Abstract
Have you ever wondered if today’s oceans were different millions of years ago? Well, a group of small algae called diatoms can help us to find this out. Diatoms build a strong glass skeleton, like a shell, which can last for thousands and even millions of years after their deaths. To build their glass skeletons, diatoms take up silicon from the seawater, similar to us eating food to build our bodies. Diatoms preferentially use one type of silicon in their menu, leaving behind the type they do not like. Researchers can track this eating habit by measuring the proportion of the two types of silicon stored within diatoms. Using this silica-print like a fingerprint, scientists can investigate what the surface ocean was like, how much diatoms were eating silicon, and how these organisms have affected Earth’s past climate.
What Are Diatoms And Why Are They Important For The Climate?
Diatoms are tiny algae that live almost everywhere near the surface of oceans, lakes, and rivers. They stay near the surface because, just like any plant, they need light to produce energy through the process of photosynthesis (for more information about photosynthesis in the oceans, see this Frontiers for Young Minds article). To grow, diatoms also need nutrients, which are the equivalent of the food we eat.
Unlike our bones, the skeletons of diatoms are outside their bodies, like a shell, and they are made of silica (also called opal), which is the same material we use to make glass. Therefore, diatom skeletons are transparent. When diatoms die, they sink to the bottom of the ocean, carrying with them all the CO2 they used to grow and create their skeletons while they were alive (Figure 1C) [1]. Therefore, diatoms are very important players in climate regulation, because they remove CO2 from the air, which helps to reduce climate change. On the seafloor, the opal skeletons do not fall apart easily and can remain buried for millions of years. Through this process, diatoms are responsible for up to 40% of the carbon removed from the atmosphere by the oceans.
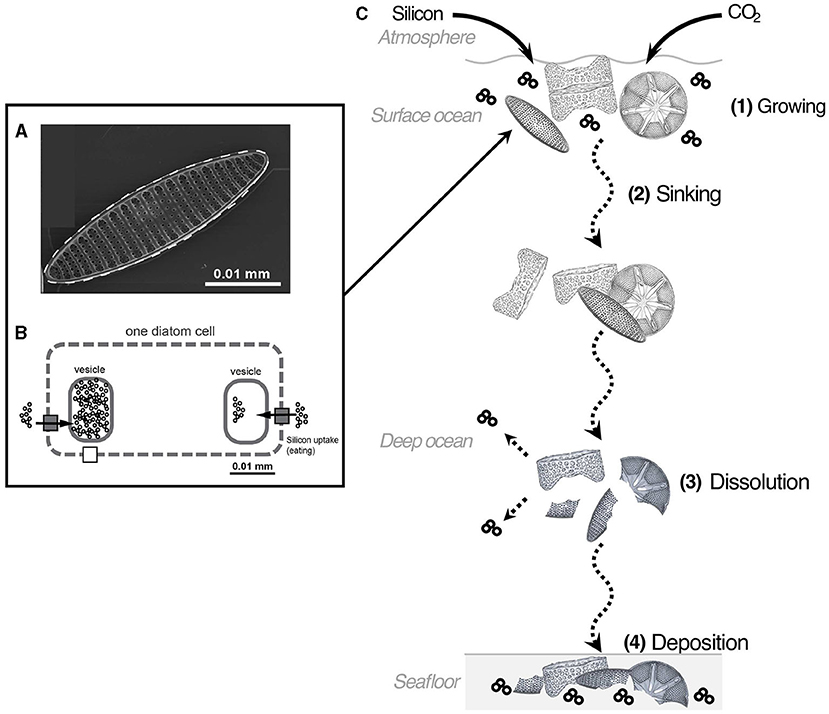
- Figure 1 - (A) A diatom seen through a microscope.
- (B) Silicon enters the diatom cell and is collected in vesicles, where it is transformed into opal crystals. The opal crystals form the diatom’s skeleton (gray dashed line). (C) At the ocean’s surface, diatoms take up CO2 and silicon and use light to grow. When they die, they sink and carry the CO2 and silicon into the deep ocean. Parts of the skeleton dissolve, releasing CO2 and silicon back into the water. Undissolved pieces remain on the seafloor, where they can store carbon (from CO2) and silicon for a very long time.
Diatoms are so tiny that you need a microscope to see them (Figure 1A). Only the biggest can reach a length of 0.5 mm and are about as wide as one of your hairs. By collecting samples from the seafloor, scientists can find diatom skeletons and use those skeletons to gather information about ocean conditions in the past and present. This research field is called paleoceanography.
How Do Diatoms Build Their Glassy Skeletons?
To build their skeletons, diatoms need silicon, which they find dissolved in seawater. They store this silicon in small pockets called vesicles inside their bodies, where they can transform the silicon into small opal crystals (Figure 1) [2]. They use these opal crystals to build their skeletons, which are decorated with spines and holes. In some places, diatoms use all the silicon from surface water, leaving nothing behind. We know that they do so because scientists have found a way to track their fingerprints—or should we say their silicon-prints—in the ocean and on the seafloor. To do so, scientists measure silicon isotopes, which is a powerful way to understand what diatoms were doing in the past, as well as what the conditions were like for these diatoms.
Did You Say Isotope?
Atoms are the main building blocks of every existing thing. Atoms are made of small parts called protons, electrons, and neutrons (Figure 2). An atom is identified by the number of protons it contains. For example, a silicon atom contains 14 protons. In most cases, an atom contains the same number of neutrons as protons, meaning 14 neutrons for silicon. The number of protons plus the number of neutrons gives the mass number of the atom. For example, most silicon atoms have a mass number of 28. However, in rare cases, silicon can have 16 neutrons instead of 14, so its mass number will be 30 instead of 28. These little variations in the number of neutrons produce “light” atoms of silicon (with a mass number of 28) and “heavy” atoms of silicon (with a mass number of 30). Those lighter and heavier atoms of silicon are called isotopes. In nature, the most abundant silicon isotope has a mass number of 28 and is called 28Si, and the least abundant has a mass number of 30 and is called 30Si.
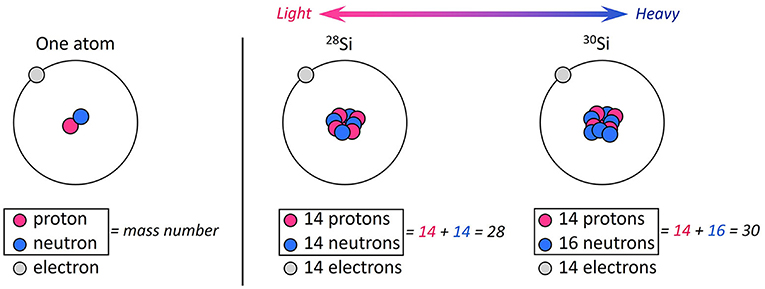
- Figure 2 - An atom contains protons and neutrons in its nucleus and electrons spinning around them.
- The number of protons defines the element. The sum of neutrons and protons gives the mass number of the element. The number of neutrons can change, which does not change the type of element but does change its mass number. An atom of silicon has 14 protons (pink circles) and can have 14 or 16 neutrons (blue circles), changing the mass number from 28 to 30. Atoms of elements with different numbers of neutrons are called isotopes. Here you can see the isotopes 28Si and 30Si.
Why Do Diatoms Care About Silicon Isotopes?
Diatoms prefer to use the lightest silicon isotope (28Si) when they build their skeletons. In scientific terms, they fractionate silicon isotopes, which means they preferentially take up the lighter isotope (28Si) to a certain amount [3]. This might sound complicated, but here is an example that might help you understand.
Diatoms are just like you and me: they prefer certain foods. Imagine having a bag full of pink and blue jellybeans in equal proportions. You like both, but you have a small preference for pink jellybeans. Because you are wise, you decide not to eat the whole bag at once, but to eat only a small fraction of jellybeans daily. On the first day, you obviously grab more pink jellybeans than blue ones because pink is your favorite. The second day, you do the same thing, but you notice that there are now more blue than pink jellybeans in your bag. On the last day, it is difficult to find pink jellybeans in your bag, and you end up with more blue jellybeans than pink in your hand. By preferentially eating pink jellybeans, you changed the ratio of jellybeans colors in your bag and your hand. You have increased the ratio of blue jellybeans (your less favorite) in the bag and your hand.
Diatoms do the same thing with silicon isotopes in the ocean (Figure 3). They prefer to use the 28Si isotope so, as they continue to take up their favorite silicon isotope from the water, they increase the ratio of 30Si that remains in the water. This means that the diatoms that grow after them will have to form opal with more 30Si [4].
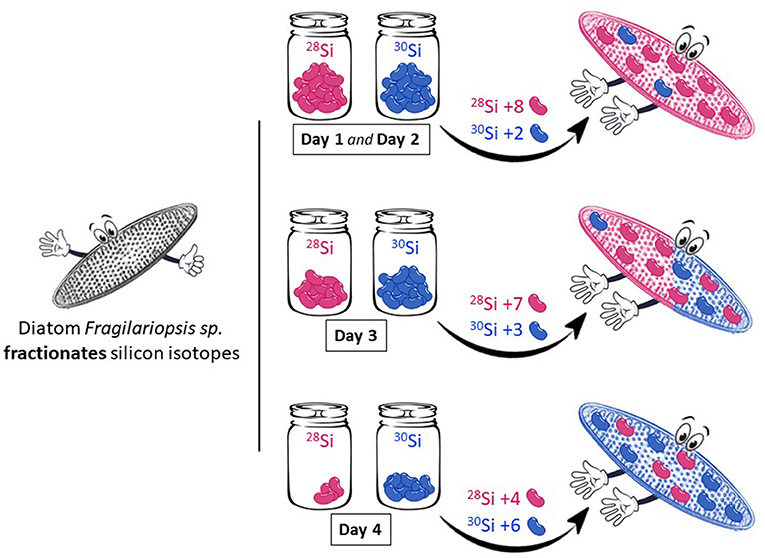
- Figure 3 - Diatoms of the species Fragilariopsis sp. fractionate (or favor) the lightest isotope (28Si, pink jellybeans).
- By choosing more 28Si compared to the heavier isotope 30Si (blue jellybeans), the diatoms change the proportion of silicon isotopes available in seawater (the jars) and within their skeletons. With time, both the seawater and the diatoms become enriched in 30Si because most of the 28Si has been used up.
Reconstructing The Past With The Silica-Print
Because of fractionation, diatom activity leaves an imprint (or silicon-print) in both seawater and in their opal skeletons. If diatoms take up a lot of silicon, they will eventually have to incorporate more 30Si into their skeletons because that is the isotope left over after they use up their favorite, 28Si. On the other hand, when diatoms take up less silicon, they will use less 30Si. When diatoms die, they sink to the seafloor where they are buried, saving this silicon-print. By measuring the proportion of the two silicon isotopes in these old diatom skeletons from the seafloor, paleoceanographers can investigate diatoms’ activity in the surface water in the past.
To do this, paleoceanographers use ships equipped with devices that collect seawater from the ocean surface and diatoms from the seafloor. Then, scientists remove the silicon from the seawater and the diatoms’ skeletons in their laboratories and use instruments that measure the ratio between the two silicon isotopes. We call this ratio δ30Si (pronounced “delta-30-silica”). When δ30Si is high, there is more 30Si in the sample, and when δ30Si is low, there is more 28Si in the sample [4, 5].
By measuring δ30Si in the seawater, scientists can calculate the amount of silicon that diatoms have taken up in the last week or the last month. When measuring δ30Si in the diatoms preserved on the seafloor, paleoceanographers can investigate how much silicon was used by diatoms that lived in the ocean thousands of years ago. Therefore, scientists can find out how abundant and healthy diatoms were and if they could absorb a lot of CO2 from the atmosphere by photosynthesis in the past.
Do We Know Everything About Diatoms’ Silicon Isotope Eating Habits?
The investigation of silicon-prints by measuring silicon isotopes in diatoms’ skeletons works very well. But scientists still have many questions regarding the fractionation of silicon isotopes by diatoms. For example, we assume that diatoms always use the two silicon isotopes in the same ratio. That means the proportion of 30Si to 28Si they take up does not change. To use the jellybeans example, this is like assuming you always take the same proportion of pink and blue jellybeans. Today, we are starting to think that this ratio can change, but we still do not know why. Maybe different types of diatoms have different preferences and fractionate silicon isotopes differently. Diatoms might also change the ratio of the two silicon isotopes they normally use depending on surrounding conditions, such as the availability of other nutrients and light. These important questions need to be answered because we use silicon isotopes to reconstruct how diatoms influence ocean chemistry and how they have interacted with the climate in the past. We need to fully understand how things work in modern times to accurately evaluate what happened in the past.
Despite all the unknowns that still need to be explored, silicon isotopes are a powerful tool that helps us to track the activity of diatoms in the past and in today’s oceans, and to better understand the role of the ocean in the regulation of Earth’s climate. Eventually, this information will allow researchers to better predict how the environment will respond to climate change and how the consequences of human activities affect the health of ocean ecosystems.
Glossary
Photosynthesis: ↑ A process by which plants/algae produce oxygen and food for themselves and other organisms using sunlight and CO2.
Climate: ↑ The average long-term pattern of weather in a region across the Earth.
Paleoceanography: ↑ The study of past ocean conditions.
Vesicle: ↑ A structure inside a cell, consisting of liquid enclosed by an additional membrane. Vesicles transport and store material within the cell.
Isotopes: ↑ Atoms of the same element with the same number of protons but a different number of neutrons and, thus, a different mass number.
Mass Number: ↑ The number of protons plus the number of neutrons of an atom.
Fractionation: ↑ The preferred ratio of two isotopes that are taken up during a specific process.
Acknowledgments
This manuscript is part of a series of 6 manuscripts about the marine silicon cycle by the Early Career Researchers SILICAMICS group. We thank the SILICAMICS ECRs consortium for its enthusiasm for putting this project together. We warmly thank the four young reviewers and their mentors for their sensible comments that greatly improved this manuscript. This work was supported by grants from the National Science Foundation #OCE-2048998 (IC).
Conflict of Interest
The authors declare that the research was conducted in the absence of any commercial or financial relationships that could be construed as a potential conflict of interest.
References
[1] ↑ Tréguer, P., Bowler, C., Moriceau, B., Dutkiewicz, S., Gehlen, M., Aumont, O., et al. 2018. Influence of diatom diversity on the ocean biological carbon pump. Nat. Geosci. 11:27-37. doi: 10.1038/s41561-017-0028-x
[2] ↑ Martin-Jézéquel, V., Hildebrand, M., and Brzezinski, M. A. 2000. Silicon metabolism in diatoms: implications for growth. J. Phycol. 36:821-40. doi: 10.1046/j.1529-8817.2000.00019.x
[3] ↑ Fry, B. 2006. Stable Isotope Ecology. New York, NY: Springer.
[4] ↑ De La Rocha, C. L., Brzezinski, M. A., and DeNiro, M. J. 1997. Fractionation of silicon isotopes by marine diatoms during biogenic silica formation. Geochimica et Cosmochimica Acta. 61:5051-6. doi: 10.1016/S0016-7037(97)00300-1
[5] ↑ De La Rocha, C. L., Brzezinski, M. A., DeNiro, M. J., and Shemesh, A. 1998. Silicon-isotope composition of diatoms as an indicator of past oceanic change. Nature 395:680-3. doi: 10.1038/27174