Abstract
The element silicon is everywhere! In fact, silicon is the second most abundant element in Earth’s crust. Silicon in rocks and minerals breaks down and is transported from rivers and streams into the world’s oceans. Many marine organisms need silicon as it is a crucial nutrient to build their skeletons. Silicon eventually reaches the seafloor, but its journey into the abyss is not straightforward due to biological, physical, and chemical processes. All these processes transport and transform silicon, creating a cycle that we call the marine silicon cycle. The silicon cycle is directly connected to the carbon cycle, making silicon a key player in the regulation of Earth’s climate. In this article, we discuss why we need to understand the marine silicon cycle, explain the steps that happen in the ocean, and demonstrate how the marine silicon cycle affects humans.
What is Silicon and Why is it Important?
Silicon is everywhere you look! It is in the rocks beneath your feet, the plants that help you breathe, in the skeletons of some animals, and even in our own bodies. Therefore, silicon is an essential nutrient that helps support all types of life. You will find silicon in almost every living organism, from bacteria to plants and animals, on land or in the oceans. Among the 118 elements known to exist in the universe, silicon is the seventh most abundant. On Earth, silicon is the second most abundant within Earth’s crust—the very upper layer of the Earth that we live on. This means that most of the rocks we see, touch, or sit on have silicon in them. Silicon is also everywhere inside our homes. It is used to build walls and floors, as silicon sand is an important part of concrete. It is a key part of electronics, and it makes up the main ingredient in glass, like the windows that let the sunshine in and the glasses you drink your juice and soda out of (as illustrated in Figure 1).
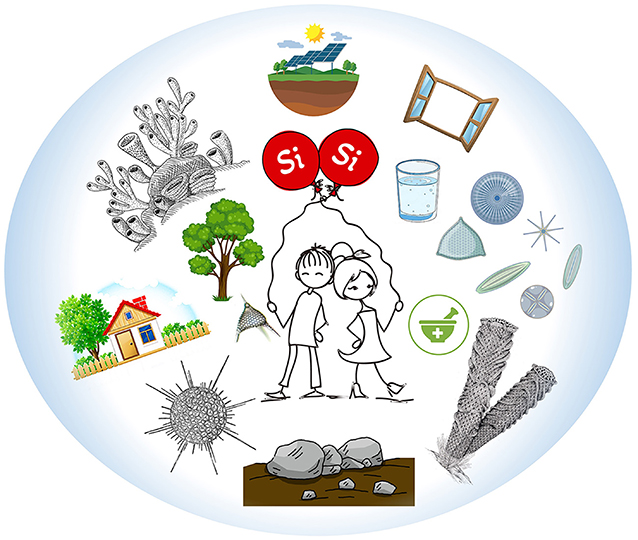
- Figure 1 - The element silicon (Si) is everywhere in our surroundings.
- It is found in the skeletons of organisms, in rocks, and in some manmade materials like glass and concrete. Silicon is critical for humans as well.
Each element on earth, including silicon, moves from the earth crust to the ocean and back to the earth crust, this is what we call the silicon cycle. Scientists study the silicon cycle because it tells us about the stability and wellbeing of our planet. Rocks and minerals on land break down over time into smaller pieces. From there, silicon travels in streams and rivers to the oceans, and ends up in the interior of the Earth, creating a cycle that keeps the environment stable. Fossil records (see this article for more info) have shown us that, for millions of years, silicon and carbon have been linked together. But how?
The silicon cycle starts on land, via a process called rock weathering. Rocks on Earth are naturally broken apart by water from the rain and rivers, or by the wind. A common example is the coastline being destroyed after a big storm passes through, but this process also happens at a smaller scale on land, especially in the mountains. There, water from rain and rivers mixes with rocks made of silicon (called silicate rocks) and creates a chemical reaction that uses up the carbon dioxide (CO2) in the atmosphere. When the climate is warmer, there is more CO2 in the air. This makes it easier for CO2 to react with silicate rocks, which helps to remove CO2 from the atmosphere. In this way, silicate rocks and rain participate in the natural balance of our climate [1]. This is what we call the silicate carbon sink [2]. It helps to balance the amount of carbon in the atmosphere and oceans, and it can help to reduce the effects of human impact on climate change. Weathering is not only important for removing atmospheric CO2, but it also releases dissolved silicon into the waters flowing into the rivers toward the ocean. This silicon is in a dissolved form (like when you put solid sugar or salt in water and it disappears), making it available as a nutrient for organisms on land and in the oceans.
How Does Silicon Get Into the Oceans and Where Does It Go?
Because rivers, streams, and water within the ground eventually flow into the oceans, the dissolved silicon in these waters also ends up in the oceans. This is also where a new part of the silicon cycle begins—the marine silicon cycle (Figure 2). Silicon eventually makes it to the bottom of the oceans, but not by a direct path, and it takes a very long time—maybe as much as 8,000 years [3]. In coastal areas, river waters and groundwaters supply the top layers of the ocean with dissolved silicon—a tasty new food for the organisms there. This ocean layer is home to many tiny organisms called phytoplankton that need sunlight to survive. Every spring and summer, the light from the sun and nutrients from the rivers create perfect growth conditions for a very important type of phytoplankton, called diatoms. Billions of diatoms grow in every ocean worldwide, but there is one special place on Earth where diatoms and other silicon-using marine organisms flourish, called the Southern Ocean.
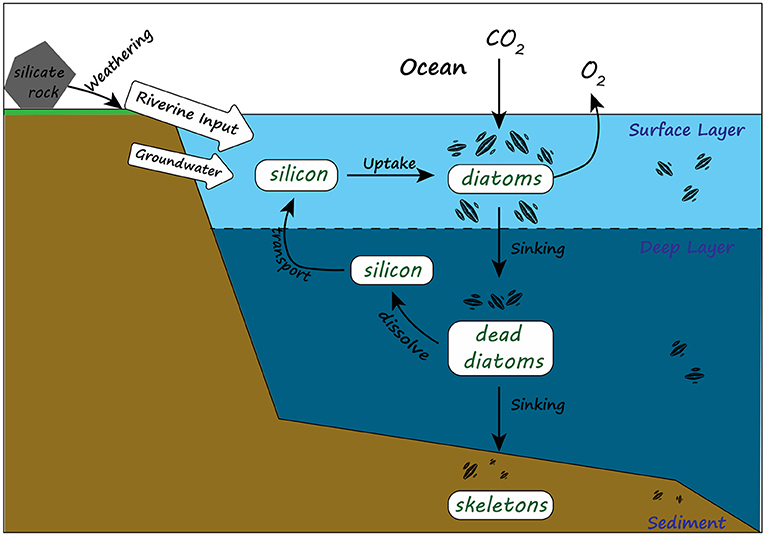
- Figure 2 - In the marine silicon cycle, silicon travels from the land into the oceans via rivers and groundwater.
- Near the ocean’s surface, silicon is taken up by phytoplankton like diatoms and incorporated into their skeletons. When these organisms die, they sink to the bottom of the ocean. Along the way, some of the skeletons dissolve and release silicon back into the water, but others end up buried in the sediment, along with the carbon they contain.
Diatoms use silicon to build their armor-like skeletons, much like we use calcium to build strong bones. The skeleton of diatoms is very strong compared to other phytoplankton. As diatoms grow, they eat up all the dissolved silicon, but they also remove CO2 from the surface waters of the ocean and produce most of the oxygen we breathe. At the end of summer, diatoms die and sink toward the deeper layers of the ocean. Some diatom skeletons dissolve, and some continue their journey to the very bottom of the ocean. There, on the deep, dark seafloor, diatom skeletons get buried in marine sediments (mostly made of mud). This means the carbon they have removed from the surface waters is buried, too. This very important process of CO2 removal from the atmosphere by sinking diatoms is called the biological carbon pump. Through this process, diatoms in all of the world’s oceans contribute to 40% of the oceanic carbon removal [4].
Silicon is also essential for other marine organisms such as sponges, and the zooplankton group called radiolarians (read more here). Just like diatoms, these organisms take part in the marine silicon cycle and help to remove CO2 from the atmosphere.
How is Silicon Relevant to Our Lives?
The silicon cycle is important in our everyday lives, even though we may not realize it. Understanding the past and present marine silicon cycle helps us to better predict how the environment will respond to climate change. It is especially important to understand how diatoms, radiolarians, and sponges will react to a rapidly changing environment. Diatoms are the first step in the marine food web. Zooplankton eat diatoms; larger fish and whales eat the zooplankton, and so on, moving energy up the food web. This means that if diatoms disappear from the ocean, the entire food web will suffer. Whales would not be the only ones without food, fishermen would be, too. This would have a lot of consequences because more than 3 billion people rely on fisheries.
Diatoms need special, stable conditions to grow. The climate has changed in the past, and so have diatoms, but these changes were slower compared to what we are experiencing today. Unfortunately, we have already observed a replacement of diatoms by other organisms in some places, for example in the Baltic Sea. These replacements disturb the food web and impact food availability for many people (read more here).
Diatoms, sponges, and radiolarians are also helping us to create better and more efficient designs in engineering. These organisms are great engineers themselves. The processes they use to transform silicon into silicon-based skeletons are of great interest. Their skeleton structures are beautiful but also very strong. Engineers are trying to recreate the same patterns used in the skeletons of diatoms, sponges, and radiolarian (Figure 3), which could make building structures or fabrics stronger. In addition, diatoms may be the perfect candidate to help us build the next generation of solar panels because their skeleton structure contains many holes that efficiently capture sunlight.
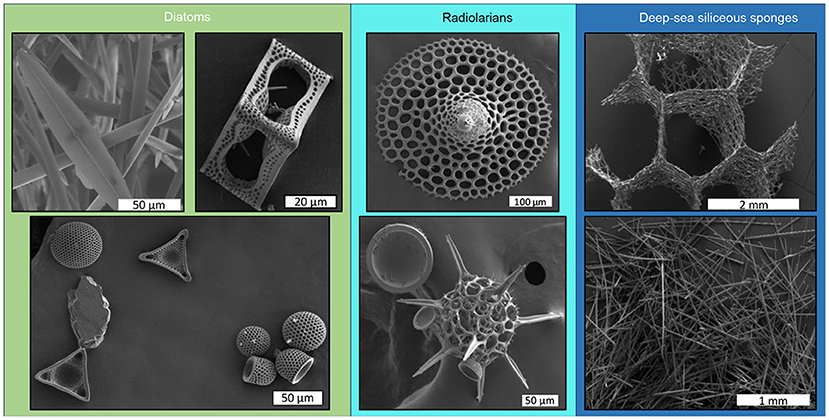
- Figure 3 - Pictures made with a powerful microscope (called a scanning electron microscope) showing examples of the silicon skeletons of diatoms, radiolarians, and deep-sea sponges.
- The scale bars show how tiny these skeletal structures are: 1 mm = 0.1 cm, 10 μm = 0.001 cm (Photo credits: Lucie Cassarino).
Deep sea sponges are another example of how amazing features of nature might help humans. Scientists are studying the connection between sponges and microbes (tiny organisms living within the sponges), which is giving them information on new molecules that could be used to produce human medicines. There are probably many more ways the silicon cycle can help us in our daily lives, so scientists have even more to discover.
In summary, the silicon cycle plays a key role in the health and stability of Earth’s ecosystems. It helps to regulate the levels of nutrients and carbon in the atmosphere and oceans. This, in turn, affects the planet’s health, which is vital for our survival and well-being. By studying the silicon cycle, scientists can gain a better understanding of Earth’s natural systems and how they are impacted by human activities. This knowledge can help us to make the best possible decisions about how we use and manage our natural resources.
Glossary
Silicon Cycle: ↑ The transfer of the element silicon from the earth crust, through the rivers and oceans, followed by its burial in the sediment to finally going back in the earth’s crust.
Rock Weathering: ↑ The process by which rocks, solid, and other materials are broken down and worn away by the effect of wind, water and the other natural forces over time.
Silicate Carbon Sink: ↑ This is the transfer of carbon from the surface of the ocean to the deep layer due to organisms made of silica.
The Marine Silicon Cycle: ↑ It represents the loop of the element silicon in the ocean. It describes how silicon enter the ocean, is transformed within the ocean and exits the ocean.
Phytoplankton: ↑ Tiny marine plant-like organisms that live in the water, like diatoms. They make their own food using sunlight and nutrients, produce oxygen and are an important part of aquatic food webs.
Biological Carbon Pump: ↑ A set of processes, including photosynthesis performed by algae, that trap or “pump” CO2 from the atmosphere into the ocean, where it is stored for a long time.
Zooplankton: ↑ Small aquatic animals that are an important part of the food web and play a key role in the carbon and nutrient cycles of aquatic ecosystems.
Marine Food Web: ↑ A complex system of interrelated food chains that exists in aquatic environments, from tiny plankton to large whales.
Conflict of Interest
The authors declare that the research was conducted in the absence of any commercial or financial relationships that could be construed as a potential conflict of interest.
Acknowledgments
This abstract is part of a series of six manuscripts about the marine silicon cycle put together by the ECR SILICAMICS group. We thank the SILICAMICS ECRs consortium for its enthusiasm for putting this project together. Authors thank the European Union’s Horizon 2020 research and program under the Marie Skłodowska-Curie grant agreement No 899546, Bundesministerium für Bildung und Forschung for the project “SO289—S Pacific GEOTRACES” funding, which supported this work.
References
[1] ↑ Zhang, S., Bai, X., Zhao, C., Tan, Q., Luo, G., Wang, J., et al. 2021. Global CO2 consumption by silicate rock chemical weathering: its past and future. Earths Future 9:e2020EF001938. doi: 10.1029/2020EF001938
[2] ↑ Tao, Z., Gao, Q., and Liu, K. 2011. Carbon sequestration capacity of the chemical weathering processes within drainage basins. Quat. Sci. 31:408–16. doi: 10.3969/j.issn.1001-7410.2011.03.02
[3] ↑ Tréguer, P., Sutton, J., Brzezinski, M., Charette, M. A., Devries, T., Dutkiewicz, S., et al. 2021. Reviews and synthesis: the biogeochemical cycle of silicon in the modern ocean. Biogeosciences 18:2269–89. doi: 10.5194/bg-18-1269-2021
[4] ↑ Tréguer, P., Bowler, C., Moriceau, B., Dutkiewicz, S., Gehlen, M., Aumont, O., et al. 2018. Influence of diatom diversity on the ocean biological carbon pump. Nat. Geosci. 11:27–37. doi: 10.1038/s41561-017-0028-x