Abstract
Have you ever wondered how scientists investigate human diseases and develop treatments to improve human health? For many years, the main way to do so was to use research animals. Animal studies have greatly improved our understanding of human diseases, but some disorders are unique to humans, which limits the usefulness of research animals. So scientists began using a special type of human cells, called embryonic stem cells. These cells are present in the body only during the early stages of development, and they can give rise to all types of cells and tissues. How can we produce stem cells from patients with certain diseases? How can we make these cells develop into the type of cell affected by the disease and use them to test new drugs in the lab? In this article, we explore how human stem cells can help us understand and treat human diseases.
Experimental Models of Diseases
While working as a country doctor, the English surgeon Edward Jenner made an interesting observation. Young dairymaids who suffered an attack of cowpox, which is a relatively mild disease that they could catch from cattle, could not become infected with smallpox—a related, dangerous disease that killed millions of people all over the world. On May 14th, 1796, Jenner conducted an experiment: he infected 8-year-old James, the son of the family gardener, with cowpox from a dairymaid, and then Jenner infected the boy again with liquid contaminated with smallpox. Luckily, James did not develop smallpox, and this is how the best method for avoiding infectious diseases came about: vaccination.
Doing a similar experiment today would be inconceivable! Medical research involving humans has major restrictions that are designed to protect the participants from any harm that might be caused by new treatments. This change was a challenge to scientists seeking to study and treat human diseases—how could they test their hypotheses? To overcome this challenge, scientists came up with methods to mimic human disease states in the lab, without using humans. These methods, which are called experimental models of disease, allow scientists to study the causes of diseases and their possible treatments (Figure 1).
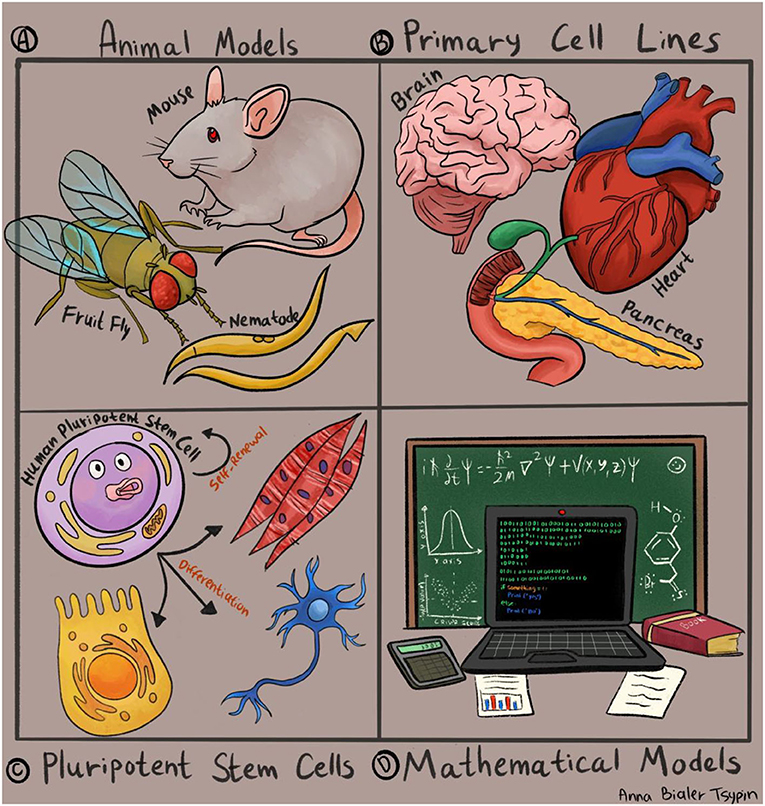
- Figure 1 - Experimental systems used by scientists to study human diseases.
- (A) Animal models such as worms, flies, and mice. (B) Experiments using cell and tissue samples taken from human organs, such as the heart, brain, or pancreas. (C) Human pluripotent stem cells, which are capable of differentiating into all cell types. (D) Mathematical models and computer simulations.
The earliest and most well-established models for human diseases were developed in animals (Figure 1A). Over the years, much effort has been invested into mimicking human diseases in animals like rats, mice, fish, and even flies and worms. Since humans share many similarities with these animals, animal research has taught us a great deal about human disorders and has even led to groundbreaking discoveries in medicine. The study of diabetes is a well-known example. Diabetes is a condition caused by high blood sugar levels, which are controlled primarily by the pancreas. Dogs that had their pancreases surgically removed were the earliest models of diabetes, and they were used to discover the link between the function of the pancreas and the development of diabetes. Dogs even helped scientists to identify a life-saving treatment: insulin.
However, in many cases, the differences between humans and animals make it challenging to mimic diseases in animal models. In these cases, the results from animal experiments do not predict the results that would have been obtained in humans. For this reason, scientists have been trying to find ways to study diseases directly in human cells.
In some cases, scientists collect tissue samples from patients with various diseases and compare these samples with tissues collected from healthy individuals, to find the differences between unhealthy and healthy tissues (Figure 1B). In some conditions, such as certain cancers, it is relatively easy to obtain a tissue sample, to isolate cancer cells in the lab, and to conduct experiments to determine the differences between a normal cell and a cancer cell. But what about diseases that affect the cells of the heart or the brain? These cell types are more difficult to isolate, and even if samples are obtained, it is often challenging to grow these cells in the lab and conduct experiments with them.
Pluripotent Stem Cells: A Novel Model For Human Disorders
In recent decades, scientists have overcome some of the limitations of research animals and human tissue samples by using a special type of cells, called embryonic stem cells. Embryonic stem cells are present in the body only at a very early stage of development, about a week after fertilization. At this stage, the embryo consists of a tiny handful of unique cells, which have two distinctive properties. First, these cells can divide indefinitely; and second, they can differentiate, which means they can develop into all types of cells and tissues, like heart cells, brain cells, lung cells, and many more. This quality is also known as pluripotency (Figure 1C).
To use embryonic stem cells for research purposes, scientists first had to figure out how to grow them in the lab, while keeping them in the pluripotent state. At the same time, scientists searched for ways to make the stem cells develop into different cell types: brain cells, heart cells, blood cells, or whatever they were interested in.
Initially, the only way to isolate embryonic stem cells was using donations of very early embryos that were created by in vitro fertilization (IVF), a procedure that helps couples who are having trouble getting pregnant (Figure 2A). In cases when one or both parents is known to carry a disease-causing mutation in their DNA, special tests can identify embryos that inherited the mutation and will be affected by the disease. Normal embryos that do not carry the mutation allow couples to have healthy children, while the embryos that inherited the mutation can be used for obtaining embryonic stem cells.
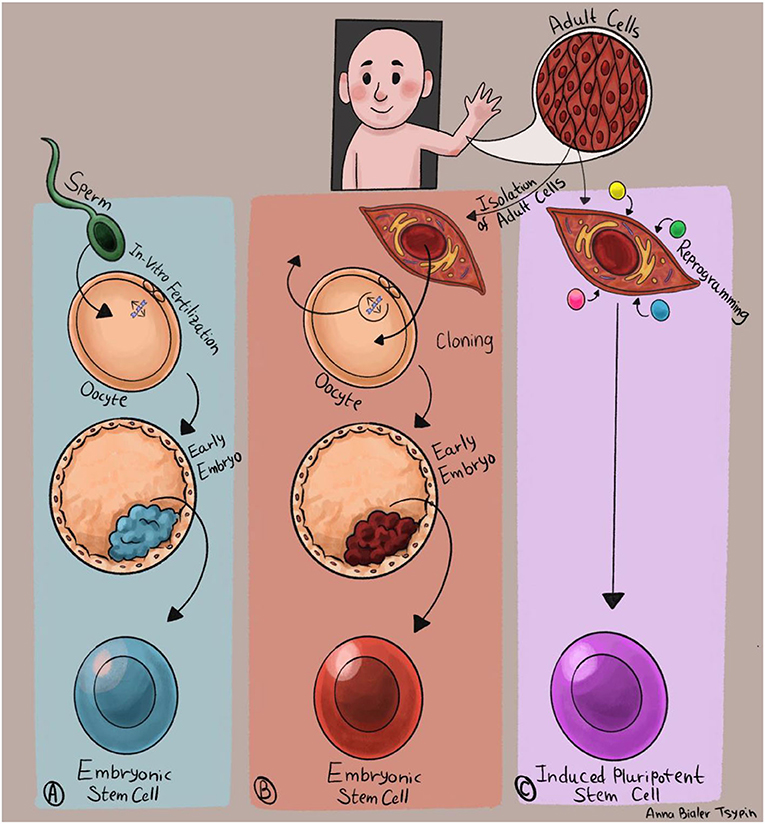
- Figure 2 - Producing human pluripotent stem cells.
- (A) The encounter between a sperm and an egg creates a tiny embryo, from which embryonic stem cells are produced. (B) DNA of a mature cell is injected into an emptied egg cell. The egg cell environment causes the DNA to return to its embryonic form, and the cloned egg develops into an embryo from which pluripotent stem cells are obtained. (C) Mature cells are isolated from a patient and injected with a combination of four genes that cause them to undergo reprogramming and acquire the characteristics of embryonic stem cells.
However, using 1-week-old embryos raises both ethical and technical issues, and therefore researchers have been working to produce pluripotent stem cells without using embryos. One way to do this is cloning: a method by which pluripotent stem cells can be generated from adult cells [1]. For the cloning process, a human egg cell (also known as an “oocyte”) is emptied of its DNA and injected with the DNA of a mature cell (Figure 2B). The unique environment of the empty egg cell causes the adult DNA to return to its embryonic form. The cloned egg, containing the DNA from the mature cell, starts to develop into a tiny embryo, producing embryonic stem cells. The advantage of cloning is that this process makes it possible to create embryonic stem cells using the cells of an adult patient, and those cells will contain that person’s unique genetic material. The disadvantage is that cloning is a complex process, which requires the use of human eggs.
In recent years, another breakthrough has taken place in the stem cell field. Japanese scientist Shinya Yamanaka has shown that it is possible to transform adult cells directly into pluripotent stem cells, through a process called reprogramming [2]. The cells obtained by this process are called induced pluripotent stem cells (Figure 2C). Yamanaka’s discovery revolutionized the field of stem cells for human disease research. Through reprogramming, pluripotent stem cells can now be produced directly from patients with any disease, grown in the lab, and transformed into the cell types affected by the disease.
Obtaining The Desired Cell Type
Suppose we used one of the described methods to generate pluripotent stem cells carrying a genetic mutation responsible for a disease that we are interested in studying. How can we use the unique properties of these stem cells to learn about the causes of the disease and to develop novel treatments (Figure 3)?
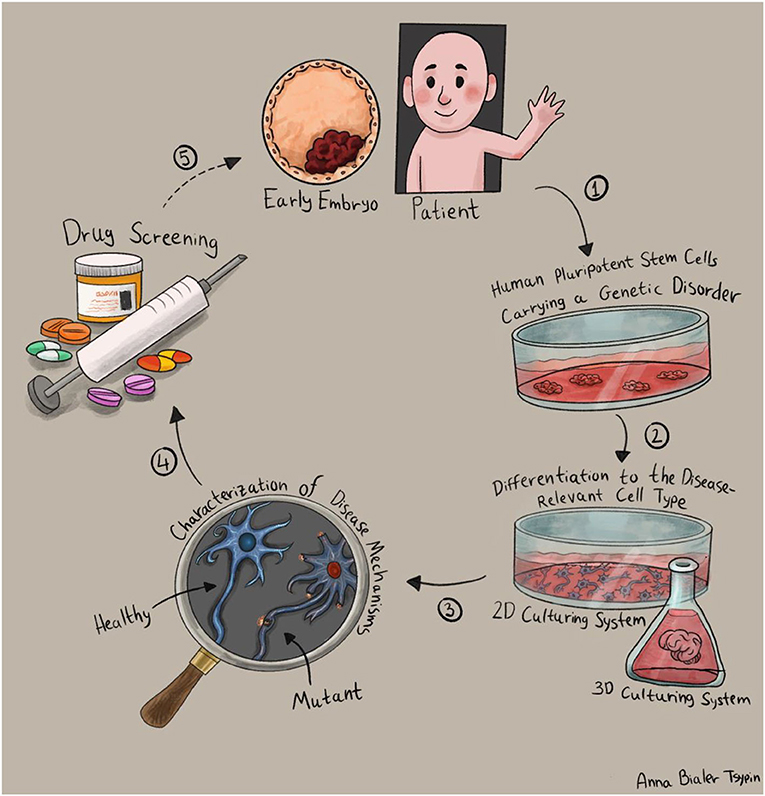
- Figure 3 - Studying diseases using stem cells.
- (1) First, we generate pluripotent stem cells with the disease-causing mutation, either by using embryonic stem cells or induced pluripotent stem cells. (2) We obtain the cell type affected by the disease, by stimulating the stem cells to grow into our cells of interest: two-dimensional (left) or three-dimensional growing methods that produce miniature versions of organs, called “organoids” (right). (3) We study the differences between healthy and unhealthy cells, to understand the disease and develop treatments. (4) We screen possible drugs to treat the disease. (5) Finally, we transition from the lab to medical trials in patients.
First, we must transform the stem cells into the specific type of cells damaged by the disease. For example, if we are investigating a disease that causes intellectual disability, we should study brain cells; and to study a disorder that affects the heart, we should study heart cells. To make the stem cells develop into the desired cell type, we must adapt their growth conditions and expose them to signals that guide their development into that cell type. The methods scientists use to convert stem cells into various cell types are still evolving, and there are many “recipes” for creating unique cell types in the lab. Recently, three-dimensional growing methods have been developed that cause pluripotent stem cells to create miniature versions of real organs. These scaled-down versions of human organs are called organoids [3].
Stem Cells For Treatment and Drug Screening
Once we have obtained the cell type damaged by the disease, we need to understand how the mutation disrupts the function of the cells. Does it interfere with the structure of the cell, its division, or maybe its chemical or electrical activity? When it comes to cells that we grow in the lab, this is a very complex question—we need to understand how the changes we see in the lab reflect the behavior of the cells in the body. Despite this challenge, testing the differences between healthy and disease-affected cells is extremely important because it allows us to understand the mechanism that causes the disease and to come up with treatment strategies.
One of the important benefits of using stem cells is the ability to test different types of treatments directly on patients’ cells without endangering the patients themselves. The ease with which the cells can be grown and developed in the lab allows scientists to test thousands of drugs to find medications that can “fix” the abnormalities in patients’ cells. In addition, since pluripotent stem cells can be produced directly from human patients, the treatment can be personalized to the specific genetic mutation the patient carries.
Scientists are also examining the use of pluripotent stem cells as a treatment. Some diseases are caused by damage to a specific cell population that is necessary for one of the body’s functions. For example, Parkinson’s disease is caused by the destruction of a specialized population of brain cells essential for controlling body movements. If we could transform pluripotent stem cells into the type of brain cells damaged in Parkinson’s disease and transplant these cells into patients’ brains, we might be able to improve the patients’ symptoms.
Summary
Pluripotent stem cells have enormous medical potential. In recent decades, research models using pluripotent stem cells have been developed for many disorders [4]. New methods have allowed the production of numerous cell types and the testing of many drugs in the lab. Although many studies have used pluripotent stem cells to investigate human conditions, many questions remain unanswered. What gives stem cells their miraculous ability to form all types of cells in the body? Will advanced growing methods succeed in creating real organs in the lab, addressing the severe shortage of human organs for donations? What is the right way to transition between experiments in the lab and tests in patients? Many more studies will be required in the future, to dig deeper into these subjects.
Glossary
Cancer: ↑ A condition in which cells from a certain tissue change their properties, divide, and grow out of control.
Cell: ↑ The smallest and most basic unit of life. Our body is made of many different cell types, organized into specialized tissues and organs: brain cells, muscle cells, skin cells, and more.
Fertilization: ↑ The encounter between a sperm cell and an egg cell that leads to the formation of the embryo.
Differentiate: ↑ To develop from a stem cell into a cell with a defined function, such as a brain cell, a heart cell, a blood cell, or a skin cell.
Pluripotency: ↑ A Latin term (“pluri”—“a lot,” “potency”—“ability”) which describes the capability of embryonic stem cells to develop into all types of cells in the body.
In Vitro Fertilization (IVF): ↑ A process in which a sperm cell and an egg cell are joined together outside of the body, forming a tiny embryo that can be implanted into the uterus.
DNA: ↑ The genetic material found in every cell of our body and contains the “instructions manual” for all the body’s functions and characteristics.
Reprogramming: ↑ Conversion of mature cells to pluripotent stem cells through the introduction of four genes involved in embryonic development. The resulting reprogrammed cells are known as induced pluripotent stem cells.
Conflict of Interest
The authors declare that the research was conducted in the absence of any commercial or financial relationships that could be construed as a potential conflict of interest.
Acknowledgments
We thank Anna Bialer Tsypin for her help in preparing the illustrations.
References
[1] ↑ Tachibana, M., Amato, P., Sparman, M., Gutierrez, N. M., Tippner-Hedges, R., Ma, H., et al. 2013. Human embryonic stem cells derived by somatic cell nuclear transfer. Cell. 153:1228–38. doi: 10.1016/j.Cell.2013.05.006
[2] ↑ Takahashi, K., and Yamanaka, S. 2006. Induction of pluripotent stem cells from mouse embryonic and adult fibroblast cultures by defined factors. Cell. 126:663–76. doi: 10.1016/j.Cell.2006.07.024
[3] ↑ Lancaster, M. A., and Knoblich, J. A. 2014. Generation of cerebral organoids from human pluripotent stem cells. Nat. Protoc. 9:2329–40. doi: 10.1038/Nprot.2014.158
[4] ↑ Avior, Y., Sagi, I., and Benvenisty, N. 2016. Pluripotent stem cells in disease modelling and drug discovery. Nat Rev Mol Cell Biol. 17, 170–82. doi: 10.1038/nrm.2015.27