Abstract
Cardiomyocytes are the muscle cells that make the heart beat, pump oxygen, and deliver nutrient-rich blood throughout the human body. During a heart attack, the blood supply to the heart is interrupted. Cardiomyocytes then die and are replaced by scar tissue that can no longer contract. As a result, the heart is weakened and may beat abnormally. For many years, researchers have been searching for a way to replace damaged cardiomyocytes with new ones. Stem cells are master cells that grow and divide rapidly. They may be ideal for repairing organs and tissues because they can turn into many different cell types, including cardiomyocytes. Among other medical therapies, stem cells have been used to develop the cardiac patch, a heart “band-aid” that can regenerate damaged heart muscle. In this article, we will discuss the advantages and limitations of using stem cells for repairing a “broken heart.”
How Does The Heart Work?
Every cell in the body needs oxygen and nutrients to survive. Blood is the body’s delivery service, bringing cells the materials they need. Your heart is the pump that moves the blood through your body, whether you are sleeping, eating, cycling, or playing video games. The heart is constantly at work, beating about 100,000 times every single day [1]. The heart has four main chambers, which are like stations for blood (Figure 1). The oxygen-poor blood returning to the heart after its long journey through the body empties into the right atrium, then flows into the right ventricle. When the heart squeezes, or contracts, the right ventricle pumps the blood to the lungs, where it is replenished with oxygen from the air that you breathe. The oxygen-rich blood then returns to the heart, emptying into the left atrium, and then moving into the left ventricle. Finally, the left ventricle contracts again, pumping oxygen-rich blood to all the cells and organs in your body. Four heart valves control blood flow between each of these chambers. The valves open and close as the blood flows from chamber to chamber, preventing it from flowing backwards. You can think of these valves as the heart’s “traffic-control operators.” They make sure the blood flows in only one direction through the “one-way streets” of the heart. How is it possible for your heart to beat without you even thinking about it? Behind the scenes, the heart has its own little brain, called the sinoatrial (SA) node. The SA node controls your heart’s activity independently from the brain. It sends electrical signals that control the synchronized muscle contraction. In addition, every chamber of the heart has mechanisms to take over the beating function if the SA node were to fail.
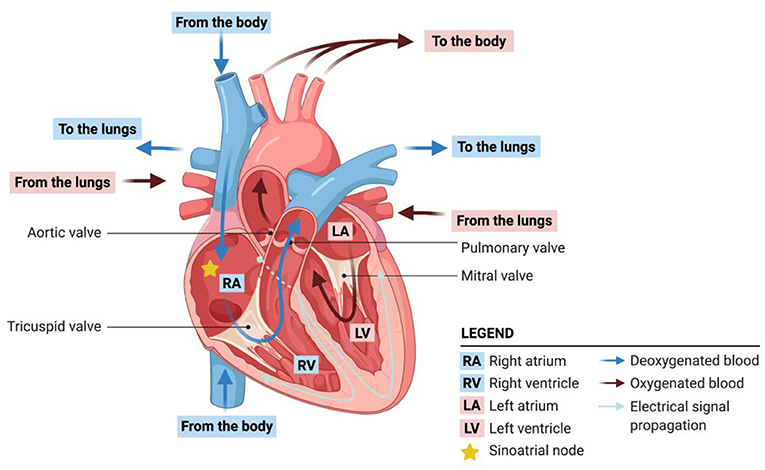
- Figure 1 - Blood circulation and electrical signal flow in the heart.
- The heart maintains a steady circulation of blood throughout the body. Blood that is low in oxygen comes into the heart from the body, flows through the right atrium and right ventricle and is pumped to the lungs, where it is oxygenated. Oxygenated blood returns to the heart through the left atrium, flows to the left ventricle, and is them pumped back out to the body. Each heartbeat is initiated by electrical signals that coordinate the contraction of cardiomyocytes.
The muscle cells of the heart are called cardiomyocytes. The average human heart is estimated to contain 2–3 billion cardiomyocytes [2]. How do all these cells know when to contract to produce a single powerful heartbeat? The cells’ membranes interlock at special junctions that anchor the cells together and allow for the passage of messenger molecules that instruct the cells to beat. As such, all the cardiomyocytes can behave as a single functional unit. The cardiomyocytes work together to pump blood through your body.
When The Heart Fails: Tale Of A Heart Attack
Much like the cells of other body organs, cardiomyocytes also need oxygen and nutrients to be functional. As such, the heart has its own network of blood vessels—arteries and veins that wrap around the entire heart. The heart may seem like the perfect organ, but it is not without flaws. Heart attacks are very common and they occur when one or more coronary (heart) arteries becomes blocked, interrupting blood flow. What can cause a blockage in a coronary artery? Most heart attacks are caused by coronary artery disease. Atherosclerosis is a type of coronary artery disease that causes fatty deposits to build up in arteries and form structures called plaques. Over time, these plaques harden and narrow the arteries, obstructing the flow of oxygen-rich blood. Plaques become particularly dangerous when they break off and obstruct the circulation. Sometimes ruptured plaques can completely cut off the blood circulation, depriving the heart’s cells of oxygen and nutrients. You can think of this blockage like a massive traffic jam. Without a fresh supply of oxygen or nutrients, heart muscle cells become damaged or die. The consequence is a heart attack.
Why is a heart attack so dangerous? First, when the heart’s cells become starved for oxygen and nutrients, they are no longer functional. They do not have the materials they need to beat. As a result, the heart muscle can no longer contract, and the blood supply to the rest of the body is disrupted. Even if a doctor fixes the blockage and restores blood flow after a heart attack, if the blood flow was disrupted for a few hours, the damaged cardiomyocytes will never come back. A heart attack can cause the loss or damage of up to a billion cardiomyocytes [3]. The damaged cardiomyocytes are replaced by scar tissue, which can no longer help the heart to pump blood and can cause an abnormal heartbeat, which can severely weaken the heart and may cause sudden death.
Stem Cells Can Become Heart Cells
Have you ever wondered where cardiomyocytes and the other types of cells in your body come from? All your body’s cells start out as stem cells (Figure 2). Stem cells can develop into many different cell types, ranging from skin cells to nerve cells to heart cells. Stem cells divide at a very fast rate, and they have not yet specialized and taken on specific jobs in the body. Just as kids eventually grow up and choose a profession, stem cells mature and become the body’s specialized cells. Stem cells originate from two main sources: adult tissues or embryos. However, only embryonic stem cells can mature into all cell types in the body. Scientists have developed methods to genetically reprogram adult cells into what are called induced pluripotent stem cells. Both embryonic and induced pluripotent stem cells are pluripotent, which means they have an unlimited ability to divide and they can differentiate (change) into any of the body’s cell types.
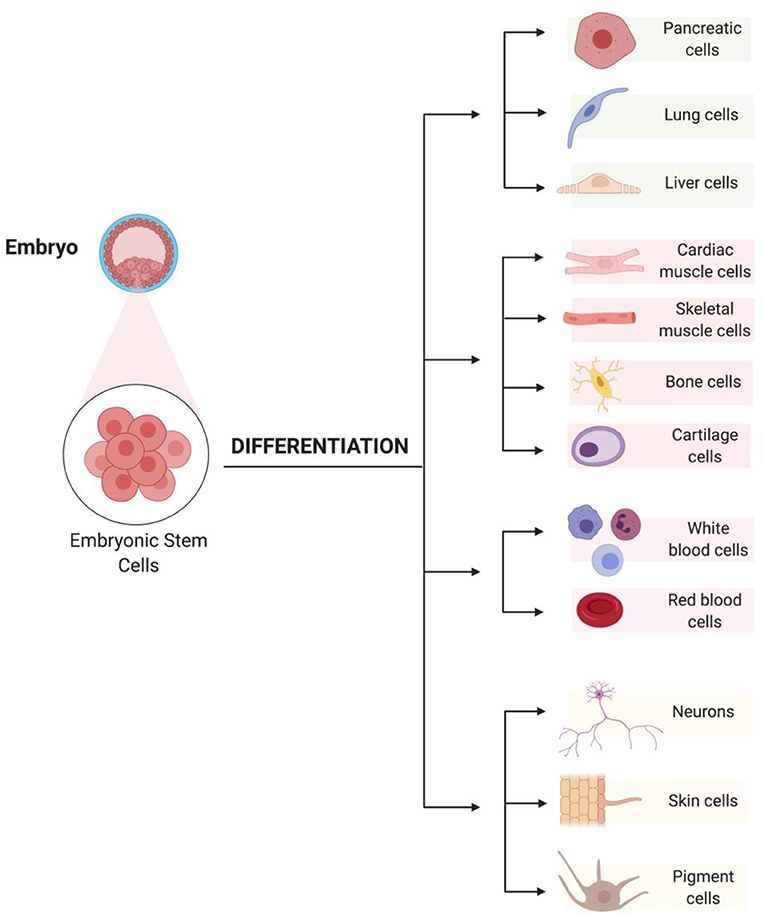
- Figure 2 - Embryonic stem cells can divide rapidly and can differentiate into any cell type in the body.
To create cardiomyocytes from stem cells in the lab, scientists expose stem cells to the same signaling molecules that produce cardiomyocytes in the developing embryo. After a few days, the developing cardiomyocytes have a heartbeat, which can be seen under the microscope (Video 1)! The stem cell-derived cardiomyocytes continue to grow and differentiate over the course of several weeks. The result is an almost endless supply of functional, beating heart muscle cells. Various molecular tests are performed to verify the identity and purity of laboratory-grown cardiomyocytes.
- Video 1 - Representative video of human induced pluripotent stem cell-derived cardiomyocytes spontaneously contracting in culture. The contraction observed in the video indicates the cells are alive and able to communicate that results in the observed movements.
Stem Cells To The Rescue!
To help patients recover from their heart attacks, scientists are using stem cell-derived cardiomyocytes to replace cardiac muscle in areas where the original heart muscle has turned into scar tissue. One method is called the cardiac patch, which is a laboratory-grown piece of heart tissue that can be implanted in the heart to replace the patient’s damaged tissue (Figure 3) [4]. A cardiac patch is like a special band-aid for a damaged heart. To create a cardiac patch, cells are grown on a scaffold, which is a template for tissue formation, under conditions that simulate those found in the body [5]. For example, the temperature, oxygen, and carbon dioxide levels found in the body are replicated. A combination of cells found in the heart are placed on the scaffold, along with stem cell-derived cardiomyocytes. Finally, the cardiac patch is implanted in the heart, in the hopes of replacing dead, non-beating scar tissue with functional, beating heart muscle.
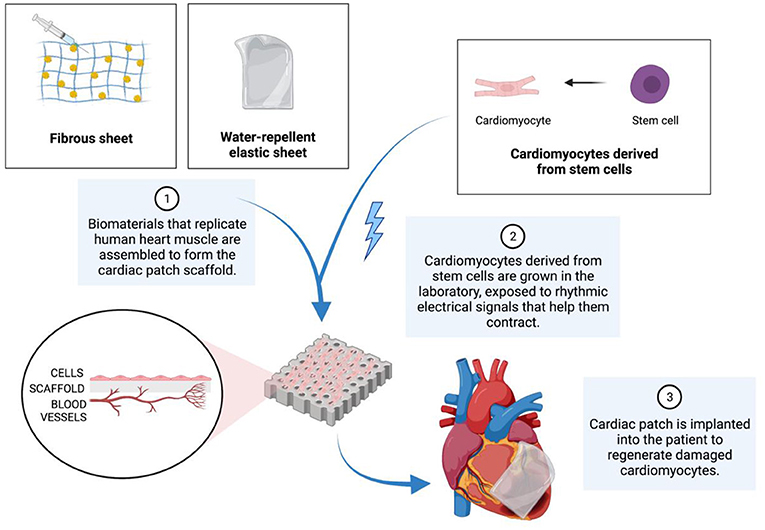
- Figure 3 - To produce cardiac patches, a scaffold must first be assembled.
- The scaffold is made from materials that replicate human heart muscle. Next, cardiomyocytes that have differentiated from stem cells are placed onto the scaffold. Eventually, the complete scaffold is implanted into a patient to help heal the damaged heart muscle.
Scientists have been investigating various methods to administer cardiac patches. They have found that exposing stem cell-derived cardiomyocytes to rhythmic electrical signals, essentially gently “electrocuting” them before implantation, improves their ability to contract. However, there are still many roadblocks to the use of cardiac patches in therapy. First, when a cardiac patch is implanted, only 0.1–10% of implanted cells survive to become functional cardiomyocytes [3]. This number is too low to fully restore heart function. It is also possible that some of the implanted cells may not be able to beat at the same time or perform the same function as the rest of the heart, which can disrupt heartbeat rhythm and efficiency [6].
New discoveries are constantly being made in the field of cardiac tissue regeneration. Although heart attacks are dangerous and deadly, we scientists can “attack” them using therapies like the cardiac patch. Using science, we can regrow heart tissue, restore heartbeats, and bring hope to patients with damaged hearts.
Stem Cells In A Wider Context
The opportunities possible because of stem cell therapies are boundless, extending far beyond the scope of cardiac regeneration. For example, stem cells have been used to replace brain cells that are damaged due to spinal cord injuries or diseases such as Alzheimer’s or Parkinson’s [7]. Another potential application of stem cells is using them to produce insulin, a hormone the body produces to regulate blood sugar levels. This can help treat patients with diabetes [8]. Scientists are not only continuing to explore and create medical ways to use stem cells to solve medical problems in the lab—they are also seeking out ways to use stem cell therapies to improve the health of patients with multiple diseases or disorders.
Glossary
Sinoatrial (SA) Node: ↑ A specialized structure in the heart that initiates heartbeats by signaling heart muscle cells to contract.
Cardiomyocytes: ↑ The heart’s muscle cells that contract to pump blood throughout the body.
Atherosclerosis: ↑ A disease caused by the thickening, stiffening, and narrowing of the arteries, resulting from buildup of plaque, fats, cholesterol and other substances along the inner artery linings.
Plaques: ↑ Deposits in the arteries composed of fat, cholesterol, and other substances in the blood that build up and harden over time, narrowing the arteries and blocking blood flow.
Stem Cells: ↑ Master cells that divide quickly and can become any cell type. Embryonic stem cells come from embryos and induced pluripotent stem cells come from adult tissues that have been genetically reprogrammed.
Pluripotent: ↑ Describes cells that can differentiate into any other cell type.
Cardiac Patch: ↑ A piece of heart muscle tissue, composed of cardiomyocytes and other cardiac cell types, grown on a scaffold in the lab and used to repair a damaged heart.
Scaffold: ↑ A framework or platform made of special materials that form a surface for cells to grow on, to create an engineered piece of tissue.
Conflict of Interest
The authors declare that the research was conducted in the absence of any commercial or financial relationships that could be construed as a potential conflict of interest.
Acknowledgments
The authors would like to thank the support of the Canadian funding agencies NSERC, CIHR, NFRF, and Heart and Stroke Foundation Canada. EA thank the Ministry of Economic Development, Job Creation and Trade for an Early Researcher Award as well the University of Ottawa Heart Institute. The authors would like to thank Dr. Wenbin Liang from the University of Ottawa Heart Institute for providing the iPSC cells displayed in the video.
References
[1] ↑ Ceconi, C., Guardigli, G., Rizzo, P., Francolini, G, and Ferrari, R. 2011. The heart rate story. Eur. Hear. J. Suppl. 13:C4–13. doi: 10.1093/eurheartj/sur014
[2] ↑ Tirziu, D., Giordano, F. J., and Simons, M. 2010. Cell Communications in the heart. Circulation 122:928. doi: 10.1161/CIRCULATIONAHA.108.847731
[3] ↑ Nguyen, P. K., Rhee, J.-W., and Wu, J. C. 2016. Adult stem cell therapy and heart failure, 2000 to 2016: a systematic review. JAMA Cardiol. 1:831–41. doi: 10.1001/jamacardio.2016.2225
[4] ↑ Hosoyama, K., Ahumada, M., McTiernan, C. D., Davis, D. R., Variola, F., Ruel, M., et al. 2018. Nanoengineered electroconductive collagen-based cardiac patch for infarcted myocardium repair. ACS Appl. Mater. Interfaces 10:44668–77. doi: 10.1021/acsami.8b18844
[5] ↑ Sedlakova, V., Ruel, M., and Suuronen, E. J. 2019. Therapeutic use of bioengineered materials for myocardial infarction. Nanoeng. Mater. Biomed. Uses 161–93. doi: 10.1007/978-3-030-31261-9_9
[6] ↑ Zhang, J., Zhu, W., Radisic, M., and Vunjak-Novakovic, G. 2018. Can we engineer a human cardiac patch for therapy? Circ. Res. 123:244–65. doi: 10.1161/CIRCRESAHA.118.311213
[7] ↑ Lee, C., Willerth, S. M., Nygaard, and H. B. 2020. The use of patient-derived induced pluripotent stem cells for Alzheimer’s disease modeling. Prog Neurobiol. 192:101804. doi: 10.1016/j.pneurobio.2020.101804
[8] ↑ Jiang, W., Shi, Y., Zhao, D., Chen, S., Yong, J., Zhang, J., et al. 2007. In vitro. derivation of functional insulin-producing cells from human embryonic stem cells. Cell Res. 17:333–44. doi: 10.1038/cr.2007.28