Abstract
Over 100 years ago, Nobel Prize winner Ramon y Cajal first described structures called dendritic spines, located on the surface of brain cells. Since then, scientists have been trying to understand what dendritic spines are and how they function in the brain. Even with the latest technology, it is very difficult to research dendritic spines because they are so tiny—one dendritic spine is about 1/1,000 of a millimeter, and there are thousands of them on the surface of most nerve cells in the brain. Since dendritic spines can change shape and size quickly, some researchers have suggested that they are the structures in the brain where memories are created and stored. This hypothesis is based on hundreds of studies showing that forming a memory causes dendritic spines to undergo major changes in number, size, and shape. Likewise, preventing dendritic spines from changing may stop memories from forming. However, there is still a long way to go before we can identify exactly where memories are kept in the brain. Future studies may help us answer this fascinating riddle.
About 130 years ago, powerful microscopes were developed that allowed researchers to see brain cells called neurons in more detail. At that time, the Spanish researcher Ramon y Cajal discovered that the membrane, or outer covering, of brain neurons was not as smooth as scientists previously thought it was. He discovered that brain neurons have thousands of tiny outgrowths, each about 1/1,000 of a millimeter long, which are now called dendritic spines. For this work, Ramon y Cajal won the Nobel Prize for Physiology or Medicine in 1906.
Since then, we have learned a huge amount about dendritic spines. Most of what we know supports the idea that these spines are one of the main places in the brain where memories are created and stored [1]. This article will explain, support, and expand on this hypothesis.
What Are Dendritic Spines?
Let us begin by describing the parts of the dendritic spine. A dendritic spine looks like a mushroom (Figure 1), with a large head and a thin neck. The head is the place where two neurons connect and exchange information, an area called the synapse. The head contains all the components the neuron needs to receive information from other cells, including molecules on its surface called receptors, which can bind to substances called neurotransmitters that carry signals between neurons; and substances that control the number of receptors and their activity.
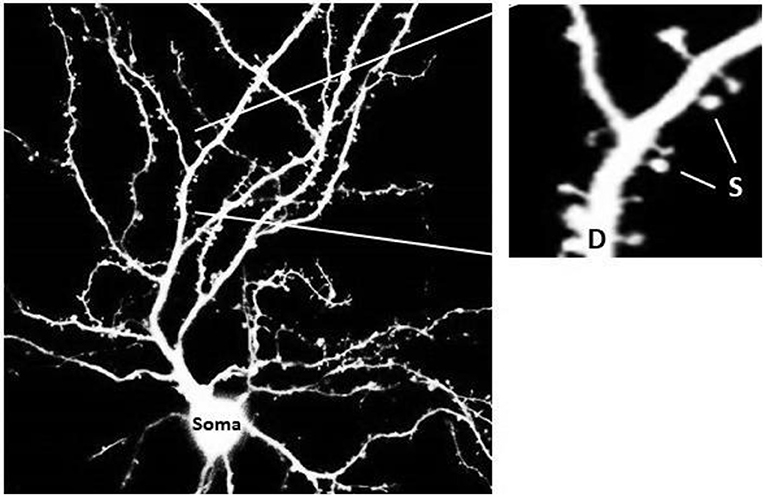
- Figure 1 - The structure of a neuron.
- The cell body (soma, bottom) is about 1/40 of a millimeter in size. The cell body sends out long extensions called dendrites (D, in the right figure, which is a magnified image of part of the cell on the left), along which there are dendritic spines of various sizes (s, marked by an arrow, about 1/1,000 of a millimeter.
The neck of the dendritic spine can be very thin—about 1/10 the thickness of the head or approximately 1/2000 of a millimeter. One of the neck’s functions is to maintain the relationship between the neuron and other cells in the neural network, by regulating the movement of substances in and out of the head. As a result of this regulation, the neuron changes the way it responds to stimuli.
According to one estimate, there are between 300–400 different types of substances that go in and out of dendritic spines through their necks, some in a very short amount of time. The movement of some of these substances can be controlled with molecular tools or with certain drugs. Controlling the passage of these substances may change the size and shape of the dendritic spine. It is also possible to control the dendritic spine’s ability to send and receive electrical signals, which are one way that neurons communicate, but due the tiny size of dendritic spines, the methods we have today do not allow us to measure their electrical activities directly or reliably. This measurement problem makes it difficult for scientists to determine the neck’s role in transmitting messages from the dendritic spine’s head into the neuron.
Calcium is one important substance found in small amounts in dendritic spines. In addition to the many roles of calcium in the body, and especially in neurons, it is also responsible for activating enzymes (biological molecules that make reactions happen more easily) that initiate the transfer of messages from one neuron to another. There are sophisticated laboratory tools that allow us to precisely measure the amount of calcium in neurons and in their dendritic spines. These measurements can help us learn about how the head and the neck of the spine communicate, and can give us a fairly accurate picture of how dendritic spines change their shape and function.
Role of Dendritic Spines in Learning and Memory
The idea that dendritic spines are associated with learning and memory has been tested in a series of experiments that tracked changes in the structure of dendritic spines in the brains of laboratory rats and in brain slices. In these experiments, researchers looked at the spines before and after exposure to electrical stimulation. The electrical stimulation caused a phenomenon called long-term potentiation (LTP), which is expressed as an increase in responses of the neuron to electrical stimulation, and is generated along the dendritic tree (see Figure 1). Over the past 50 years, LTP has been studied in thousands of experiments looking at the whole brain, brain slices, and brain cells grown in the lab. In a nutshell, these experiments showed that the brain uses an LTP-like mechanism to generate memory, and if this mechanism is disabled, the memory disappears or cannot be acquired. Our findings of LTP in dendritic spines following electrical stimulation thus tell us that spines assume an important role in synaptic plasticity. Likewise, a reduction in synaptic activity may lead to a process of Long-Term Depression (LTD), whereby dendritic spines that are not activated may shrink and lose their synaptic partner (e.g. Figure 2, bottom line).
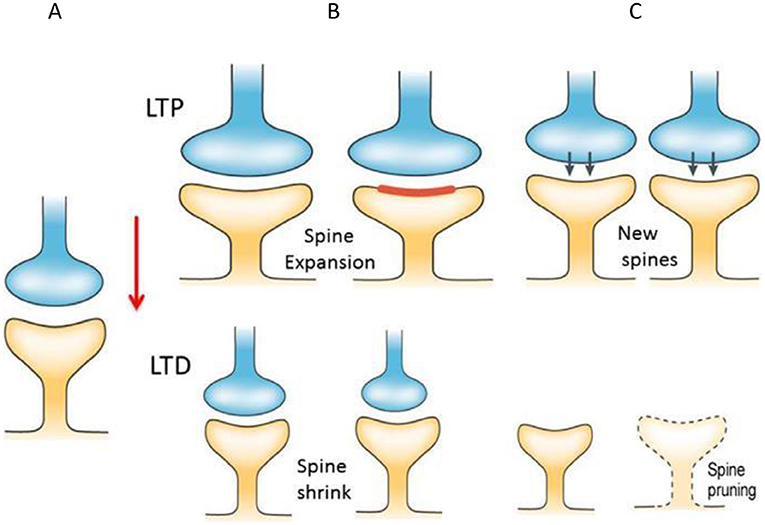
- Figure 2 - Dendritic spines change following electrical stimulation.
- (A) Before electrical stimulation applied to the presynaptic neuron (blue) is applied, dendritic spines exist in a calm and balanced state. (B) Following electrical stimulation to the blue neuron, a phenomenon called long-term potentiation (LTP) occurs in the dendritic spines, causing the spine head to initially expand, increase the size of the spine membrane (red) and leading to the creation of new spines. In an opposite process, where the presynaptic cell ceases to act, a process called Long Term Depression (LTD) takes place, leading to elimination of the inactive spines in a process called “pruning.” (C) Bottom right.
When researchers looked at a single dendritic spine as it was exposed to electrical stimulation, they discovered that, within a fraction of a second, there is a massive flood of calcium ions into the spine. As a result, the head of the spine can swell up to 3–4 times its original size. Immediately after, there is a flow of neurotransmitters to receptors in the head of the spine, which increases the spine’s response to the electrical stimulation (Figure 2). This change, at a later stage, in a process that lasts from minutes to hours, results in formation of new dendritic spines. These new spines cause a stronger connection between cells. In other words, in the brain, learning is based both on a change in the response strength of dendritic spines and on the creation of new spines. In this way, the connection between the cells that make up the memory in the neural network is tightened.
Dendritic Spines in Aging and Brain Diseases
Another path to understanding the relationship between learning and changes in dendritic spines is to look at how the spines change in an adult animal as it ages. As we know, as adult humans get older, memory gradually decreases. This process is not the same for everyone: some elderly people experience a sharp decline in memory, while others have only a moderate decline. This decrease in brain functions, including memory, is known as cognitive decline. A similar phenomenon also occurs in lab animals. Studies have identified a correlation between cognitive decline and the number of dendritic spines in the brains of lab rats. That is, as rats’ memory loss increases, the number of spines in their neurons also decreases. This does not mean that the decrease in the number of spines causes cognitive decline, but only that there is a positive correlation between the two observations. Further studies are required to confirm and fully understand these findings.
Another area in which there has been interesting progress in the study of dendritic spines is in the case of diseases of the nervous system. In one of the first studies on this topic, researchers examined neurons in the brains of patients with fragile X syndrome [2], a disease that causes varying degrees of mental disability, autism, and communication disorders (among other things). It was found that the dendritic spines do not fully develop in the brains of people with this syndrome.
While these studies focus on understanding the relationship between brain disabilities and the structure of dendritic spines, they cannot explain the process that causes the disabilities. To fully understand the relationship between faulty dendritic spines and problems of brain function, we should be able to fix the problem, which we do not yet have [3].
Summary and Conclusions
Understanding the structure and function of dendritic spines in the brain is very important, but also very challenging. First, the tiny size of dendritic spines makes them difficult to access, and in each neuron there are tens of thousands of such spines. Second, there are many kinds of dendritic spines, and it is not yet clear what the role of each type is. Beyond that, there is the big, unanswered question about the identity of the memory “unit” in the brain and how it works: Are memories held in a single dendritic spine, a single cell, or a group of cells connected to each other in a neural network? Researchers will only be able to answer this if they can locate a certain memory (say, of the letter A) in the brain; by damaging the dendritic spine/cell/neural network to see if only that specific memory is lost—not any other memory or function. If we can understand how the structure and function of an individual dendritic spine relates to the functioning of the entire brain, perhaps we will also be able to repair particular spines to restore memory and treat memory-related diseases such as Alzheimer’s disease. Future generations of neuroscientists will face these challenges—maybe you will be one of them?
Glossary
Neuron: ↑ The elementary unit of the brain. It contains the receiving end (spines and dendrites), the transmitting end (axon) and the central part, the soma, which controls the activity of the whole unit.
Dendritic Spine: ↑ A small structure opposite a presynaptic terminal (end) which receive input from the presynaptic cell, and controls the flow of information between the two cells.
Synapse: ↑ The connection between two neurons, where messages are transferred between the cells.
Neurotransmitter: ↑ A chemical substance that passes through the synapse between neurons and transmits signals between them.
Neuronal Network: ↑ A series of neurons, connected in a network that receives information from other networks, modifies the information, and passes it on to other networks. A network can consist of dozens to millions neurons, who share information in a particular region of the brain.
Enzyme: ↑ A protein produced by living cells that helps to start a chemical reaction or process.
Long-term Potentiation (LTP): ↑ Long-term amplification of an electrical signal along a brain neuron, which strengthens the connection between neighboring neurons.
Long-term Depression (LTD): ↑ A condition opposite to LTP, whereby the connection between two neurons is weakened, leading to loss of connectivity and eventual loss of memory.
Conflict of Interest
The author declares that the research was conducted in the absence of any commercial or financial relationships that could be construed as a potential conflict of interest.
References
[1] ↑ Sala, C., and Segal M. 2014. Dendritic spines: the locus of structural and functional plasticity. Physiol. Rev. 94:141–88. doi: 10.1152/physrev.00012.2013
[2] ↑ Telias, M. Yanovsky L., Segal, M., and Ben-Yosef, D. 2015. Functional deficiencies in fragile X neurons derived from human embryonic stem cells. J. Neurosci. 35:15295–306. doi: 10.1523/JNEUROSCI.0317-15.2015
[3] ↑ Bock, J., Weinstock T., Braun, K., and Segal M. 2015. Stress in utero: Prenatal programming of brain plasticity and cognition. Biol. Psychiatry. 78:315–26. doi: 10.1016/j.biopsych.2015.02.036