Abstract
Axons are the thin and long extensions of neuronal cells that snake away from the cell body. Axons in our nervous system are like bridges spanning long distances to bring information from one nerve cell to another. However, axons are alive and need nutrients and energy to survive. Axons degenerate if nutrients and energy run out. This results in devastating symptoms in many conditions called neurodegenerative diseases. Scientists must understand why and how axons degenerate to prevent or delay it from happening. Recent studies have identified a destruction process that leads to energy loss and death of injured axons. The destruction pathway is completed by a rogue “demolition crew” driven by the watchdog molecule SARM1. Blocking this demolition crew delays the death of injured axons and helps sick axons. These findings may lead to new therapies that can decrease the symptoms of many people suffering from neurodegenerative diseases.
Introduction
It is time for dinner and you hear your mother call you into the kitchen. You spring up off of the couch when all of a sudden—YOW!—your foot lands right on one of your younger brother’s spiny dinosaur toys! You may not realize it, but in this short time, countless messages were sent throughout your body telling it to move your legs, pull your foot away, and, unfortunately, feel the pain (Figure 1).
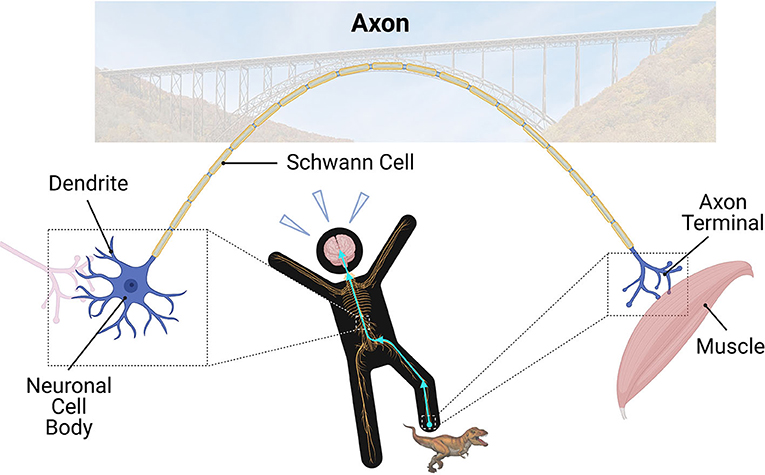
- Figure 1 - In the nervous system, dendrites receive information from other neurons.
- The cell body is the control center of the neuron. Axons are the long “bridges” of the nervous system; they relay electrical and chemical signals to other neurons through the axon terminal. Finally, Schwann cells create a myelin sheath around larger axons to keep the electrical signal strong in nerves. When you step on something painful, the signal travels up the nerves in your leg to the cell body in your spinal cord, and eventually makes its way to your brain. Ouch! After that, the signal then travels down the nerves to your muscle to move your leg.
The nervous system, which is made of the brain, spinal cord, and a bunch of nerves, is in charge of sending these messages. The nervous system works all the time without us realizing it. Anytime you catch a ball, feel the soft fur on a dog, or take a math test, your nervous system is at work! The brain enables us to learn and think. The spinal cord and nerves send messages from the brain to the body and send information to the brain from the outside world.
How does our nervous system do all of these amazing things? Special cells called nerve cells or neurons communicate information to the rest of our nervous system using chemical and electrical signals.
Neurons have four major parts: dendrites, cell body, axon, and the axon terminal (Figure 1). Dendrites are the small branches of the neuron that receive incoming messages from other neurons they are connected with. The signals then travel through the neuron’s cell body, which is the control center for the cell’s activities. Eventually, the signals reach the largest part of the neuron: the axon. Axons extend a long way out from the cell body and relay the messages to other neurons or targets. This occurs at the end of the axon, called axon terminal. Just like how bridges allow cars to drive to reach land on the other side, axons are important “bridges” of the nervous system (Figure 1). They allow electrical and chemical signals to travel up to 1 m (3 feet) away! No other cell type in your body can do this!
Larger axons are covered by a myelin sheath. This keeps the electrical signal strong and gives it a speed boost. The myelin sheaths are like the insulation of electric cables in the walls of your house. In nerves, special cells called Schwann cells help make the myelin sheaths (Figure 1). In the brain and spinal cord, myelin sheaths are produced by cells known as oligodendrocytes. Axons grouped together in bundles surrounded by myelin look white when examined under the microscope. Therefore, we call areas with these structures “white matter.”
When Axons Get Sick
Sometimes things go wrong in neurons. Since axons are long, skinny, sensitive structures, they are typically the first to break. Axons are also very “hungry.” They need lots of energy to keep electrical and chemical “traffic” flowing smoothly. Newer studies have discovered that some of the energy axons require comes from the surrounding Schwann cells and oligodendrocytes.
When there is not enough energy, the traffic along axons can be affected. This contributes to axon death. As explained before, axons are important for proper communication between neurons. If too many axons decay, it can disrupt the communication between neurons. Imagine if several basketball players on the same team were injured at once. It would be very difficult for the rest of the team to play well! Similarly, axon degeneration can disrupt the team of neurons.
In fact, axon degeneration is an early and central event in many neurodegenerative diseases. For example, degeneration of axons that connect to muscles (Figure 1) is a cause of amyotrophic lateral sclerosis (ALS). This leads to very weak muscles, making it difficult for patients to move and breathe. Another example is Alzheimer’s disease in which important brain axons and their terminals degenerate. This results in problems with memory and thinking. In the eye disease glaucoma, axons in the optic nerve behind the eyeball die. This can cause blindness. Multiple Sclerosis is another disease that causes many devastating symptoms. We are not sure why, but the body’s immune system incorrectly attacks myelinated axons in this condition. Currently, neuroscientists are studying axon degeneration to try to find a way to reduce it. In this way, they hope to help people who live with neurodegenerative diseases.
The Axon Demolition Crew
Let us think about the axon like a long bridge again. If severe damage to an axon occurs, the “bridge” becomes weak and electrical and chemical “traffic” can no longer drive over it safely. A ravaging demolition crew is then unleashed to tear down the bridge because, in the body’s opinion, it is better to tear down a badly damaged bridge than to try to maintain a broken one.
The process by which the body destroys the damaged portion of an axon is called Wallerian degeneration (Figure 2). The British scientist Augustus Waller first described this process while looking at cut frog nerves through a microscope [1]. Can you imagine how difficult this must have been with the technology of 170 years ago? Despite this, Augustus Waller found that injured axons in the cut frog nerve break into pieces in a speedy degeneration process. Cells other than neurons help disassemble the axons.
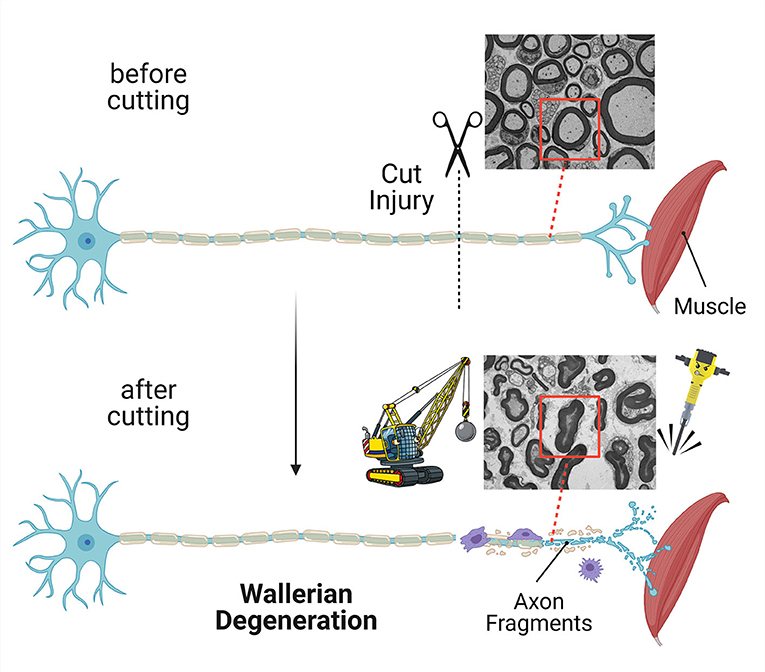
- Figure 2 - If an axon is cut, the part separated from the neuron cell body is quickly destroyed in a process called Wallerian degeneration.
- Other cells help with the demolition and removal of the axon. In this image, a neuron loses its communication with a muscle. Electron micrograph images show cross-sections of nerves before and after the axon was cut. You can see that, after cutting, the axon looks demolished (red boxes).
In laboratory mice, within 2–3 days after injury the destruction process is finished for thousands of disconnected axons in a cut nerve [2]. It is even faster if we look at single axons only. They are rapidly destroyed within a few hours [2]. Now that is fast and furious! Think of Wallerian degeneration as an express demolition program—instead of leaving the bridge to crumble and decay over time, the demolition crew uses their tools to quickly tear down the bridge. Afterwards, a cleanup crew arrives to clear away the axon remains. This helps with the construction of new axons.
Is Wallerian degeneration similar to the axon degeneration happening in neurodegenerative disease? In the last three decades, neuro-scientists found that, through a microscope, the axon degeneration in the nerves of diseased patients looks like Wallerian degeneration. This is why they call the axon demise in neurodegenerative disease Wallerian-like degeneration, even though cutting of axons is not involved. Additionally, they discovered that special genetic mutations that block the demolition program in mice greatly suppress both Wallerian degeneration and Wallerian-like degeneration [3]. Imagine axons, separated from their cell bodies, that can survive for weeks in the cut nerves of mutant mice. The same mutations also delay the degeneration of diseased axons and reduce the symptoms of neurodegenerative diseases in some cases. It is also interesting that the same mutations cause the same effects in other species, like the fruit fly. This tells us that axon destruction in various species is controlled by the same demolition program. Importantly, these results show that a cut nerve can be used as a helpful model to understand Wallerian-like degeneration in neurodegenerative disease.
How Does Axon Demolition Work?
Although you might prefer to eat candy, your parents remind you to eat your fruits and vegetables because those foods contain important nutrients that give us energy and help us stay strong. For axons to stay strong and healthy, they need a special nutrient molecule called NAD. NAD plays an important role in the axon’s ability to produce the energy it needs to survive. The energy is generated through the pathways glycolysis and mitochondrial respiration, which require NAD to function. NAD is made from the molecule NMN by the enzyme NMNAT2. NMNAT2 is distributed throughout the healthy axon by a delivery system called axonal transport [4] (Figure 3A). When the levels of NMNAT2 decrease due to an axon being injured or diseased, the axon struggles because of the resulting drop in NAD and energy (Figure 3B).
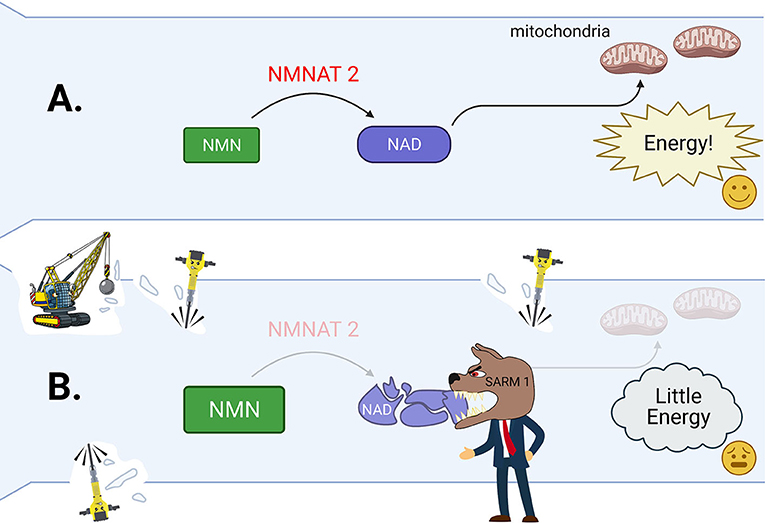
- Figure 3 - (A) In a healthy axon, NMNAT2 helps to create plenty of NAD from NMN.
- NAD helps to generate energy. This keeps the axon energized and healthy. (B) In cut or seriously sick axons, NNAT2 levels drop. This also causes the amount of NAD to also drop, and the level of NMN to rise inside the axon. The watchdog molecule SARM1 is activated by these changes. SARM1 then tears apart the remaining NAD, leading to complete energy failure inside the axon. This tells the Wallerian degeneration demolition crew to start their work breaking down the axon (wrecking ball, jackhammers).
But what happens if these problems get out of hand? Strong changes of NMN and NAD trigger the activation of the molecule SARM1 [5, 6]. SARM1 can be seen as a watchdog and the boss of the Wallerian degeneration demolition crew (Figure 3B). It constantly watches over what is happening in the axon by sniffing out the amounts of NAD and NMN. When it is activated, SARM1 destroys all remaining NAD and speeds up the axon’s energy loss. This is the demolition crew’s cue to start working. Calpains are the key tools of the demolition crew to disassemble sick axons. These tools are enzymes that chop up the axon’s scaffold like little jackhammers (Figure 3).
Going back to the similarities between Wallerian and Wallerian-like degeneration: Many neuroscientists now think that the activation of SARM1 and the demolition crew also takes place in diseased axons, similar to what happens in cut axons. They believe that blocking SARM1 can be used to rescue axons in neurodegenerative diseases.
Conclusion
Axons can easily break down like crumbling bridges. The destruction of axons by Wallerian-like degeneration can lead to serious trouble in many neurodegenerative diseases. With our new knowledge about how the demolition crew and their commander can be controlled, we are starting to believe that there are ways we can rescue axons. Maybe one day, readers like you will use your nervous systems to discover new ways to save axons, which could help people with numerous neurodegenerative diseases!
Funding
We are grateful for the support of mentored undergraduate level research at the University at Buffalo through the Experiential Learning Network (ELN) program. The current work in our laboratory is supported by grants from the National Institute of Health (R01NS111024, R01NS123450, and S10OD030488) and the Muscular Dystrophy Association (577844) as well as start-up funding provided through the Empire State Development Corporation for Hunter James Kelly Research Institute grant nos. W753 and U446 and the Hunter’s Hope Foundation. The figures were created using BioRender (https://biorender.com).
Glossary
Neuro-degenerative Diseases: ↑ A large group of diseases caused by the progressive breakdown of the nervous system.
Wallerian Degeneration: ↑ The biological process by which a disconnected nerve stump with its injured axons degenerates.
Mutation: ↑ A change in the structure of a gene that is transmitted to subsequent generations and may lead to the production of a changed protein.
NAD: ↑ Nicotinamide adenine dinucleotide: molecule found in all living cells that is important for providing cells with energy.
Glycolysis and Mitochondrial Respiration: ↑ Central metabolic pathways that use the sugar molecules glucose and pyruvate to produce energy.
Mitochondria: ↑ Mitochondria are specialized structures in our cells key for energy production. Like batteries, they power various functions in the cells.
NMN: ↑ Nicotinamide mononuculeotide: a molecule that is used to generate NAD.
Enzyme: ↑ A class of proteins created by all organisms that are responsible for speeding up biochemical reactions.
NMNAT2: ↑ Nicotinamide mononucleotide adenylytransferase 2: An enzyme in axons that helps to produce NAD.
Conflict of Interest
The authors declare that the research was conducted in the absence of any commercial or financial relationships that could be construed as a potential conflict of interest.
References
[1] ↑ Waller, A. 1850. Experiments on the section of glossopharyngeal and hypoglossal nerves of the frog and observations uf the alternatives produced thereby in the structure of their primitive fibres. Philos. Trans. R. Soc. Lond. B Biol. Sci. 140:423–9.
[2] ↑ Beirowski, B., Adalbert, R., Wagner, D., Grumme, D. S., Addicks, K., Ribchester, R. R., et al. 2005. The progressive nature of Wallerian degeneration in wild-type and slow Wallerian degeneration (WldS) nerves. BMC Neurosci. 6:6. doi: 10.1186/1471-2202-6-6
[3] ↑ Coleman, M. P., and Hoke, A. 2020. Programmed axon degeneration: from mouse to mechanism to medicine. Nat. Rev. Neurosci. (2020) 21:183–96. doi: 10.1038/s41583-020-0269-3
[4] ↑ Sleigh, J. N. 2020. Axonal transport: the delivery system keeping nerve cells alive. Front. Young Minds (2020) 8:2. doi: 10.3389/frym.2020.00012
[5] ↑ Figley, M. D., Gu, W., Nanson, J. D., Shi, Y., Sasaki, Y., Cunnea, K., et al. 2021. SARM1 is a metabolic sensor activated by an increased NMN/NAD(+) ratio to trigger axon degeneration. Neuron. (2021) 109:1118–36.e11. doi: 10.1016/j.neuron.2021.02.009
[6] ↑ Jiang, Y., Liu, T., Lee, C. H., Chang, Q., Yang, J., and Zhang, Z. 2020. The NAD+-mediated self-inhibition mechanism of pro-neurodegenerative Sarm1. Nature (2020) 588:658–63. doi: 10.1038/s41586-020-2862-z