Abstract
The Southern Ocean, the ocean encircling Antarctica, has been described by explorers as cold, empty, and dangerous. Despite this, it is a paradise for tiny algae called diatoms that are crucial players in the regulation of our climate. Why are these tiny organisms so happy in this cold and far away ocean? Diatoms have a solid shell made of a glass-like material called silica, so they need to find silicon in surface waters to build it. The Southern Ocean is the perfect place for diatoms because it is full of silicon compared to the other oceans. This is due to a special phenomenon called the silicon pump, which makes the Southern Ocean a giant trap for silicon. In this article, we point out the central role of the Southern Ocean in the regulation of Earth’s climate and how it controls the distribution of silicon and the wellbeing of diatoms in Antarctic waters.
Antarctica and the Ocean Conveyor Belt
Seventy percent of the surface of our beautiful blue planet is covered by oceans. It is therefore not surprising that the oceans play a key role in the fragile balance of Earth’s climate. For example, water at the surface of the ocean absorbs heat from the sun and carries this heat around the planet using ocean currents. These currents also transport nutrients that are important for life.
The planet’s strongest current is called the Antarctic Circumpolar Current (ACC) and it is so wide that, by itself, it makes up an entire ocean named the Southern Ocean. The ACC flows eastward in the southern hemisphere, circling Antarctica, and connects the Atlantic, Pacific, and Indian oceans together (Figure 1A). The Southern Ocean plays a key role in Earth’s climate by absorbing a fraction of the carbon dioxide (CO2) produced by human activities, and therefore helps to balance the accumulation of this gas in the atmosphere [1].
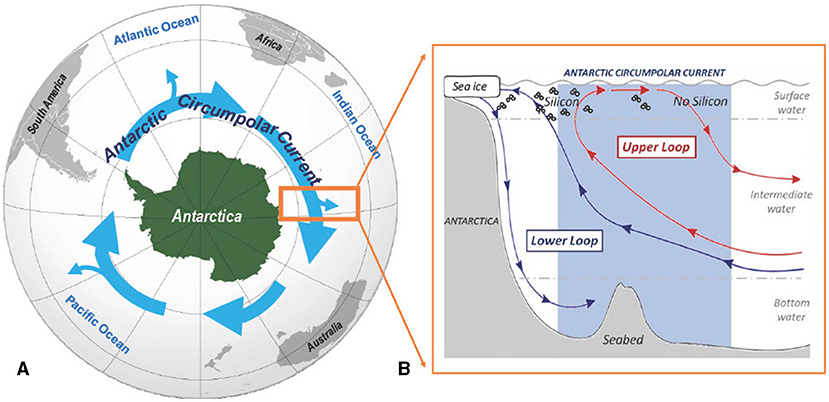
- Figure 1 - (A) The strong Antarctic circumpolar current flows clockwise (eastward) around Antarctica.
- Some water escapes northward into the Atlantic, Pacific, and Indian oceans. (B) Water from the deep ocean is brought to the surface in the Southern Ocean, and it brings lots of silicon with it. As the current flows northward, the silicon is used by diatoms, which leave no silicon in the water that escape the Southern Ocean through the upper loop of the ocean conveyor belt (red). In blue is the water that encounters sea ice dives to the bottom of the ocean (Figure credit for A: AntarcticGlaciers.org).
The ACC is considered to be the core of a much larger system of currents that flow all around the planet; a phenomenon called the overturning circulation, or the ocean conveyor belt. Just like a pulsing heart, the Southern Ocean collects water from the deep layers of the Atlantic, Pacific, and Indian oceans and brings it to the surface. This seawater interacts with the atmosphere and with sea ice and feeds many living organisms. Then the water circulates in two ways, forming two different loops (Figure 1B). In the lower loop, seawater moves southward toward Antarctica. It cools down when meeting sea ice and sinks to the very bottom of every ocean (see also this Frontiers for Young Minds article). In the upper loop, seawater moves northward and eventually dives to intermediate depths (around 1,000 m). These intermediate waters are then transported very far north. They even cross the equator and eventually reach the surface in warmer regions of the ocean [2]. The ocean conveyor belt is very important because it connects the waters at the surface to the intermediate and bottom layers of the ocean, and exchanges heat and nutrients between the Southern Ocean and the rest of the globe.
As you will learn, a small change in the unique environment of the Southern Ocean could have strong consequences on the ability of the ocean to both distribute nutrients throughout the oceans and to regulate the climate.
Why is Antarctica a Diatom Paradise?
Tiny algae live in the surface water of the ocean. There, they absorb nutrients and combine them with sunlight to produce energy and to grow. This process is called photosynthesis, and it is very important for us because photosynthesis uses up CO2 and releases oxygen, making life possible on Earth (for more information on photosynthesis in the ocean, see this Frontiers for Young Minds article). While there are many different algae in the world’s waters, one specific group, called diatoms, thrives in the Southern Ocean’s cold waters (Figure 2). There, diatoms can make up more than 90% of the total amount of algae in the surface ocean [3].
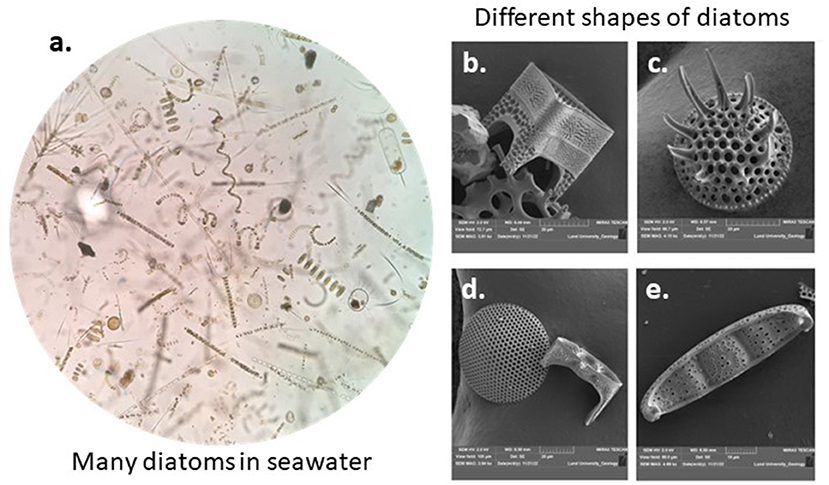
- Figure 2 - (a) There are many diatoms in one drop of Antarctic seawater, but they can be seen only using a microscope.
- (b–e) With a very powerful microscope, the delicate features of the frustule, such as holes and spines, can be observed for each individual diatom species.
Diatoms measure <1 mm in size and are encased in a hard shell, called a frustule, made of silica (the scientific word for glass). Frustules are like beautifully decorated tiny glass boxes and make diatoms unique among all the algae. Billions of diatoms grow during spring and summer at the surface of the ocean, and as they do they absorb CO2. Thanks to their frustule, they are quite heavy (compared to other algae) and they sink to the bottom of the ocean, carrying with them all the carbon they took up in the surface waters. This process is called the biological pump of carbon because the carbon is “pumped” from the atmosphere and stored at the bottom of the ocean for a very long time [3].
Since they need silicon to make their glassy frustule, diatoms depend strongly on the availability of silicon within their environment. Diatoms are so voracious that they can use all the silicon in the surface waters, leaving very little behind. When they have exhausted all the silicon around them, diatoms die and sink toward the bottom of the ocean, carrying all the silicon and carbon with them. Without silicon in the seawater, diatoms cannot grow. Thankfully for diatoms, the Southern Ocean is a paradise because it is the ocean region where silicon is the most abundant. But why is that?
How Does the Southern Ocean Trap Silicon?
Diatom frustules are heavy, making diatoms sink faster than other tiny algae, although it can take them several days to several months to reach the bottom of the ocean. While they are sinking down through the ocean, their frustules progressively break down and dissolve, releasing the silicon back to the ocean in a form that can be used as a nutrient by other organisms. This process is slow and occurs mostly in the intermediate and bottom parts of the ocean. Scientists think that at least half of the diatoms dissolve between the surface of the ocean and 2,000 m depth [4]. Interestingly, because of this slow breakdown of diatoms, most of the silicon is recycled in the ocean layers where the water is flowing toward Antarctica. The silicon then travels back to the surface waters, where it will be consumed by new diatoms, closing the circle. Diatoms in the Southern Ocean therefore always have enough silicon to grow. The combination of these two processes: (i) transforming silicon (dissolved in seawater) into silica (within the frustule) at the surface, and (ii) bringing it to the deep ocean where it is recycled is called the silicon pump (Figure 3).
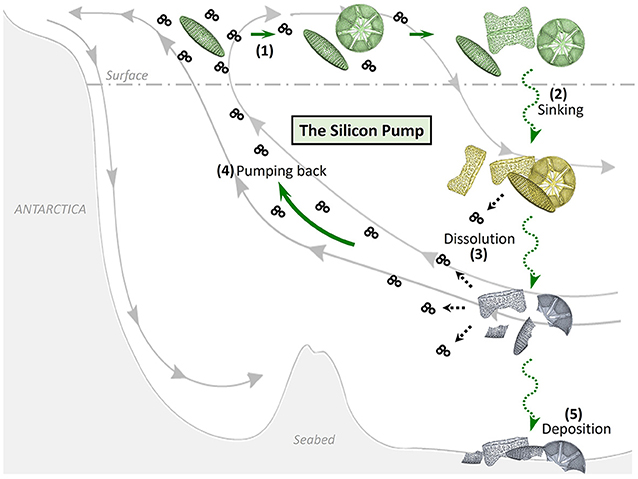
- Figure 3 - The silicon pump in the Southern Ocean.
- Silicon dissolved in seawater is used by diatoms at the surface of the ocean, to grow (1). When they die, diatoms sink (2), break down and dissolve (3), releasing the silicon back to the ocean. This recycled silicon is brought back to the surface (4) by the ocean circulation that is unique to the Southern Ocean. The diatoms that did not dissolve in the water reach the bottom of the ocean and accumulate to the seabed (5) to form the opal belt.
Currently, the silicon pump is very efficient and keeps most of the silicon in the Southern Ocean. As the surface current in the Southern Ocean flows northward, diatoms progressively consume all the silicon in the seawater. Therefore, when this water dives within the upper loop of the conveyor belt and escapes Antarctica, it contains almost no silicon. This low-silicon water is transported to the other oceans via the conveyor belt, and therefore will reduce or prevent the growth of diatoms there.
The Opal Belt: The Antarctic’s Unique Sediments
Only the diatoms that are not dissolved during their journey through the deep ocean reach the bottom of the ocean and the sediments. There, they accumulate and remain buried for a very long time (up to several million years). In the Southern Ocean, because diatoms are so numerous and active in the surface water, they make up the majority of particles that sink toward the bottom of the ocean. As a result, some regions of the ocean seabed can be made up of more than 80% diatom fossils [5] and form an extremely unique circle of silica-rich sediment all around Antarctica called the opal belt.
Conclusion: Is the Antarctic Silicon Trap Leaking?
The giant silicon trap around Antarctica holds more than half of the total amount of silicon existing in all the oceans. Scientists estimate that, today, very little of the silicon that enters the Southern Ocean (<5%) can escape this ocean trap, while other nutrients, such as nitrate and phosphate, escape more easily and are transported toward other regions of the ocean [4].
Evidence from diatoms buried within the Antarctic has shown that, at some periods in the past, the silicon trap has been weaker than we observe today. The lower efficiency of the trap allowed silicon to “leak” from the Southern Ocean and to be transported to the other oceans, through the upper loop of the conveyor belt. When more silicon escapes the Southern Ocean and is redistributed to the surface waters of other oceans, it allows more diatoms to grow and therefore more CO2 to be removed from the atmosphere via the biological pump of carbon. If this is true, it could partly explain the transitions between ice ages, when the Earth was much colder than today, and warmer periods like we have now. For example, the last ice age, which ended about 15,000 years ago, would have been characterized by a leaking silicon trap in Antarctica, allowing more diatoms to pump CO2 into the Atlantic, Pacific, and Indian oceans. The reduction of CO2 in the atmosphere from diatom pumping makes the atmosphere cooler because there is less CO2 capturing and storing heat, instead the heat is released out in space.
Of course, there is still much that scientists do not know about the silicon trap and its role in the regulation of Earth’s climate. Studying this topic involves working in the very cold, wild Antarctic environment, which, as you can imagine is quite challenging. However, scientists continue to do this important research, because despite the close link between the carbon and silica cycle, the models that predict future climate lack the silica component as it is a complex cycle.
Glossary
Antarctic Circumpolar Current (ACC): ↑ The Antarctic Circumpolar Current (ACC) is one of the strongest current in the world and it encircle Antarctica.
Overturning Circulation: ↑ It is the global ocean current that travel around all continent.
Diatoms: ↑ A group of small, single-celled algae that can capture silicon from water and light from the sun to build a transparent shell called frustule.
Frustule: ↑ Skeletal structure of diatoms made of silica.
Silica: ↑ A mineral that exists in various forms including sand, glass, and opal.
Biological Pump of Carbon: ↑ A set of processes, including photosynthesis performed by algae, that trap or “pump” CO2 from the atmosphere into the ocean, where it is stored for a long time.
Silicon: ↑ Chemical element that is found in silica, rocks, sand, glass, and opal.
Silicon Pump: ↑ This is the transfer of silica from the surface of the ocean to the deep layer due to diatom.
Conflict of Interest
The authors declare that the research was conducted in the absence of any commercial or financial relationships that could be construed as a potential conflict of interest.
Acknowledgments
This manuscript is part of a series of 6 manuscripts about the marine silicon cycle by the ECR SILICAMICS group. We thank the SILICAMICS ECRs consortium for its enthusiasm for putting this project together. We warmly thank the three young reviewers and their mentor for their sensible comments that greatly improved this manuscript. This work was supported by grants from the National Science Foundation #OCE-2048998 (IC) and from the European Union’s Horizon 2020 research and program Marie Skłodowska-Curie #899546.
References
[1] ↑ Lenton, A., Tilbrook, B., Law, R. M., Bakker, D., Doney, S. C., Gruber, N., et al., 2013. Sea-air CO2 fluxes in the Southern Ocean for the period 1990-2009. Biogeosciences 10:4037–4054. doi: 10.5194/bg-10-4037-2013
[2] ↑ Sarmiento, J. L., Gruber, N., Brzezinski, M. A., Dunne, J. P. 2004. High-latitude controls of the thermocline nutrients and low latitude biological productivity. Nature 427:56–06. doi: 10.1038/nature02127
[3] ↑ Tréguer, P., Bowler, C., Moriceau, B., Dutkiewicz, S., Gehlen, M., Aumont, O., et al. 2018. Influence of diatom diversity on the ocean biological carbon pump. Nat. Geosci. 11:27–37. doi: 10.1038/s41561-017-0028-x
[4] ↑ Holzer, M., Primeau, F. W., DeVries, T., Matear, R. 2014. The Southern Ocean silicon trap: data-constrained estimates of regenerated silicic acid, trapping efficiencies, and global transport paths. J. Geophys. Res. 119:313–331. doi: 10.1002/2013JC009356
[5] ↑ Chase, Z., Anderson, R. F., Fleisher, M. Q., Kubik, P. W. 2003. Accumulation of biogenic and lithogenic material in the Pacific sector of the Southern Ocean during the past 40,000 years. Deep-Sea Res. 50:799–832. doi: 10.1016/S0967-0645(02)00595-7