Abstract
The axons of our nerves are like long wires that carry important communication signals around the body. Axons are not alone in nerves; they are surrounded by special cells called Schwann cells. This companionship allows our bodies to do things such as go to school, catch a ball, and eat. In some people with illnesses called neuromuscular diseases, axons fall seriously sick and may wither away. We discovered that when axons become sick, Schwann cells quickly detect the issue. The Schwann cells act as an emergency-response team that delivers an extra supply of special sugars to axons in need. This sugar-boost makes harmed axons stronger and allows them to recover. We hope that this discovery will lead to novel therapies that will help keep axons alive in sick people.
Cellular Teamwork!
Everyone who has watched Paw Patrol knows that this Canadian TV cartoon is all about communication and teamwork, to help friends and neighbors in trouble. Like in Paw Patrol, the cells in our bodies act as a team. They combine their efforts to allow us to perform all of our daily activities, as well as to grow and repair damage done to our bodies. A legendary physiologist, Theodor Schwann, once described the importance of this cellular companionship. He wrote: “The whole organism subsists only by means of the reciprocal action of the single elementary parts” [1].
What happens if one part of this system becomes diseased and does not work well? When cells or tissues become sick, the cellular teamwork gets stronger. In other words, troubled cells call for help from other cells. These cells then work together to fix the problem. The help can occur through the exchange of nutrients or fuels needed to maintain cellular energy metabolism.
Energy metabolism is the process in all our cells that breaks down food to produce energy, through chemical reactions. Energy metabolism is absolutely necessary for life. What if there are not enough fuels available in one cell type? Would this stall cellular metabolism? No, because other cells intervene and transfer new fuels. A clear example of this teamwork occurs during intense exercise or hunger [2]. Desperate cells of the heart, brain, or muscles call for help from the liver. Liver cells then release special emergency fuels, which are transported through the blood to reach the organs and cells in need.
Axons and Schwann Cells, Besties Forever
Axons are fingerlike extensions of neurons (nerve cells) in our nervous system. To clearly observe axons in the laboratory, neurons can be removed from the nervous system and transferred into a special dish with a barrier (Figure 1). This barrier allows the separation of the neuronal cell bodies and the axons. The axons can then be studied in detail through a microscope. Scientists use this system as a simple model to simulate the behavior of neurons in the nervous system.
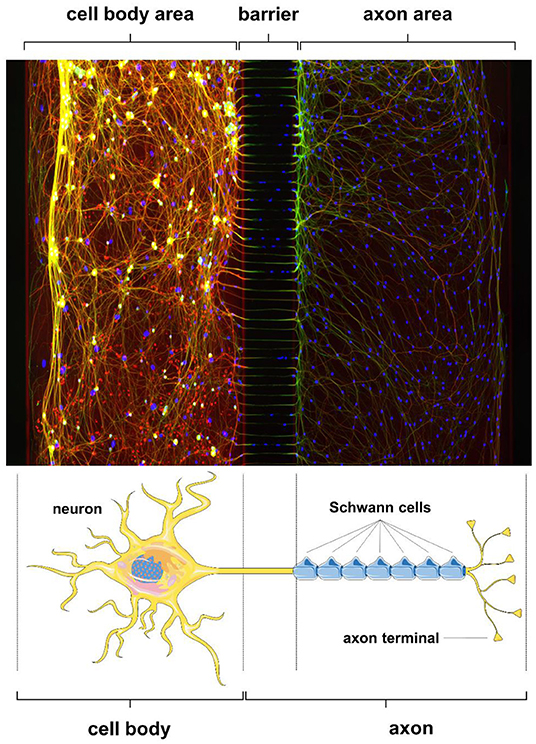
- Figure 1 - Neurons in a cell culture dish, with their cell bodies and axons separated by a barrier.
- The barrier helps researchers to study cell bodies and axons separately. The blue dots in the cell body area show the position of the neuron cell bodies. The blue dots in the axon area indicate the position of Schwann cell bodies that the axons associate with (Photograph credit: Elisabetta Babetto). Each axon is encased by numerous Schwann cells as shown in the schematic that illustrates the general structure of a neuron (bottom).
Typically, in our bodies, the axon of one neuron connects with another neuron or cell type. This connection allows the axon to speak to another neuron, or cell. They communicate using a language made by electrical and chemical signals. For instance, axons talk to muscle and sensory cells which allows us to move and feel.
However, the pictures in Figure 1 demonstrate that axons are actually much thinner and longer than fingers. Did you realize that axons with their tips (axon terminals, Figure 1 bottom, right, Figure 2B) make up the largest part of the neuron [3]? In the human body, axons can be up to one meter long! If you imagine the neuronal cell body as the size of a tennis ball, the axon would be many miles long! To stay alive and healthy, axons must receive crucial materials from the neuronal cell body. The process of transporting materials along axons has been described in a previous Frontiers for Young Minds article [4]. Since the transport of these materials along axons can be a very long journey, it uses a lot of energy. This is especially true for the lengthy axons in the system of nerves outside of the brain and spinal cord, which is called the peripheral nervous system (PNS). Nerves are like long cables that carry bundles of axons tightly packed together (Figure 2A). Such particularly long axons in nerves can break very easily.
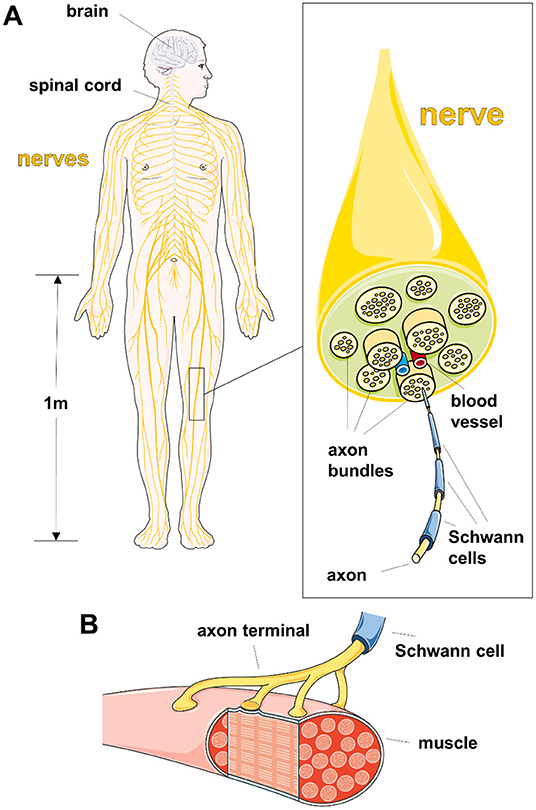
- Figure 2 - (A) Nerves (yellow) of the peripheral nervous system run outside the brain and spinal cord.
- Hundreds to thousands of long axons run in bundles inside nerves. Each axon is surrounded by a multitude of Schwann cells. The axons in a human leg can be up to one meter long. Neuronal cell bodies are found in the spinal cord, whereas the axon terminals (tips) can reach all parts of the body. (B) The axon tips or terminals contact other cells, such as muscle cells, which enables us to move.
Nature has designed unique cells, called Schwann cells (named after the aforementioned Theodor Schwann, who discovered them), which support axons in various ways. Schwann cells embrace axons to protect them (Figures 1, 2). This close contact nourishes and strengthens the axons within the nerves of the PNS. Schwann cells can deliver important nutrients to the axons, which is much faster for the axons than getting nutrients from the far-away neuronal cell body. Typically, many thousands of Schwann cells are associated with each axon, creating an important buddy system.
Axons Get Sick Early in Some Diseases
Like any flimsy and delicate structure, axons break first when things go wrong. What happens if axons deteriorate? Remember that many neurons are linked together in series by their axons. Even if only one axon in the chain breaks, the normal flow of information along the nerve can be disrupted.
Research in the laboratory and in patients has shown that axons are damaged early in many illnesses of the nervous system. This ranges from Alzheimer’s and Parkinson’s disease, Multiple Sclerosis and Traumatic brain injuries [5], to neuromuscular diseases such as motor neuron disease and peripheral neuropathies [6]. Peripheral neuropathies can affect the movement of the arms and legs, through the destruction of axons that connect to muscles. The disconnected muscles become weak, floppy, and eventually shrink over time. Sometimes breathing becomes difficult and heartbeat weakens because the axons connecting to the lungs and heart muscles degenerate.
These are some of the worst illnesses a person can have and, at present, there are no cures for them. Researchers around the world at Universities and drug companies are working hard to understand why and how axons die. With this knowledge, they try to find ways to delay or prevent the degeneration of axons. A recent study has shown that the decline of energy in injured axons explains why they eventually fall apart [7]. We asked: “Is it possible to protect injured axons by providing energy from outside of the neuron?” If so, we next asked: “Could Schwann cells provide this energy to injured axons?” We also asked these questions because we were inspired by the general principle of metabolic fuel exchange between different cells, and the cellular teamwork laid out in the introduction. We were additionally motivated by our earlier discovery that perturbation of energy metabolism in Schwann cells causes axon degeneration [8].
How Do Schwann Cells Help Injured Axons?
Indeed, using a variety of methods and neuroscientific models, we discovered that Schwann cells do come to the rescue!
We found that injured axons develop an increased appetite for certain special sugar molecules called pyruvate and lactate. When they sense injured axons, Schwann cells produce greater amounts of pyruvate and lactate, through a process called glycolysis. The sugars are then taken up by injured axons as fuels to produce energy in their mitochondria to promote axonal recovery (Figure 3). In other words, the Schwann cells become a kind of energetic emergency-support system for injured axons. We believe this is a natural way to help six axons survive. Natural axon damage occurs during the movement of nerves in our limbs or through aging, for example.
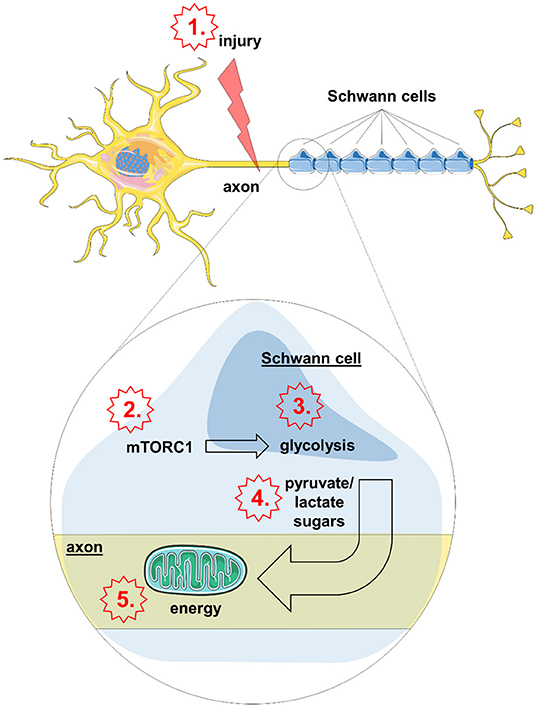
- Figure 3 - Axon injury (1) leads to activation of mTORC1 (2) in Schwann cells.
- This activates glycolysis (3) and results in the increased production of the sugars pyruvate and lactate (4) which are then transported from the Schwann cell into the axon for energy production in axonal mitochondria (5). This process counteracts the energetic failure of axons and their breakdown.
What will happen if we change glycolysis itself in Schwann cells? What about if we change the release of sugars from Schwann cells? We found that the degeneration of injured axons speeds up or slows down in such situations. We also identified what sparks the Schwann cells to change their glycolysis. It is a small but mighty molecular signal made from a large protein body known as mTORC1 (mammalian target of rapamycin complex 1).
Can we then alter the activity of this signal so that it protects axons in illnesses? To test this, we sickened axons in laboratory mice with a toxic drug known as acrylamide. This substance causes axon disease in human nerves. We forced Schwann cells to make more sugar by increasing the mTORC1 signal over a longer time. This had a soothing effect on axons wounded by acrylamide. In this way, it was possible to protect injured axons for the first time from outside of the neuron.
Summary and Future Research
We now know that injured axons in nerves degenerate due to problems obtaining enough energy. Axons form unique relationships with Schwann cells. The close bond between axons and Schwann cells supports the axons. We have found a way to protect sick axons through the increased supply of energy in the form of sugars released from Schwann cells. It is exciting to have found a cell type that controls axon health in this intriguing way. For many years, researchers focused on protecting axons mainly through treating the neuron itself. We now have knowledge of an additional mechanism to improve axon health!
How can our findings be used to help human patients? In the future, this knowledge could be applied to fight axon loss in patients with diseases of the nervous system. It may be possible to increase glycolysis and sugar release from human Schwann cells, to help axons recuperate from injury. Or perhaps we could directly administer the axon-protective sugars to patients, to ease their symptoms. It will also be interesting to see whether similar mechanisms protect axons in the brain and spinal cord, in addition to axons in nerves. The cells surrounding such axons are known as oligodendrocytes and astrocytes. Do these cells together maintain axons by providing sugars as well (similar to Schwann cells)? There is still much to be studied to ultimately help patients with diseases in which axon degeneration plays a key role. But we have strong hopes that no axon is too long, and no Schwann cell is too small to help save the day for many sick people!
Funding
This work was supported by Muscular Dystrophy Association Grant Nos. 577844 (to BB) and 292306 (to EB), and start-up funding for BB and EB provided through Empire State Development Corporation for Hunter James Kelly Research Institute Grant Nos. W753 and U446 and the Hunter’s Hope Foundation.
Glossary
Peripheral Nervous System: ↑ The division of the nervous system outside the brain and spinal cord.
Alzheimer’s Disease: ↑ A neurodegenerative disease with axon swelling and degeneration of axons in the brain that causes poor memory and thinking.
Parkinson’s Disease: ↑ A neurodegenerative disease with degeneration of midbrain axons resulting in shaking and stiffness of limbs.
Multiple Sclerosis: ↑ A neurodegenerative disease of the brain and spinal cord in which axon fibers degenerate due to inappropriate immune attacks.
Peripheral Neuropathies: ↑ A large group of neurodegenerative diseases of the peripheral nervous system in which nerve axons degenerate for a variety of reasons.
Glycolysis: ↑ A central metabolic pathway that breaks down the sugar molecule glucose to produce two of a smaller sugar molecule called pyruvate. Pyruvate can then be converted to lactate.
Mitochondria: ↑ Organelles in our cells that produce most of the energy to power the cells’ biochemical reactions.
mTORC1 (mammalian Target of Rapamycin Complex 1): ↑ A central signaling instructor in all of our cells that controls metabolism and growth.
Oligodendrocytes: ↑ Cells with similar functions as Schwann cells in the peripheral nervous system. Oligodendrocytes directly surround axons in the brain and spinal cord.
Astrocytes: ↑ Star-shaped cells in the central nervous system that are closely associated with neurons to provide nutrients and regulate the transmission of electrical signals.
Conflict of Interest
The authors declare that the research was conducted in the absence of any commercial or financial relationships that could be construed as a potential conflict of interest.
Acknowledgments
We are grateful for the support of mentored undergraduate level research at the University at Buffalo through the Experiential Learning Network (ELN) program. We thank Anthony Curione for participating in the preparation and initial stages of this project. Some parts of Figures 1–3 were generated using templates from Servier Medical Art (https://smart.servier.com), and adapted using Microsoft PowerPoint.
Original Source Article
↑Babetto, E., Wong, K. M., and Beirowski, B. 2020. A glycolytic shift in schwann cells supports injured axons. Nat. Neurosci. 23:1215–28. doi: 10.1038/s41593-020-0689-4
References
[1] ↑ Schwann, T., and Smith, H. 1847. Theory of the Cells, Microscopical Researches Into the Accordance in the Structure and Growth of Animals and Plants. London: Sydenham Society.
[2] ↑ Cantrell, C. B., and Mohiuddin, S. S. 2020. Biochemistry, Ketone Metabolism. Treasure Island, FL: StatPearls.
[3] ↑ Beirowski, B. 2013. Concepts for regulation of axon integrity by enwrapping glia. Front. Cell. Neurosci. 7:256. doi: 10.3389/fncel.2013.00256
[4] ↑ Sleigh, J. 2020. Axonal transport: the delivery system keeping nerve cells alive. Front. Young Minds. 8:12. doi: 10.3389/frym.2020.00012
[5] ↑ Morrow, E. L., and Duff, M. C. 2020. What is recovery like after traumatic brain injury? Front. Young Minds. 8:559017. doi: 10.3389/frym.2020.559017
[6] ↑ Coleman, M. P., and Perry, V. H. 2002. Axon pathology in neurological disease: a neglected therapeutic target. Trends Neurosci. 25:532–7. doi: 10.1016/s0166-2236(02)02255-5
[7] ↑ Yang, J., Wu, Z., Renier, N., Simon, D. J., Uryu, K., Park, D. S., et al. 2015. Pathological axonal death through a MAPK cascade that triggers a local energy deficit. Cell. 160:161–76. doi: 10.1016/j.cell.2014.11.053
[8] ↑ Beirowski, B., Babetto, E., Golden, J. P., Chen, Y. J., Yang, K., Gross, R. W., et al. 2014. Metabolic regulator LKB1 is crucial for Schwann cell-mediated axon maintenance. Nat. Neurosci. 17:1351–61. doi: 10.1038/nn.3809