Abstract
If you walk on the beach, you may observe many kinds of stones of diverse colors. Surprisingly, some of these “stones” may be algae! A certain type of red algae creates structures called redstones, also known as rhodoliths. Rhodoliths are important builders and can create extensive banks at the bottom of the oceans. These bioengineers create an oasis for marine life. Marine worms, crabs, and sea stars, for example, can live in and on rhodoliths. However, human activities, including pollution and removal of rhodoliths for aquarium decoration, may harm rhodolith banks. Rhodoliths are sensitive to disturbances and should be protected. In this article, we explain the main attributes of rhodoliths, including their formation, distribution, and importance, as well as conservation measures, we can take. We hope that what you learn about rhodoliths will inspire you to defend these fascinating “rock-and-rolling” ocean creatures.
Mysterious Redstones: “Living Rocks”
Perhaps, while walking on the beach, snorkeling, or diving, you have seen stones of various colors. You may have even seen a redstone, but you probably did not recognize its importance at the time, because redstones look just like any other stone in the sea—but they are not! While redstones may look like stones, gravel, or rocks, they are in fact made of living and dead algae [1]. The algae that makeup redstones belong to the group Rhodophyta (red algae). Redstones have a scientific name: rhodoliths! This means stones with a reddish color that roll on the ocean bottom. Rhodolith “stones” are composed of at least 50% red algae.
Rhodoliths live in all oceans: the Pacific Ocean (Southern Japan), near Southern Australia, in the Gulf of California, the Mediterranean Sea, the North Atlantic Ocean (from Norway to Portugal), and the Caribbean Sea. There are many rhodoliths in the South Atlantic Ocean, particularly in the Abrolhos Archipelago in Northeast Brazil [2]. They can be present from the intertidal beach, where we walk, to the open sea, up to 270 meters in depth [1]. Rhodoliths have lived in the seas since the Cretaceous period, 145–66 million years ago [1]. This is the same time that dinosaurs lived on our planet!
Although our “rock-and-rolling” friends are not as famous as dinosaurs, rhodoliths play essential roles in marine ecosystems! Algae produce oxygen, making it available for other marine life. Rhodoliths are also bioengineers, meaning that they build structures that serve as habitats and food sources for many sea creatures.
Hopefully, after reading this article, you will understand the importance of these amazing marine “houses” and be motivated to help us preserve them!
How Are Rhodoliths Formed?
The algae that form rhodoliths are called calcareous algae because they use a substance called calcium carbonate (CaCO3) to grow and form banks on the ocean bottom [3]. How do they do this? Well, calcium carbonate is composed of two substances: calcium and carbonate. Calcium (Ca2+) is naturally present in seawater. Carbonate () is created when carbon dioxide (CO2) gas from the atmosphere contacts seawater and undergoes some chemical reactions to create calcium carbonate. Then, corals, molluscs, calcareous algae, and other ocean organisms can take up calcium carbonate to build their skeletons, in a process called calcium carbonate assimilation. The breakdown of rock formations in the ocean may also add calcium carbonate to the seawater. When organisms that have skeletons built of calcium carbonate die, the calcium carbonate returns to the water to be used again. By this process, dying rhodoliths are one of the main sources of calcium carbonate in the oceans (Figure 1).
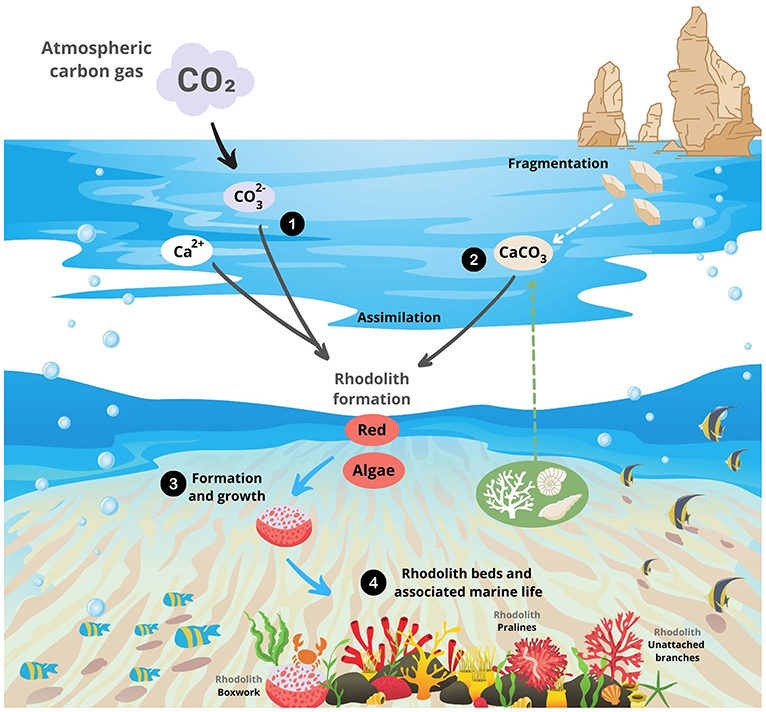
- Figure 1 - (1) Calcium (Ca2+), found in ocean water, and carbonate (), created when CO2 from the atmosphere undergoes chemical reactions, combine to form calcium carbonate (CaCO3).
- (2) Other sources of CaCO3 include dead shells, corals, other algae, and fragments of broken-down rocks. (3) Rhodoliths form and grow by assimilating (CaCO3) into their skeletons. (4) Rhodolith banks on the ocean bottom consist of three rhodolith types: boxwork, pralines, and unattached branches. These banks serve as habitat for other ocean creatures.
Rhodoliths Have a Variety of Forms
Rhodoliths may grow into different forms [1]. In contrast to coral reefs, they are not attached to the seabed and may “rock and roll” underwater with the ocean currents. When they get too heavy, rhodoliths settle to the bottom and establish extensive banks, which provide important habitats for other sea creatures.
There are three types of rhodoliths: boxwork, unattached branches, and pralines (Figures 1, 2A) [4]. The boxwork type has an internal structure called a nucleus, which can be a small pebble or the remnants of another living creature, such as a piece of coral skeleton or shell. From this nucleus, the rhodolith grows into a compact shape. Unattached branches do not have a nucleus and have several tree branch-like structures. Finally, pralines also have a nucleus, upon which multiple bumps develop. All three types of rhodoliths grow by adding overlapping layers of calcium carbonate (Figure 2B).
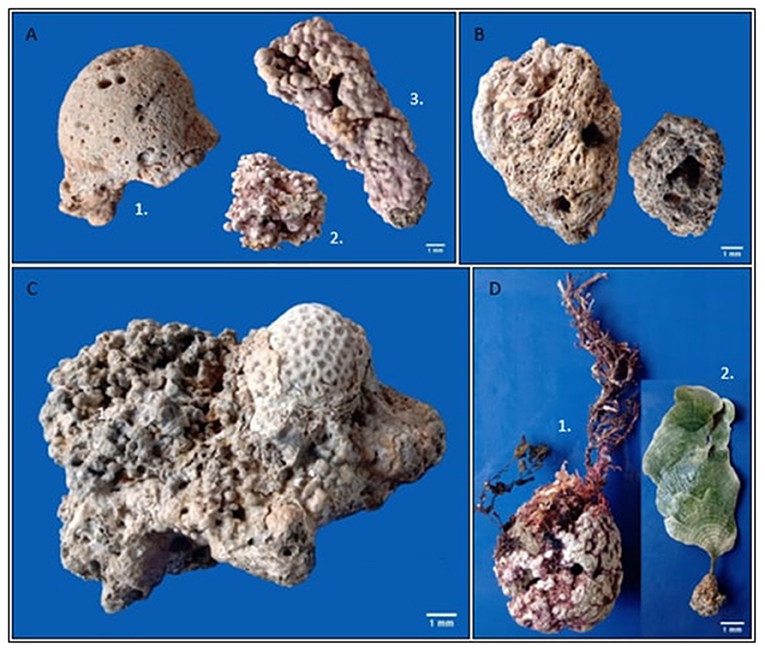
- Figure 2 - Examples of rhodoliths.
- (A) Rhodolith types: 1. Boxwork; 2. Unattached branches; 3. Praline. (B) Internal part of rhodoliths, with the overlapping layering (remarkably on the right photo). (C) Rhodolith associated with the starlet coral Siderastrea stellata, seen on the top right. (D) Algae growing on the rhodolith surface: 1. red algae; 2. calcareous green algae (Photographs’ credit: K. Massei).
Many factors may influence rhodolith formation and growth, including the movement of ocean water, the amount of light present, and calcium carbonate availability. Ocean water movement influences their shape: rhodoliths get “rounder” as they roll with the water. The less water movement, the more branches the rhodoliths will have [5]. Light is essential for rhodolith growth because algae need light for photosynthesis [6]. Calcium carbonate in seawater is essential because calcareous algae need this substance for their growth.
As rhodoliths develop, other organisms may stick to their surfaces. The tropical starlet corals are an example because they take advantage of the shelter and attachment surface provided by the rhodolith, making the rhodolith its home (Figure 2C). Other kinds of algae may also attach and grow on rhodoliths (Figure 2D).
Importance of Rhodolith Banks
A tropical rhodolith bank can reach a diameter similar to that of a soccer ball, but these banks grow slowly: only 1 to 1.5 mm per year [2]. They also have a long life span (>100 years). These “elders of the sea” are potential indicators of environmental changes that have happened over time.
Rhodolith banks provide several essential benefits. In addition to providing habitats for other creatures and promoting local biodiversity, rhodolith banks can also protect the coasts against the erosive action of waves. They also have important benefits for the local climate. Too many free carbonates () in the oceans can be harmful. The chemical reactions that generate carbonates also produce hydrogen ions (H+), which increases the seawater acidity. This is the cause of ocean acidification, which is dangerous because it can cause the death of ocean life, including our rock-and-rolling friends. Calcareous organisms like rhodoliths are essential for “storing” carbonates in their skeletons. This process turns carbonates into non-harmful forms and helps to prevent ocean acidification (Figure 3). However, this equilibrium is fragile, and human activities disrupt it [7].
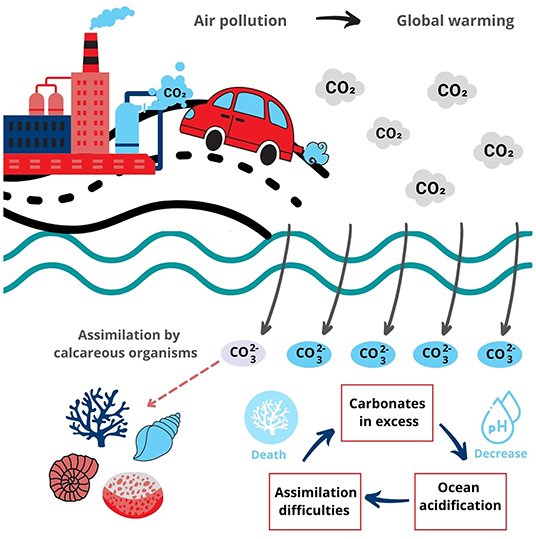
- Figure 3 - When there is too much carbon dioxide (CO2) in the air from air pollution, then too much carbon dioxide will be dissolved in the ocean which increases the acidity of the ocean.
- Ocean acidification makes it difficult for calcareous creatures—like rhodoliths, corals, snails, or clams—to absorb carbonate as they grow and can even result in their death in extreme cases.
Another climate-related benefit of rhodoliths is that the algae “trap” carbon dioxide (CO2) from the atmosphere during photosynthesis. Carbon dioxide is naturally present in the atmosphere and living organisms release it when they breathe. However, over the last two centuries, the carbon dioxide concentration has increased considerably due to the burning of fossil fuels. When there is too much carbon dioxide in the atmosphere, it can contribute to global warming and climate changes. So, when rhodolith beds capture and trap carbon, they help to lower atmospheric carbon dioxide levels (Figure 3). The carbon stored in marine ecosystems is known as blue carbon [8].
Humans and Rhodoliths
For humans, rhodoliths are important sources of marine limestone, which has several uses. It can be used in agriculture, to lower the soil’s acidity; in the cosmetic industry, to produce toothpaste and bath salts; and in the food industry, as a food supplement. Rhodoliths can also be used to create bone implants that are compatible with human tissues. Finally, rhodoliths can be used in aquariums, as decoration and as living water filters.
While direct harvesting of rhodoliths can obviously damage them, other human actions may also affect rhodoliths and the life forms linked to them. One example is inappropriate waste disposal at sea, causing for example contamination of seawater (organic pollution), increased amount of plastics (plastic pollution), and other materials that are harmful to the ocean balance. Uncontrolled tourism can result in the trampling of rhodoliths that live on coastlines. Among the most damaging human actions are dredging and bottom trawling, directly removing the bottom-dwelling creatures, such as rhodoliths. Drilling for oil and gas are also damaging, and the disposal of waste material produced by drilling can bury entire communities of rhodoliths.
Help Us Preserve Rhodoliths!
We hope you now better understand the importance of our “rock-and-rolling” friends, the rhodoliths! There are still many things we do not know about them, so more research on rhodoliths is needed to better understand their role in regulating Earth’s climate and how we can continue to harvest them for important purposes while also conserving their populations. We must be especially careful because they recover so slowly: thousands of years are required to form an extensive rhodolith bank! Protecting rhodoliths is urgent because the destruction of their banks would be catastrophic. Imagine everything else that might be negatively affected: marine species that use rhodoliths as habitat, coastal lands that they protect from erosion, and even our climate, which rhodoliths help to keep in balance the best they can.
So, what can you do to help preserve rhodoliths? Knowing about them—what they are, where they are found, their importance, and the negative impacts of their destruction—is the best way to start protecting them. Now that you know these things about rhodoliths and their benefits for the environment, you can raise other people’s awareness of them [9]. If more people understand these organisms, we will be in a better position to create the needed strategies and policies for their conservation. The more people who understand these fascinating, mysterious, “living rocks,” the greater our power to preserve them!
Funding
This study was financed in part by the Coordination for the Improvement of Higher Education Personnel (CAPES, Brazil)—Finance Code 001. In partnership with Interdisciplinary Center of Marine and Environmental Research (CIIMAR, Portugal), this research was supported by funds through Foundation for Science and Technology (FCT, Portugal) within the scope of UIDB/04423/2020 and UIDP/04423/2020. DA was supported by a Ph.D. scholarship provided by Doctoral Programme Sandwich Abroad (PDSE)/CAPES (Edital no 47/2017), in partnership with Fundação de Apoio à Pesquisa do Estado da Paraíba (FAPESQ) (Edital no 03/2016). KM was supported by a Ph.D. scholarship provided by Fundação de Apoio à Pesquisa do Estado da Paraíba (FAPESQ) (Edital no 03/2016). MD was supported by the FCT Researcher programme contract (MD, IF/00919/2015), subsidized by the European Social Fund and Portuguese Ministry of Science, Technology and Higher Education (MCTES), through the Human Potential Operational Programme (POPH). MC thanks the productivity grant provided by the National Council for Scientific and Technological Development (CNPq, Brazil).
Glossary
Algae: ↑ Also known as seaweed, representing marine creatures that produce their own food through a process of light capture (photosynthesis), similar to terrestrial plants. Examples: kelps, rhodoliths, rockweed, sea lettuce.
Rhodoliths: ↑ Unattached calcareous reddish stones, composed of at least 50% of a red alga type, that roll on the ocean bottom, establishing extensive banks, as a set of marine houses.
Bioengineers: ↑ These are the creatures that are able to carry out constructions or modifications in the environment where they live, for example by building their own houses or making small paths.
Calcareous: ↑ Anything that is formed by calcium carbonate, for example, shells, pearl, coastal cliffs, corals, limestones, and some algae (such as rhodoliths).
Calcium Carbonate: ↑ It corresponds to a substance formed by calcium (Ca2+) and carbonate (constituted by carbon and oxygen: ), constructing the chemical formula CaCO3, which forms the calcareous materials.
Assimilation: ↑ In nature, involves the acquisition of a certain substances by living beings with the aim of using it for their own benefit, for example for food or protection.
Ocean Acidification: ↑ Phenomenon characterized by increased acidity (pH decrease) in seawater due to the carbonates spread, caused essentially by human action, with the burning of petroleum products, such as coal and oil.
Blue Carbon: ↑ Carbon is a component widely found in nature, constituting from graphite and diamond to petroleum. When this substance is stably stored in the ocean it is called blue carbon.
Conflict of Interest
The authors declare that the research was conducted in the absence of any commercial or financial relationships that could be construed as a potential conflict of interest.
Acknowledgments
We thank the academic support provided by Programa Regional de Pós-Graduação em Desenvolvimento e Meio Ambiente (PRODEMA), Federal University of Paraíba (UFPB), and Aquário Paraíba, Brazil.
References
[1] ↑ Riosmena-Rodríguez, R., Nelson, W., and Aguirre, J. 2017. Rhodolith/maërl Beds: A Global Perspective, eds. R. Riosmena-Rodríguez, W. Nelson, J. Aguirre (Cham: Springer International Publishing). doi: 10.1007/978-3-319-29315-8
[2] ↑ Amado-Filho, G. M., Moura, R. L., Bastos, A. C., Salgado, L. T., Sumida, P. Y., Guth, A. Z., et al. 2012. Rhodolith beds are major CaCO3 bio-factories in the tropical South West Atlantic. PLoS ONE. 7:e35171. doi: 10.1371/journal.pone.0035171
[3] ↑ Teed, L., Bélanger, D., Gagnon, P., and Edinger, E. 2020. Calcium carbonate (CaCO3) production of a subpolar rhodolith bed: methods of estimation, effect of bioturbators, and global comparisons. Estuar. Coast Shelf Sci. 242:106822. doi: 10.1016/j.ecss.2020.106822
[4] ↑ Sañé, E., Chiocci, F. L., Basso, D., and Martorelli, E. 2016. Environmental factors controlling the distribution of rhodoliths: an integrated study based on seafloor sampling, ROV and side scan sonar data, offshore the W-Pontine archipelago. Cont. Shelf Res. 129:10–22. doi: 10.1016/j.csr.2016.09.003
[5] ↑ Melbourne, L. A., Denny, M. W., Harniman, R. L., Rayfield, E. J., and Schmidt, D. N. The importance of wave exposure on the structural integrity of rhodoliths. J. Exp. Mar. Bio. Ecol. (2018) 503:109–119. doi: 10.1016/j.jembe.2017.11.007
[6] ↑ Carvalho, V. F., Assis, J., Serrão, E. A., Nunes, J. M., Anderson, A. B., Batista, M. B., et al. 2020. Environmental drivers of rhodolith beds and epiphytes community along the South Western Atlantic coast. Mar. Environ. Res. 154:104827. doi: 10.1016/j.marenvres.2019.104827
[7] ↑ Riosmena-Rodríguez, R. 2017. “Natural history of rhodolith/maërl beds: their role in near-shore biodiversity and management,” in Rhodolith/maërl Beds: A Global Perspective, eds R. Riosmena-Rodríguez, W. Nelson, J. Aguirre (Cham: Springer International Publishing). p. 3–26. doi: 10.1007/978-3-319-29315-8_1
[8] ↑ Macreadie, P. I., Anton, A., Raven, J. A., Beaumont, N., Connolly, R. M., Friess, D. A., et al. 2019. The future of blue carbon science. Nat. Commun. 10:3998. doi: 10.1038/s41467-019-11693-w
[9] ↑ Costa, D. A., de Lucena, R. F. P., Christoffersen, M. L., Piñeiro-Corbeira, C., and Dolbeth, M. 2021. Improving environmental awareness and ocean literacy through hands-on activities in the tropics. Appl. Environ. Educ. Commun. 1–20. doi: 10.1080/1533015X.2021.1887778