Abstract
You may have heard that coral reefs are overheating and are vanishing from our planet. Although this is certainly the case, the news is not all grim and gloomy! Every so often, surprising experiments show us that there is still hope for corals and much more to learn from them. Working along the Red Sea coast, we were surprised to find that corals living in very salty water seemed to be stronger during overheating periods. Moreover, we also found a link between the saltiness of water and the ability of anemones (the close cousins of corals) to deal with heat. In this article, we will explore this relationship by zooming into anemone cells to measure molecules invisible to the naked eye—all with the aim of figuring out how salt can make anemones and corals stronger in the face of increasing temperatures.
Hot Reefs In a Changing Climate
Corals are animals that make beautiful underwater gardens called coral reefs. These reefs are home to millions of other organisms, and some people call them our planet’s underwater rainforests. Unfortunately, just like real rainforests, coral reefs are in danger. Ocean warming, a result of climate change, is threatening corals everywhere. In other words, corals are overheating!
When corals start to feel the stress of overheating, which is called heat stress, they lose the vital partners that help them make such lush reefs possible. These partners are tiny plant-like cells called microalgae. Microalgae live inside the coral cells, supplying them with the food needed to grow and stay healthy. This relationship is a type of symbiosis, in which two different organisms live close together and generally benefit from each other. The tiny algae are often referred to as algal symbionts (Figure 1). When the algal symbionts are expelled from the coral’s tissue due to heat stress, the coral loses its main food supply. This event is called coral bleaching because the corals become white due to the loss of their algal symbionts. Without food from the symbionts, corals may eventually starve and die if heat stress continues.
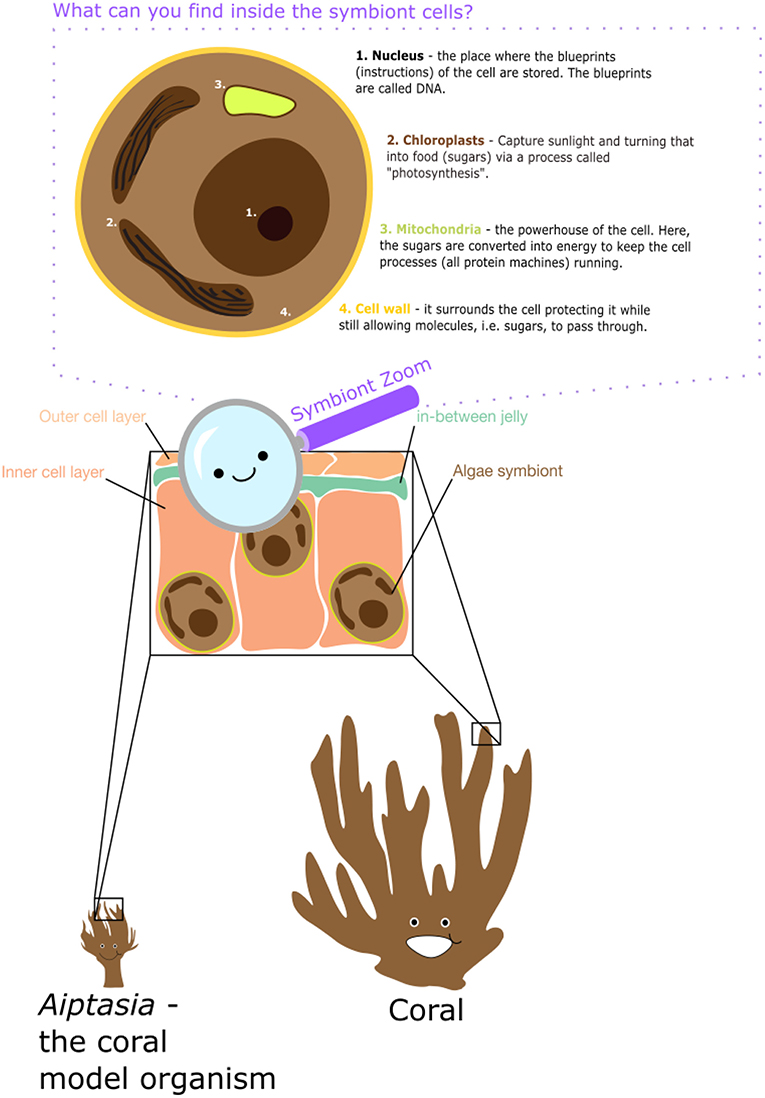
- Figure 1 - A cell within a cell.
- In addition to all the normal parts of a cell, coral and anemone cells contain an additional cell—an algae cell. Corals and anemones live in symbiosis with their algal symbionts by exchanging nutrients they both need to survive. The symbiont cell can produce a lot of sugars using its chloroplasts. The symbiont gives some of the excess sugars to the coral or anemone cell in exchange for other important nutrients it cannot produce with any of its other cell compartments.
Saltier Anemones are Stronger During Heat Stress
In a previous Frontiers for Young Minds article, we described an experiment in which we used the sea anemone Aiptasia—a close relative of corals with the same algal symbionts—to learn more about coral bleaching. We were interested in the effects of salinity, the salt content of water, on bleaching. To our surprise, we discovered that anemones in high-salinity seawater are stronger during heat stress. Anemones that lived in saltier seawater bleached less! They kept more of their algal symbionts, which continued to supply the anemone with food. We measured this by counting the algal symbionts inside the anemones [1]. While this was an interesting experiment, we were left wondering why our salty anemones kept their algal symbionts, while anemones growing at a lower salinity did not.
Our main hypothesis was that anemones in high-salinity conditions adjusted their cells to the higher salt content. To do so, the anemones or their algal symbionts must produce something that either helps them handle saltier water or makes them more resistant to heat stress. Moreover, we knew from other experiments that, during heat stress, dangerous molecules called reactive oxygen species (ROS), are produced, which contribute to coral bleaching [2, 3]. ROS can damage the cells of anemones/corals and their algal symbionts. Therefore, we thought that, in high-salinity conditions, maybe there are some molecules present that can protect anemones and their algal symbionts from ROS. To find molecules produced in high-salinity water that could counter the damaging ROS, we repeated our previous experiment—but we analyzed the molecules produced inside the cells of the anemones and their algal symbionts.
Sugar, the Fuel of Life
All cells are tiny, closed compartments filled with factories, storage rooms, building blocks, and miniscule molecular “machines.” And, just like a machine, the cell needs energy to function properly. Energy is a precious resource for cells. It is created by one of the most important cellular structures, the powerhouses of the cell, called mitochondria. Inside mitochondria, the sugars from the foods we eat are transformed into energy for the cellular machinery. In addition to mitochondria, algal symbionts have special structures, called chloroplasts, that generate food by producing energy-rich sugars from sunlight! This process is known as photosynthesis—the same process your garden plants use to produce their own food!
Since the anemones and corals do not have chloroplasts, they cannot perform photosynthesis to produce sugars from sunlight. However, if they live together with their algal symbionts, they can receive these sugars from those photosynthesizing algal cells. While some of the sugars are used by the algal symbionts to keep their own machinery running, a big portion is shared with the anemone or coral host! What a deal!
Heat: An Enemy of Sugar Sharing
Unfortunately, during heat stress, the symbiont’s cell machinery gets damaged. This stops photosynthesis and the sugar sharing between algal symbiont and coral. This damage is done by the accumulation of the ROS molecules we mentioned earlier—they can be imagined as tiny “sparks” generated during the energy-production processes. If too many of these sparks are generated, or if they are generated in an uncontrolled way, they can “set fire” to parts of the algal symbionts, destroying them [4]. This may result in bleaching. The details of coral bleaching, the role of ROS, and the ending of photosynthesis by algal symbionts are all complex topics that are still being researched!
More Salt Leads to More Sugars: Avoiding Osmotic Shock
You may be wondering why are we talking about sugars when salt is the factor that protects anemones from bleaching during heat stress, right? The reason is that sugar is not only used for energy! There are many forms of sugars and sugar-containing molecules within cells. Like many other molecules, sugars help cells handle the salinity of the water around them. Sugars influence the natural process of osmosis by acting as osmolytes. Osmolytes are substances that can either be produced or broken down in the cell to help the cell adjust to the surrounding salinity. If osmolytes are not adjusted rapidly, the cell enters a state called osmotic shock, which can damage it. Osmolytes are therefore lifesaving for certain cells.
Anemones use some sugars as an energy resource, but also use sugars as osmolytes to keep up with the high salinity around them. Since the algal symbionts are inside the anemone cells, they too must adjust to the salinity. This salt-sugar link connects the salty environment to the changes inside the anemone cells as well as their algal symbionts.
In our experiment, we put anemones under heat stress at low, medium, and high salinity. Then, we looked to see if any osmolyte sugars were changing, to get an idea of what was going on inside the algae cells. In doing so, we found a sugar-containing molecule that stood out: floridoside.
The Many Faces of Floridoside
Floridoside is a sugar-containing molecule and a known osmolyte. We found high amounts of floridoside in the algae cells of anemones that were kept in high-salinity water. By building up more floridoside, the algal symbionts adjusted to the new salinity and did not enter into osmotic shock, avoiding damage!
Interestingly, floridoside lives a double life! It can also act as a ROS neutralizer! In other words, floridoside puts out the “sparks” that are produced during energy production. By preventing ROS from building up in the algal symbionts, floridoside prevents the cell damage that stops photosynthesis and the sharing of sugars between anemone and algal symbiont and that can lead to coral bleaching.
To detect floridoside’s ROS neutralizing action, we measured the amount of ROS produced by the algal symbionts during heat stress at different salinities. We observed that the more floridoside that was detected in the algal symbiont, the less ROS were present. This relationship between more floridoside and fewer ROS may explain why anemones bleach less in higher salinity (Figure 2). Floridoside, acting as an osmolyte and ROS neutralizer, may protect the machinery of the algal symbionts and ensure the continued supply of sugars to the anemone. More food for the anemone means more energy, which in turn means more resources to fight against other stressors such as heat stress. This may result in a stronger symbiosis or, in other words, less bleaching!
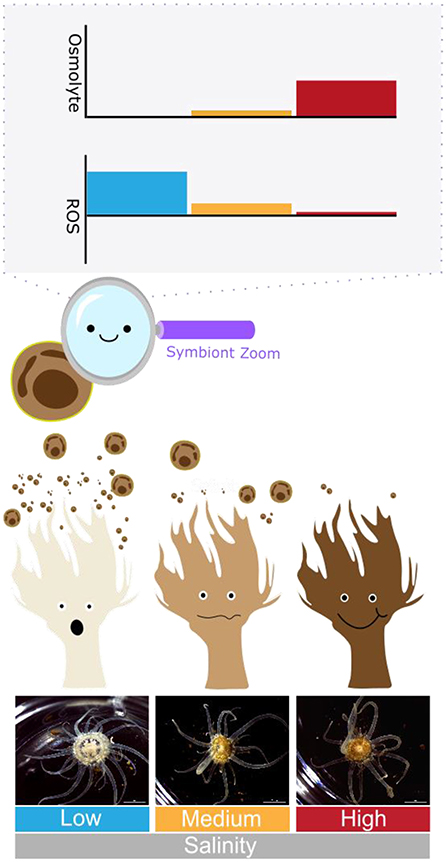
- Figure 2 - The saltier the better.
- At the bottom, you can see anemones in low (blue), medium (yellow), and high (red) salinity levels during heat stress. The one with the most algae inside is the darkest—notice this anemone is in the highest salinity, while the anemone in the lowest salinity is completely bleached. The level of the osmolyte floridoside and the levels of ROS were measured in each of these anemones’ algal symbionts. In the lowest salinity, we saw low floridoside and high ROS during heat stress. In the highest salinity, we saw high floridoside and low ROS during heat stress. This suggests that a higher salinity is linked to high levels of floridoside. High levels of floridoside seem to protect the algal symbiont from the thermal stress by potentially neutralizing ROS molecules and preventing bleaching.
In A Nutshell
The salinity of water changes the way cells behave and which substances they produce. This means that salinity has a big influence on the entire animal. High salinity causes anemone cells and their algal symbionts to adjust their levels of osmolytes, such as floridoside. They produce more osmolytes the saltier the water gets. Floridoside is a sugar-containing osmolyte that also neutralizes the ROS that can damage algal symbionts. This link between salinity, heat stress, and bleaching can help explain why anemones and some coral species bleach less during heat stress when living in saltier water, like the ones living in the Red Sea.
As usual in science, this is not the end of the story. There are many molecules that can act as osmolytes, and not all anemones and corals rely on the same ones. They may use different osmolytes to adjust to salinity changes. Also, not all anemones and only a few corals tested show this salinity effect! This opens a lot of new questions for future experiments.
Glossary
Algal Symbiont: ↑ Microscopic single-celled organisms that live in symbiosis with a coral or anemone cell by producing sugars through photosynthesis, like plants, and sharing them with their host.
Coral Bleaching: ↑ When organisms such as corals or anemones, that live in symbiosis with algal symbionts, become white due to the loss of their algal symbionts.
Salinity: ↑ The amount of salt in water. You can find different salinities in oceans or seas depending on their location. The Red Sea is one of the saltiest seas worldwide.
Reactive Oxygen Species (ROS): ↑ Molecules that are generated during the production of energy, a reaction inside every cell that involves oxygen.
Osmosis: ↑ Movement of water through membranes. Water always moves toward the side of the membrane that has more dissolved substances, like salt or sugar.
Osmolytes: ↑ Molecules that are produced, broken down, or transported into the cell to balance the level of dissolved substances, like salt and sugar, between the cell and the surrounding water.
Osmotic Shock: ↑ Sudden change in dissolved substances around a cell, which makes water move in or out rapidly, causing damage to the cell.
Floridoside: ↑ A sugar-containing molecule produced inside algal symbionts that can help them adjust to different salinities and to neutralize harmful molecules that arise from energy production.
Conflict of Interest
The authors declare that the research was conducted in the absence of any commercial or financial relationships that could be construed as a potential conflict of interest.
Original Source Article
↑Gegner, H. M., Rädecker, N., Ochsenkühn, M., Barreto, M. M., Ziegler, M., Reichert, J., et al. 2019. High levels of floridoside at high salinity link osmoadaptation with bleaching susceptibility in the cnidarian-algal endosymbiosis. Biol. Open 8:bio045591. doi: 10.1242/bio.045591
References
[1] ↑ Gegner, H. M., Ziegler, M., Rädecker, N., Buitrago-López, C., Aranda, M., and Voolstra, C. R. 2017. High salinity conveys thermotolerance in the coral model Aiptasia. Biol. Open 6:1943–8. doi: 10.1242/bio.028878
[2] ↑ Weis, V. M. 2008. Cellular mechanisms of Cnidarian bleaching: stress causes the collapse of symbiosis. J. Exp. Biol. 211:3059–66. doi: 10.1242/jeb.009597
[3] ↑ Lesser, M. P. 2006. Oxidative stress in marine environments: biochemistry and physiological ecology. Annu. Rev. Physiol. 68:253–78. doi: 10.1146/annurev.physiol.68.040104.110001
[4] ↑ Szabó, M., Larkum, A. W. D., and Vass, I. 2020. “A review: the role of reactive oxygen species in mass coral bleaching,” in Photosynthesis in Algae: Biochemical and Physiological Mechanisms, eds A. W. D. Larkum, A. R. Grossman, and J. A. Raven (Cham: Springer International Publishing). p. 459–88. doi: 10.1007/978-3-030-33397-3_17