its center. In other words, black holes are huge cosmic mysteries. In this article, we present an analogy that helps us make sense of these mysteries. The analogy offers a new way of thinking about space and time.
What Are Black Holes?
Black holes are some of the universe’s most fascinating objects. Black holes are dark, dense regions in space where the pull of gravity is so strong that nothing can escape. Not even light can get out of these regions. That is why we cannot see black holes—they are invisible to our eyes. Because nothing can get out of black holes, physicists struggle understanding these objects. Not even the laws of physics tell us what happens when something falls into a black hole—at least not yet. Therefore, black holes remain cosmic mysteries, and many scientists work hard to solve the mystery of black holes.
To understand what is going on with black holes (Figure 1), we first need to learn more about space and time. Hardly anyone understood space and time better than Albert Einstein, the famous physicist with wild curly hair. Although Einstein used advanced mathematics to develop his ideas about the universe, we can grasp basic elements of Einstein’s theories using pictures, analogies, and our imagination. Analogies are valuable learning tools in physics because they compare abstract science concepts to familiar concepts we know from everyday life [1]. This article uses a river analogy to explore why we cannot escape a black hole [2].
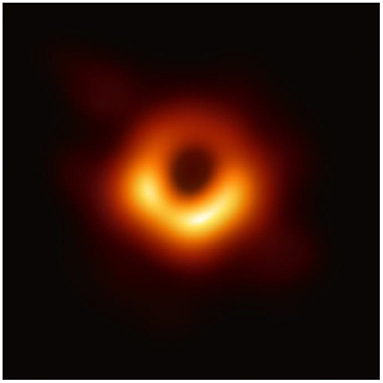
- Figure 1 - Black holes are regions in spacetime in which gravity is so strong that nothing can escape—not even light.
- This is why black holes are black and why we can only see the surrounding matter in this picture (photo credit: Event Horizon Telescope CC BY 4.0).
What Is Spacetime?
Let us start with general relativity, which is Einstein’s theory that links space and time to gravity. The theory is based on Einstein’s insight that we should not think of space and time as separate but rather as connected elements that make up spacetime. Spacetime is our way of describing all events in the universe—where they happen and when they happen. For example, if you want to meet your friends after school, you need to agree on where you want to meet (the place) and when to meet (the time). Thinking of all places and all times in the universe taken together is conceptually challenging—the universe is so vast, after all! How can we picture spacetime?
Spacetime Flows Like a River
Luckily, an analogy can come to our rescue as we try to understand spacetime. This analogy compares the structure of spacetime to the flow of water [2–4]. Spacetime far away from planets and stars is flat and smooth without any movement, just like a large, quiet lake. Objects and people can freely float in this lake. You can stay still, or you can swim in any direction easily. This scenario is similar to astronauts floating freely in outer space, where they can easily move up and down and left and right.
This scenario changes when we look at spacetime surrounding massive objects such as planets, stars, and black holes. Spacetime close to these cosmic objects is no longer still and smooth but curved and whirly, just like strong streams or rivers. Just imagine that there are strong streams flowing within certain parts of a lake. You cannot see or perceive their presence when you are very far away from these currents because the water around you remains still. But if you swim to a different part of the lake, near one of the currents, you can start feeling the current dragging you with it. In the same way, astronauts feel the gravitational pull of planets if they come too close.
If the current is very weak, you are near a planet-stream, like spacetime around the Earth. But the pull becomes stronger near a star-river, like spacetime around our Sun, and much more intense near a black-hole torrent. The closer you are to the current, the faster you need to swim if you want to escape its strong pull. You need to exert effort to go back to the quiet zone of the lake, where you can stay still and float around to sunbathe. The same is true for spacetime: the closer you are to a cosmic object, the harder it is to escape the gravity that pulls you toward that object.
A Better Explanation of Gravity
The river analogy illustrates a key insight in Einstein’s theory, namely that space and time are linked to gravity. Gravity is what makes apples fall from trees and what keeps you stuck to the ground. You have probably learned that gravity is an attractive force between massive objects: the mass of the Earth pulls everything to its center, and it is this pull that we associate with the force of gravity.
This description of gravity is a pretty good explanation of what is happening on Earth. But Einstein realized that an even better explanation could describe all gravitational processes in the universe: a spacetime explanation! According to Einstein’s theory, gravity shapes the structure of spacetime. Massive objects like planets and stars tell spacetime how to curve, and curved spacetime tells these objects how to move. With the river analogy, this interplay between spacetime and gravity is easy to understand. Moving through spacetime is like swimming in water. If there is no planet or star, you swim in a lake. The water is calm and you can float around in the same way as an astronaut floats in outer space. Close to a planet, the water is like a river. You are pulled along with the river, just like a close object in space would be pulled down to the ground here on Earth.
Einstein’s theory about gravity was revolutionary because it offered a better way of thinking about our universe. General relativity also predicted the existence of black holes, which are regions where the spacetime torrent is so strong that nothing can escape it, not even light. It is like a cosmic one-way street: objects can fall into a black hole, but nothing can ever return. Strange, isn’t it? At first, Einstein thought black holes were too strange to be true. Maybe the equations were playing a trick on him, and he hadn’t understood them fully. But the longer Einstein and other physicists studied the equations, the more convinced they were that black holes exist.
Falling into a Black Hole
Of course, we are not going to use equations to explain black holes to you. We will use the less complicated river analogy. Let’s see how the river analogy helps us understand what is going on if you fall into a black hole. Imagine you are back in the lake, and the lake borders a river with a strong current. If you come too close to the river and do nothing, the current drags you into the river. You start moving with the river, and your speed gradually increases. You might get tired of being carried along by the river and decide to swim back to the lake. You notice, however, that the farther you are from the lake, the faster you need to swim to get back. And your effort depends on where you are: escaping from a planet-stream is easier than from a star-river which requires a greater effort. But if you are in shape, you can always do that!
Black-hole torrents have a much stronger current than planet streams or star rivers, and black-hole torrents are special in another way: they form waterfalls! As you approach the waterfall, nothing seems strange or unusual: your speed continues to increase, and you are carried further down the stream. But once you have crossed the waterfall, you realize that you cannot return (Figure 2)! Even if you swim with great energy, you cannot swim back up the waterfall—the current is too strong and always drags you away. Your friends cannot help you either: they are up above the waterfall and can’t hear or see you down below.
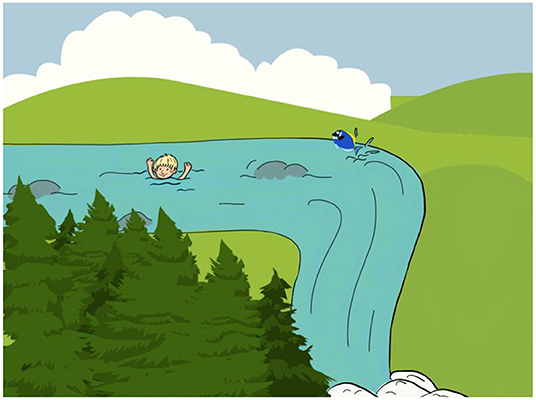
- Figure 2 - The river analogy compares the event horizon of a black hole to a waterfall: once you have fallen down a waterfall, you cannot swim back up to the top of it.
- The same is true for black holes—you cannot return once you have crossed the event horizon.
Physicists observe a similar phenomenon around black holes and they call it the event horizon. Just like the waterfall marks a point of no return in our imaginary lake, the event horizon marks a point of no return around a black hole. Instead of water, it is gravity that drags you down and prevents you from returning. You can picture the event horizon as a surface surrounding the black hole. Anything outside this surface —including astronauts, rockets, or light—can escape from the black hole. But once this surface is crossed, nothing can escape, regardless of its speed, because of the strong gravitational pull toward the center of the black hole. Not even light can escape—and light is the fastest thing in the universe! Since light cannot escape, you cannot see what is inside the event horizon. It is like the horizon of the Earth, beyond which you cannot see [5]. This is why physicists are so fascinated by black holes: they know that objects continue to exist after they have crossed the event horizon, but there is no way for scientists to observe exactly what is going on because gravity is so strong.
Conclusion
Black holes and event horizons might sound mysterious at first. Luckily, the river analogy helps us unveil part of the mystery. Thinking with analogies is an awesome trick that many physicists use to explore complicated physics concepts. The river analogy is mathematically correct [2], but we do not need to understand spacetime mathematics to use the analogy. Instead of studying complicated spacetime equations, we imagine spacetime around a black hole like a river. We then explore the properties of black holes and event horizons by imagining ourselves swimming in this river and falling down a waterfall. Naturally, such a simplification can sometimes also mislead our imagination [1, 4]. That is why physicists need to combine their imagination with mathematics to fully understand the world around them. If you train your imagination and maths skills, who knows—you might solve the remaining mysteries of black holes that still puzzle physicists today!
Glossary
Black Holes: ↑ A region of spacetime in which gravity is so strong that no light can escape. Albert Einstein’s theory of gravity predicts the existence of black holes.
Analogies: ↑ Analogies are comparisons that describe one thing in terms of another. Physicists use analogies to better understand and communicate complicated concepts (e.g., black holes) in terms of familiar ideas (e.g., rivers).
General Relativity: ↑ Albert Einstein’s theory of gravity, in which matter (planets, stars, etc.) tells spacetime how to curve, and curved spacetime tells matter how to move.
Gravity: ↑ Often thought of as an attractive force between massive objects, modern physics describes gravity as the curved geometry of spacetime.
Spacetime: ↑ The fabric of the universe, composed of the connected elements of space and time. Physicists use the concept of spacetime to describe events in the universe.
Event Horizon: ↑ The region around a black hole which, when crossed, prevents an object from moving away from the black hole. This is like falling down a waterfall: you cannot swim back up.
Conflict of Interest
The authors declare that the research was conducted in the absence of any commercial or financial relationships that could be construed as a potential conflict of interest.
References
[1] ↑ Kersting, M., and Steier, R. 2018. Understanding curved spacetime - the role of the rubber sheet analogy in learning general relativity. Sci. Educ. 27:593–623. doi: 10.1007/s11191-018-9997-4
[2] ↑ Hamilton, A. J. S., and Lisle, J. P. 2008. The river model of black holes. Am. J. Phys. 76:519–32. doi: 10.1119/1.2830526
[3] ↑ Taylor, E. F., and Wheeler, J. A. 2000. Exploring Black Holes: Introduction to General Relativity. San Francisco, CA: Addison–Wesley Longman.
[4] ↑ Pössel, M. 2021. “Models and analogies in teaching general relativity,” in Teaching Einsteinian Physics in Schools, eds M. Kersting and D. Blair (New York, NY: Routledge). p. 145–59.
[5] ↑ Thorne, K. S. 1995. Black Holes & Time Warps. Einstein’s Outrageous Legacy. New York, NY: W.W. Norton & Company.