Abstract
How do you feel when you hear the music of an ice cream truck? What about when you hear your alarm clock in the morning? The first might make you feel happy or excited and might even cause you to run toward the truck to get some ice cream. The second might annoy you or make you want to put your pillow over your ears! Why do you think these two sounds can cause such different emotional reactions? How can your brain tell when you should expect something pleasant or unpleasant? In our study, we investigated how emotional learning happens in a brain region called the amygdala. We found that learning about positive and negative events is divided between two different groups of amygdala cells.
How Does the Amygdala Learn?
The amygdala is small, ancient brain region that has long been thought to be important for experiencing and expressing emotions. In humans, this almond-shaped structure is nestled under the surface of the brain close to the temples (near the ears). Scientists think a part of the amygdala called the basolateral amygdala (BLA) is important for emotional learning [1, 2]. But how can the BLA—just one little brain region—do such an important task? To understand how the amygdala helps us learn how to feel about what happens to us, we first have to understand how the brain learns.
Brain cells called neurons form connections called synapses to communicate with one another. Different neurons are sensitive to different things that happen inside and outside of the body. The messages neurons send to each other produce experiences like fear, pleasure, and excitement, and also cause behaviors like startling, social interaction, and reward-seeking. When the brain needs to learn that one thing leads to another, synapses are strengthened in a process called long-term potentiation (LTP). This means that the connections between those specific neurons become stronger, so communication becomes faster and easier.
For example, imagine two neurons in a mouse’s brain that are connected (Figure 1). One of these neurons responds when the mouse hears a particular sound and the other neuron responds when the mouse tastes something sweet. Normally, there is no reason for the mouse to connect these experiences, so communication between the two neurons does not happen much and the connection between them is weak. If we want to train the mouse to expect a sweet treat whenever we ring a bell, he needs to learn the connection between the bell and the treat. If we ring the bell and give the treat at the same time over and over, the two neurons that respond to the sound and the taste will be active at the same time over and over, too. Because they are active at the same time, the connection between them will be strengthened (this is LTP). Once that happens, the mouse will expect to get the treat whenever he hears the bell. This is because the “bell” neuron communicates with the “sweet” neuron through their strong connection.
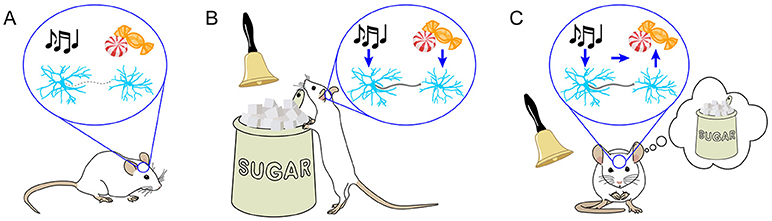
- Figure 1 - Learning strengthens the connections between certain neurons.
- (A) Before learning, the neurons in the mouse’s brain are weakly connected, represented by the gray dashed line between them. The first neuron is sensitive to sound, and the second is sensitive to sweet taste. (B) To train the mouse to expect a sweet reward whenever he hears the sound of a bell, the bell and the reward are repeatedly presented together. This training procedure will cause both neurons to become active at the same time. As a result, the connection between them is strengthened (bold gray line). This process of strengthening is called long-term potentiation. (C) Once the connection between these neurons is strengthened, it is much more likely that activity in the first neuron will lead to activity in the second neuron. This may be why after learning, the sound of the bell leads the mouse to expect the reward, even before he receives it.
The BLA uses LTP to help us form connections between neutral things that happen (like the bell, or the music of the ice cream truck, or the sound of the alarm clock) with things that cause emotions (like the rewarding treat, the ice cream, or waking up before we are ready) [3, 4, 5]. By forming strong connections between these things, the BLA helps us guess what might be about to happen and influences our mood, even before we actually experience the things that make us feel emotions.
Something that has been confusing to neuroscientists is how the BLA can tell the difference between things that will make us feel good and things that will make us feel bad. Scientists have found that the BLA learns about both good things and bad things using LTP. How can it let us know which is which?
It Is in the Connections
One way the BLA might separate positive and negative things is by using different groups of neurons to learn about these two types of things. This way, there would be one group of neurons that would undergo LTP when we learn about “good” things, like the sound of the ice cream truck, and a different group that would undergo LTP when we learn about “bad” things, like the sound of the alarm clock. But what could identify these two groups of neurons? We suspected that we would be able to tell these two groups of neurons apart by which other brain areas they connect to. The BLA communicates with many other brain regions. For example, the BLA sends information to a different part of the amygdala, called the centromedial amygdala (CeM), which is involved in fear behaviors. The BLA also sends information to the nucleus accumbens (NAc), which is involved in learning about and seeking rewards (Figure 2). We wondered whether different groups of BLA neurons connect to these two brain regions. If so, would neurons connected to the CeM learn about negative emotional events, while neurons connected to the NAc learn about positive emotional events?
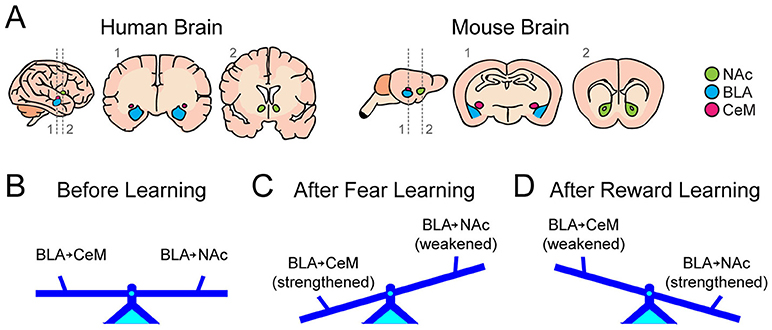
- Figure 2 - Fear and reward learning differently affect BLA neurons connected to the CeM versus the NAc.
- (A) Human brain (left three images) and mouse brain (right three images), with the positions of the BLA, NAc, and CeM shown in blue, green, and purple. For both types of brains, a surface view of the right side is shown first, and then front-facing slices taken along the dashed lines (labeled 1 and 2) are shown. We show these slices because the BLA, CeM, and NAc are located under the surface of the brain. (B) Before learning, the strength of connections between BLA neurons and the CeM and NAc exist in a baseline state, represented by a balanced seesaw. (C) After fear learning, we found evidence of LTP in BLA neurons connected to the CeM, but in BLA neurons connected to the NAc, we found that connections had been weakened. (D) The impact of reward learning was the opposite of the impact of fear learning.
To ask these questions, we performed experiments in mice. It is necessary to remove the brain and study individual neurons to learn about the strength of their connections to other neurons. For this reason, we could not do these experiments in people. The amygdala in mice is very similar to the amygdala in humans, and so are the connections the amygdala makes with other brain regions. We minimized the number of animals used and limited their pain and distress.
How can we know which BLA neurons connect to the NAc and which connect to the CeM? To find out, we used a tool called retrobeads. When we inject retrobeads into an area of the brain, any neurons that connect with that area will take up the retrobeads. Retrobeads are brightly fluorescent, so any cell that contains them will glow under fluorescent light. So, if we inject retrobeads into the NAc and then look at the BLA, we know that the glowing neurons are connected to the NAc.
We injected red retrobeads into the NAc and green retrobeads into the CeM of some mice, and in other mice we switched the colors for the two different brain regions. No matter which color retrobeads we injected into these two areas, when we looked at brain slices from the BLA under a fluorescence microscope, we found that some neurons glowed green, and some neurons glowed red, but very few neurons contained both red and green. This finding means that the BLA neurons connecting to the NAc and the ones connecting to the CeM are mostly two different groups. We were excited about this finding—could these two groups of neurons be the ones responsible for positive and negative emotional learning?
How Does Emotional Learning Change BLA Neurons?
To find out, we trained mice to expect a short electric shock immediately after we played a tone. This procedure is called fear conditioning. Meanwhile, we trained different mice to expect a drop of sugar water after we played the same tone, which is called reward conditioning. All of these mice had previously received injections of red and green retrobeads into the NAc and CeM. After the mice finished learning the positive or negative associations, we removed their brains and made thin slices of the BLA. In these slices, we could examine the strength of synapses between neurons containing retrobeads and the brain regions that are sensitive to tones. We also examined the strength of synapses in mice that did not hear tones when they received a shock or reward. These mice heard the tones when no shock or reward was present, and so could not learn a connection between those events and the sound. These mice did not undergo fear or reward conditioning, so the strength of connections in their brains represents a baseline. By comparing synaptic strength in mice that learned the positive or negative associations to synaptic strength in mice that did not, we could see if changes happened because of the positive or negative learning experience.
What do you think we found? Remember, we expected the BLA neurons connected to the NAc to learn about positive events, and the BLA neurons connected to the CeM to learn about negative events. We observed that our predictions were right, but with an exciting twist! When we examined BLA neurons connected to the CeM after fear learning, we found stronger synapses compared with mice that had not learned an association between tone and shock (Figure 2). This is evidence that these neurons had undergone LTP, consistent with a role in negative emotional learning. When we recorded the strength of synapses in these neurons after reward learning, we were surprised to find that the connections had been weakened!
Meanwhile, when we examined BLA neurons connected to the NAc, we found the opposite changes happened (Figure 2). We saw evidence that LTP occurred in these neurons when the mice experienced reward learning. This is what we expected if this group of cells is responsible for positive emotional learning. But we were surprised when we investigated synaptic strength in these cells after fear learning. In this case, the connections these neurons formed were weaker than those of mice that had not learned.
Based on these findings, it seems that BLA neurons connected to the NAc help us learn positive associations and BLA neurons connected to the CeM help us learn negative associations. But how do these neurons influence living mice? If these two groups of neurons are truly important for positive and negative emotional learning, it stands to reason that directly activating them should influence the emotional state of the mice.
How Do BLA Neurons Affect Emotional State?
We used a technology called optogenetics to ask this question. Optogenetics is a group of important tools that allows neuroscientists to turn neurons on or off using light (see Video). We deliver DNA to the neurons we are interested in, and that DNA enables neurons to make light-sensitive proteins. These proteins can increase the activity of neurons when they are exposed to light.
To get the light into the brain, we implant optic fibers above the areas we want to study. Optic fibers are flexible glass fibers a little thinner than a piece of uncooked spaghetti that can be used to carry light. A small fiber is surgically implanted into the mouse’s brain and cemented in place so that it will not move. We can connect this fiber to a long optic fiber cable, which is positioned in front of a blue laser that we can turn on or off as needed. The outside of the cable is coated so that the blue light passing through it is not visible outside the brain and the mouse cannot see it.
We used optogenetics to study whether our two groups of BLA neurons influence the emotional state of the mice. Because mice cannot tell us how they are feeling, we must figure out their emotions based on their behavior. For example, we can let a mouse explore a large chamber, and only use light to activate neurons when the mouse is on one side of the chamber (see Video; Behavior 1). If activating the neurons makes the mouse feel unpleasant, he will avoid the side of the chamber where we shine light into his brain. In fact, this is what happened when the light-sensitive protein was in the BLA neurons connected to the CeM (Figure 3; see Video). This observation makes us think that activity in these neurons was unpleasant to the mice, fitting with the idea that the BLA neurons connected to the CeM are responsible for negative emotional learning.
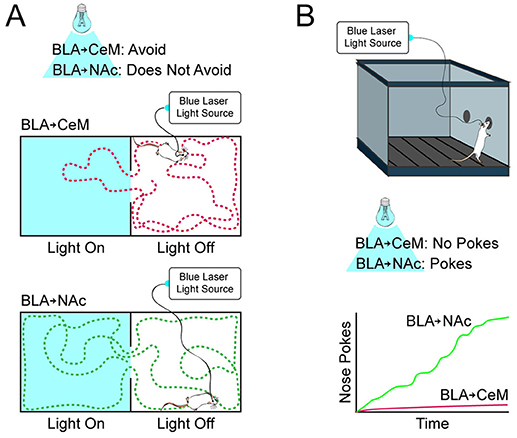
- Figure 3 - Mice like or dislike activity in BLA neurons, depending on where they connect.
- (A) We allowed mice to freely move between two sides of a chamber, where on one side they would receive light stimulation of the BLA. When we increased activity in the BLA neurons connected to the CeM, the mice avoided the side of the chamber where they received light. When we stimulated BLA neurons connected to the NAc, the mice did not avoid the light-paired side. You can see this in the diagrams showing the movement paths of mice receiving stimulation of BLA neurons connected to either the CeM or the NAc. (B) In a separate chamber, the mice could poke their noses into a hole to receive a brief light stimulation of the BLA. The mice receiving stimulation of the BLA neurons connected to the NAc would poke multiple times (as shown on the graph). But, the mice receiving stimulation of the BLA neurons connected to the CeM did not work for this stimulation.
What do you think happened when we turned on the BLA neurons connected to the NAc? The mice did not avoid the part of the chamber where we shined light! In another experiment, we let the mice control whether the light was on or off (see Video; Behavior 2). To make light flash into the BLA and turn on the neurons connected to the NAc, the mice could poke their noses into a hole in the chamber wall. The mice poked many times to activate these neurons, so we can infer that this activity was pleasant to the mice (Figure 3; see Video). This finding makes sense if BLA neurons connected to the NAc are responsible for positive emotional learning. Meanwhile, the mice would not poke to turn on the BLA neurons connected to the CeM, just as we expected.
What Did We Learn, and Why Does It Matter?
What do you think all of these findings mean? We think that it is very likely that the BLA divides fear and reward learning between different groups of neurons, based on which other brain regions they communicate with. But why did we find that certain connections were weakened by different types of emotional learning? It is possible that there is a balance between BLA neurons connected to the NAc and those connected to the CeM, so that, in order for one group to grow stronger, the other group must reduce its activity. For example, if BLA neurons connected to the NAc are like the “gas pedal” for reward learning and BLA neurons connected to the CeM are like the “brake pedal,” we might need both an increase in gas and a release of the brakes for reward learning to happen.
We hope that understanding the building blocks of emotional learning in the amygdala will help us know how this process works in the healthy brain. Emotional learning is disrupted in many psychiatric illnesses like depression, anxiety, and post-traumatic stress disorder (PTSD). If we can understand how emotional learning is supposed to happen normally, we can use this knowledge to figure out what goes wrong in illnesses like these. Research like ours is the first step in finding the best treatments for illnesses that affect many people, and that we are only beginning to understand.
Glossary
Basolateral Amygdala (BLA): ↑ Basolateral amygdala (BLA) sounds like “bay-so-lah-ter-ahl ah-mig-da-lah” → a section of the amygdala that is involved in learning about positive and negative associations; the BLA is connected with many other regions of the brain important for motivation, learning, and decision making.
Neuron: ↑ Neuron sounds like “nur-ahn” → a major category of brain cells; activity and communication among neurons drives thinking, emotion, learning, body movements, and other brain functions.
Synapse: ↑ Synapse sounds like “sin-aps” → the point of communication between neurons, which is actually a very small space across which chemical signals are sent.
Long-Term Potentiation (LTP): ↑ Long-term potentiation (LTP) sounds like “poh-ten-shee-ay-shun” → one important way brain circuits change during learning, in which the strength of connections between neurons is increased.
Centromedial Amygdala (CeM): ↑ Centromedial amygdala (CeM) sounds like “sen-tro-mee-dee-ahl ah-mig-da-lah” → a part of the amygdala that sends signals to areas that control defensive and escape-related behaviors and is important for fear learning.
Nucleus Accumbens (NAc): ↑ Nucleus accumbens (NAc) sounds like “noo- clee-us uh-kum-bins” → a part of the brain important for motivation and reward learning, which sends signals to brain areas that help control purposeful movement.
Retrobeads: ↑ Retrobeads → microscopic, fluorescent latex spheres that are taken up by neurons and transported to the cell body; used to label neurons based on where they make connections.
Optogenetics: ↑ Optogenetics sounds like “ahp-toe-jen-eh-tiks” → a group of tools in neuroscience enabling the control of neural activity through light-sensitive proteins.
Video: ↑ Using optogenetics as a tool to understand how neural activity impacts behavior. Available online at: https://www.youtube.com/embed/H6n6xS1qhlk
Conflict of Interest Statement
The authors declare that the research was conducted in the absence of any commercial or financial relationships that could be construed as a potential conflict of interest.
Original Source Article
↑ Namburi, P., Beyeler, A., Yorozu, S., Calhoon, G. G., Halbert, S. A., Wichmann, R., et al. 2015. A circuit mechanism for differentiating positive and negative associations. Nature 520:675–8. doi: 10.1038/nature14366
References
[1] ↑ Janak, P. H., and Tye, K. M. 2015. From circuits to behaviour in the amygdala. Nature 517:284–92. doi: 10.1038/nature14188
[2] ↑ Bocchio, M., Nabavi, S., and Capogna, M. 2017. Synaptic plasticity, engrams, and network oscillations in amygdala circuits for storage and retrieval of emotional memories. Neuron 94:731–43. doi: 10.1016/j.neuron.2017.03.022
[3] ↑ Rogan, M. T., Stäubli, U. V., and LeDoux, J. E. 1997. Fear conditioning induces associative long-term potentiation in the amygdala. Nature 390:604–7.
[4] ↑ McKernan, M. G., and Shinnick-Gallagher, P. 1997. Fear conditioning induces a lasting potentiation of synaptic currents in vitro. Nature 390:607–11.
[5] ↑ Tye, K. M., Stuber, G. D., de Ridder, B., Bonci, A., and Janak, P. H. 2008. Rapid strengthening of thalamo-amygdala synapses mediates cue-reward learning. Nature 453:1253–7. doi: 10.1038/nature06963