Abstract
Animal migration is the seasonal movement from one region to another and can involve thousands of individuals journeying across continents and oceans. Scientists often use electronic and physical tags to track the movements of migratory fish. However, these tags only work on bigger fish and only give a limited picture of where the fish have been. However, scientists discovered how to use fish otoliths (“ear stones”) to reveal the secret journey of migrating fish over their entire lifetime. Otoliths grow a new layer every day of the fish’s life, producing growth bands just like a tree. The otoliths incorporate chemical elements from the water as the fish grow and move, providing a chemical map of where the fish have been. The growth bands also tell us how long the fish spent in each habitat and how fast they grew. This information helps conservationists understand which habitats are most important and how to protect endangered species.
In this paper, we are going to take you on a journey into the wonderful world of fish migration and introduce you to a tiny ear stone that eavesdrops on the lives of fish, recording their every movement and so much more…
Fish Migration—What Is It and Why Do We Care?
We often hear about the seasonal migrations of birds flying from one hemisphere to the other to avoid the long, cold winters, or the great wildebeest migrations crossing the African savannah. However, fish also perform amazing migrations, but they are below the surface of the water and harder to see [1]. Like birds, fish can move in all directions, and in the ocean, many fish carry out vertical “migrations” each day, feeding at the surface at night and then hiding in the murky depths during the day, to avoid being seen by hungry predators. Fish can also swim long distances, with the mighty tuna migrating thousands of kilometers to the same breeding grounds each year [1]. Even flounder can migrate hundreds of kilometers each year by surfing the ocean currents, and they cover vast distances without the big muscles found in tuna. The sneaky flounder bury themselves in the sand when the currents change direction, to make sure they do not slip backward. There are also fish like salmon or eel that migrate through fresh and saltwater to reach their final destinations. Many of you may have eaten salmon, but did you know that they can migrate more than 3,000 km up rivers to return to the place where they were born? That is further than the distance from Mexico to Canada!
But why should we care about fish migration? Apart from being a fascinating event often involving thousands of animals, it also affects how we manage wild populations and conserve endangered species. Humans around the globe love eating fish. However, in order to avoid catching too many, we need to first understand where the fish are located across their entire lifetime. This is particularly challenging for migratory fish, but it allows fishery managers to make rules, if necessary, to reduce fishing in a certain habitat or habitats, such as those where the fish gather to reproduce and grow. Today, many fish are not able to successfully migrate to the habitats that they need to live and reproduce because humans have blocked their path with dams and other structures.
Why and How Do We Tag Animals?
Humans have always been curious to know where animals go. For example, many people put bells on their dogs or cats to locate them. This practice was already common thousands of years ago in ancient Egypt. Shepherds do the same with cattle. So, a simple bell can act as a tag that allows a person to know where an animal is located. However, this tag only allows tracking animals that are within our hearing or sight range, and the animal must be large enough to wear a collar.
Today, technological advances have moved us well beyond bells and have allowed us to track the long-distance migrations of a variety of species [1]. Electronic tags vary from simple light sensors that track bird migrations from the North pole to the South pole, to complex global positioning system (GPS) tags that record an animal’s position at any given moment, anywhere on the planet. GPS collar tags are used on many terrestrial (land) species, including lions, gorillas, elephants, zebras, bears, and pandas. Other types of GPS tags are attached like body piercings to the fins or backs of large aquatic animals, such as turtles, whales, tuna, or sharks [1]. In Figure 1, you can see the migratory route made by a shortfin mako shark in the northwestern Atlantic Ocean, as it was tracked by a GPS tag. Usually, GPS tags are big and heavy, so they cannot be used on smaller, weaker animals [1]. For this reason, most bird species are tagged with small, light, metal rings or colored plastic bands with unique codes. These tags are much lighter than GPS tags, but are less technologically advanced and only give us the position of a tagged bird when the bird’s leg or wing is observed. But, what about fish, which are typically too small for GPS tags and are swimming underwater where we cannot see or hear them?
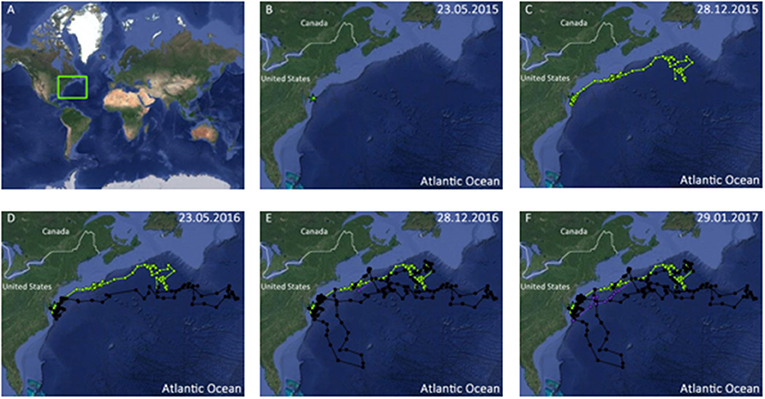
- Figure 1 - (A) Migration path of a shortfin mako shark in the northwest Atlantic Ocean, which is the region highlighted by the green box.
- (B) This shark was tagged with a GPS device off the coast of Maryland (USA) on May 23, 2015. The GPS tag transmitted the shark’s movements for 626 days During that period, this shark swam 21,974 km. (B, C) The green line represents the movements of this shark during 2015. (D, E) The black lines represent the movements during 2016. (F) The purple lines represent the movements made during 2017. It is interesting to see that this shark migrated back to the Maryland coast 1 year after being tagged there. You can track the migration of other fish at http://cnso.nova.edu/sharktracking. The images used in this figure were retrieved from this website and made available by the Guy Harvey Research Institute, Nova Southeastern University (USA).
Small fish can be tagged with various devices, too. They can be tagged with metal or plastic tags marked with a unique code, or with miniature electronic tags inserted into the abdomen or head [1]. Lucky for us, fish with a skeleton made of bones, like sardines, flounders, or salmon, are born with a natural tag that allows scientists to study their movements from birth to death, without needing to physically tag the fish [1]. This natural tag is a special bone, called an otolith, that records the fish’s daily, seasonal, and annual migrations, as well as many other aspects of their lives [1]. Fish with a skeleton made of cartilage, or cartilaginous fish, do not have otoliths, like sharks, or rays.
What are Fish Otoliths?
Otoliths, or ear stones, grow inside the inner ear of bony fish—that is over 28,000 species, including such diverse species as clownfish, seahorse, salmon, and tuna. Bony fish have three pairs of otoliths—the sagittae, the lapilli, and the asteriscii (Figure 2). Otoliths are made of calcium carbonate, the same chemical compound that chalk and limestone are made of. Different fish have otoliths that are different in shape and size, so scientists can find the otoliths in the poop of birds and seals to see what kind of fish these animals are eating-yuck! Otoliths allow the fish to keep their balance and to detect sound and water depth. Otoliths grow continuously during a fish’s life, a few microns each day from birth until death [2]. One micron is 1,000 times smaller than one millimeter, so otoliths are typically about the size of a grain of sand in juvenile fish and the size of a fingernail in most adult fish.
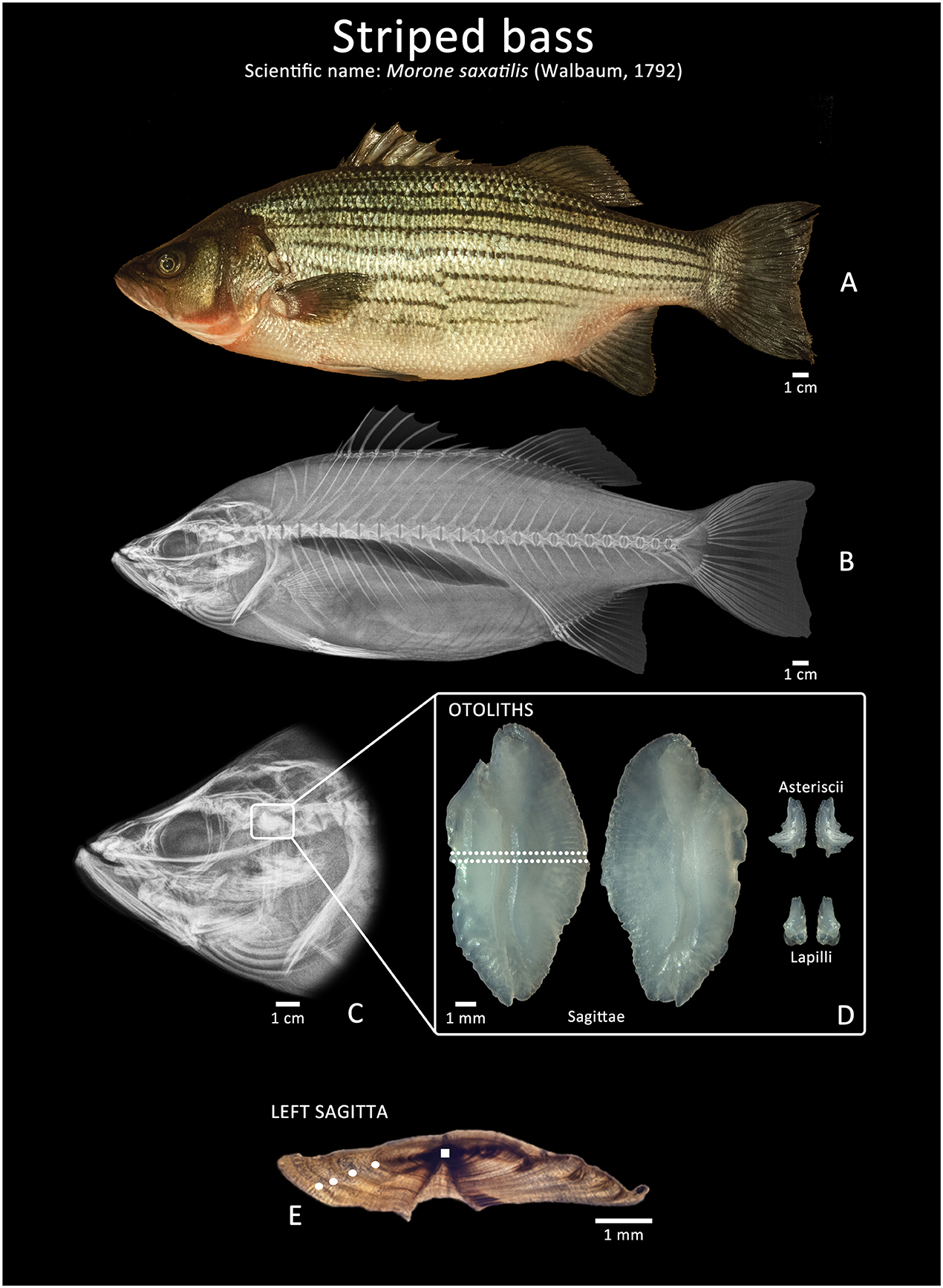
- Figure 2 - (A) Photograph of a striped bass.
- (B) X-ray of a striped bass. (C) Detail of the head in the x-ray, showing the location of the otoliths. (D) Fish have three pairs of otoliths, called the sagittae, the asteriscii, and the lapilli. (E) This photo is a slice of the left sagitta otolith, location shown by the white dashed lines in (D). The white square shows the core of the otolith, which was formed when this fish was a larva. Each white dot shows a thin and dark growth band formed during winter, so this fish was 4 years old. The x-ray was done at School of Veterinary Medicine (University of California, Davis) by the Large Animal Clinic staff under the supervision of Dr. Kathryn L. Phillips.
What Information Is Recorded in Otoliths?
Otoliths are known among scientists as the fish version of a flight box recorder that continuously records an airplane’s flight path. Like a flight recorder, otoliths log the timing and duration of critical events in a fish’s life. Scientists can use microscopes and sophisticated technology to decode visual and chemical messages printed in otoliths, such as a fish’s age and where it lived at a different point in its life [3].
Using Otolith Rings to Estimate Fish Age and Growth
We can figure out the age of a fish by counting the growth rings on its otoliths, exactly the same way we can tell the age of trees [2]. The way this works is that fish have a quieter sleepy period and an awake feeding period every 24 h, just like people. In fish otoliths, this results in light and dark bands forming each day, which you can see when you look at the otoliths under the microscope. The width of the daily bands tells scientists if the fish were growing quickly or slowly—an indicator of whether food was easy or hard to find. As fish get older, scientists can look at larger, seasonal bands to count the number of years that the fish has lived (Figure 2) [2]. One of the oldest fish in the world was a 205-year-old rougheye rockfish, and we know that because of the annual rings in its otoliths! That is older than your great-great-great-grandparents!
Tracking Fish Migrations Using Otoliths
Scientists are now using cutting-edge technology to decipher the chemical code recorded in the otoliths, to reconstruct the secret lives of fish during their migrations. This chemically-written story helps scientists to estimate the amount of time a fish spent in different habitats throughout its life. This is possible because the fish incorporates some of the chemical elements from the surrounding water and from its food into the otolith, and these chemicals can be unique to the habitats in which the fish lived. It is a bit like a chemical fingerprint being recorded in the otolith growth bands that changes when the fish migrates into a new habitat. The technology of reading the chemicals in otoliths works best when the fish moves through very different water environments, such as between rivers, an estuary (the final stretch of a river before meeting the ocean and their length usually varies between a few kilometers up to almost 100 km), and the ocean (Figure 3). But, in certain parts of the world, it is even possible to identify the exact river in which the fish was born, and then to track its movements through complex river networks [4].
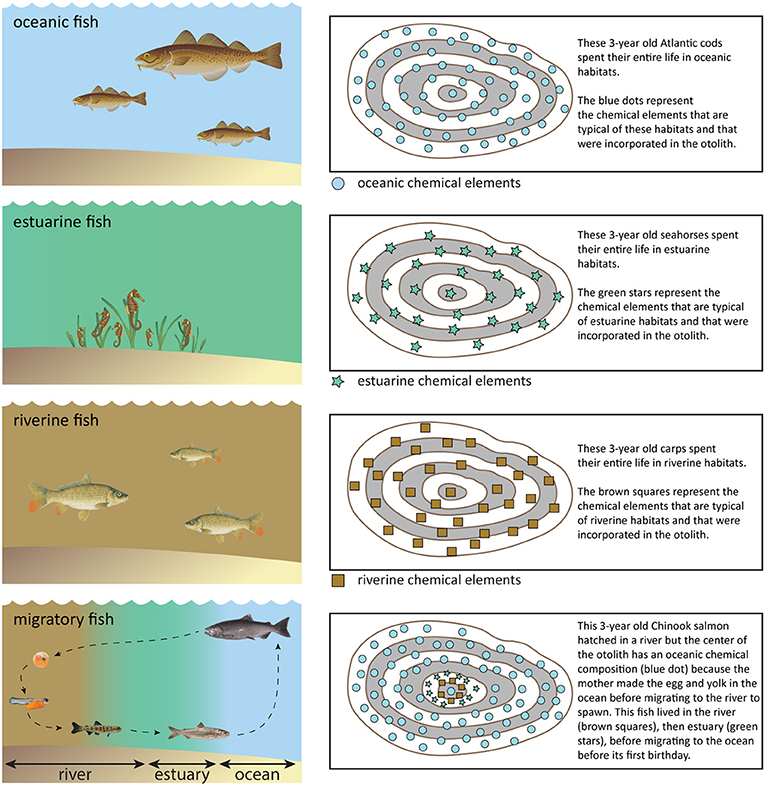
- Figure 3 - The chemical composition of the oceans, estuaries, and rivers is very different.
- Therefore, the chemical elements that are incorporated into the otoliths of fish also vary between ecosystems. So, these chemical elements can be used to determine the ecosystems used by migratory fish across their entire lifetimes (see the bottom panel), and know how much time the fish spent in each one of those ecosystems.
By combining growth rings and chemical analyses, it is possible to estimate how long a fish has spent in each habitat and to compare growth rates in each habitat. Understanding which habitats are important to the fish is necessary information for scientists who are trying to protect or restore these habitats [1].
Information in Otoliths May Help Us Save Endangered Fish
We are actively using the methods described in the previous section to try to save a highly endangered fish. You may have already heard of Chinook salmon (or King salmon). It is the largest salmon species in the world and lives along the entire northern Pacific coast and rivers, from northern Japan to central California in the United States. Like all salmon, Chinook salmon are born in a river, migrate to the ocean as juveniles, then return to the river where they were born to spawn and create the next generation of salmon. Chinook salmon spawn only once and then die. When they die, scientists collect their otoliths and retrace their migration pathways and growth rates over their entire lives [4].
There are four runs of Chinook salmon in the Sacramento-San Joaquin river system, fall-run, late fall-run, winter-run, and spring-run. The name of the runs indicates the season when adults enter freshwater to spawn. Winter-run Chinook salmon are the focus of our story, because there is just one tiny population left in California (USA), leaving them highly endangered [5]. New information obtained from their otoliths may help to protect these salmon. Historically, winter-run salmon spawned in several cold rivers in California, but humans blocked their pathway with huge dams. Today, the only place left for them to spawn is right below a dam, where river temperatures can get very hot. During the drought of 2013–2015, many eggs and juveniles died because of the hot water. However, a small number of winter-run Chinook salmon somehow survived to return as adults in 2016–2018. Scientists are excited to use otoliths to learn the stories of these hardy survivors. They think the otoliths will reveal which habitats might help winter-run Chinook salmon survive during future droughts. Otoliths already revealed that, in other years, young winter-run Chinook salmon often sneak off to smaller rivers to grow and hide [5]. Scientists had no idea how important these secret stop-offs were and think they may provide the salmon with nutritious food and refuge from predators on their way to the ocean. Are these the same habitats the winter-run Chinook salmon use during droughts? This new information will allow scientists to consider which habitats to protect in order to preserve this unique fish.
What Can You Do to Protect Migratory Fish?
Join forces with your friends and teachers to find out which migratory fish species exist in the region where you live, either in rivers or the ocean. Find out which species are endangered and need your help, and try to only buy sustainable seafood (species that were harvested or farmed without destroying natural habitats, endangering other species, and polluting ecosystems—check out Seafood Watch www.seafoodwatch.org and their app). Inform your family, friends, and community that they can help to protect these species. For example, bringing awareness of the many issues migratory fish face is the topic of the annual World Fish Migration Day (worldfishmigrationday.com). This day is celebrated every 2 years across the globe. Maybe you, your friends, and your teachers can organize an event for this day! Look for opportunities to help with beach and river trash clean-up events to provide clean homes for migratory fish and other aquatic species. Also, you can use smart water conservation tips in your homes and yards to leave more water for the fish to enjoy and to help keep the rivers healthy (https://www.smartwater.org.nz/tips). Alert people that removing some barriers along rivers can help increase the number of migratory fish, by allowing them to return to the healthy habitats where they once lived and thrived.
Glossary
Habitat: ↑ Habitats are natural areas or environments used by living organisms to sustain all their primary needs, including to feed and reproduce.
Global Positioning System or GPS: ↑ An array of satellites orbiting the Earth provide us with GPS, allowing electronic receivers on Earth to provide precise locations.
Cartilaginous Fish: ↑ Fish with skeletons made of cartilage, which is softer than bone. Sharks and rays are perfect examples.
Otoliths: ↑ Fish otoliths, or ear stones, are structures made of calcium carbonate located in the inner ear of bony fish. Otoliths help fish to detect sound and avoid predators, and to stay balanced.
Bony Fish: ↑ Fish with skeletons made of bone. Salmon, seahorses, clownfish, perch, and tuna are just a few of the many bony fish that exist.
Chemical Elements: ↑ Chemical elements are the building blocks of everything in the universe from living organisms to non-living things. For example, water is made of two chemical elements, hydrogen and oxygen.
Estuary: ↑ The final stretch of a river before it meets the ocean. The water in the estuary has variable amounts of salt in it and often moves with the tides.
Winter-Run Salmon: ↑ Salmon that return to a river (or “run” from the ocean) in the winter.
Migratory Fish: ↑ Fish that need to move between different habitats during specific phases of their life cycle to reproduce, feed, grow, and/or find refuge from predators or harmful environments.
Conflict of Interest Statement
The authors declare that the research was conducted in the absence of any commercial or financial relationships that could be construed as a potential conflict of interest.
References
[1] ↑ Morais, P., and Daverat, F. 2016. An Introduction to Fish Migration. Boca Raton, FL: CRC Press.
[2] ↑ Panfili, J., De Pontual, H., Troadec, H., and Wright, P. J. 2002. Manual of Fish Sclerochronology. Brest: Institut Français de Recherche pour l’Exploitation de la Mer.
[3] ↑ Campana, S. E. 1999. Chemistry and composition of fish otoliths - pathways, mechanisms and applications. Mar. Ecol. Progr. Ser. 188:263–97. doi: 10.3354/meps188263
[4] ↑ Sturrock, A. M., Wikert, J. D., Heyne, T., Mesick, C., Hubbard, A. E., Hinkelman, T. M., et al. 2015. Reconstructing the migratory behavior and long-term survivorship of juvenile Chinook salmon under contrasting hydrologic regimes. PLoS ONE 10:e0122380. doi: 10.1371/journal.pone.0122380
[5] ↑ Phillis, C. C., Sturrock, A. M., Johnson, R. C., and Weber, P. K. 2018. Endangered winter-run Chinook salmon rely on diverse rearing habitats in a highly altered landscape. Biol. Conserv. 217:358–62. doi: 10.1016/j.biocon.2017.10.023