Abstract
All around the world, beneath the seafloor, there are huge volumes of natural gas. But these are not the normal gas reservoirs that we collect to use for cooking, heating our homes, and making electricity in power stations. This gas is locked up in what we call gas hydrates. Gas hydrates are a solid form of water, rather like ice, that contains gas molecules locked up in a “cage” of water molecules. Gas hydrates are found on continental shelves around the world and in permafrost in the arctic. We are interested in gas hydrates because they could be used as a future source of natural gas. They are also important because they can cause large landslides on the seafloor, damaging offshore pipelines and cables and contributing to the formation of tsunami waves.
What Are Gas Hydrates?
Gas hydrates are a solid form of water, similar to ice, which contain gas molecules locked up in a “cage” of water molecules (Figures 1a,b) [1]. Methane is the most common gas found in gas hydrates and it is also the natural gas we use as a fuel source. We use natural gas to heat our homes, cook on gas stoves, and generate electricity in power stations. Gas hydrates are nicknamed “fiery ice” because you can set fire to them and the gas trapped inside will burn (Figure 1c).
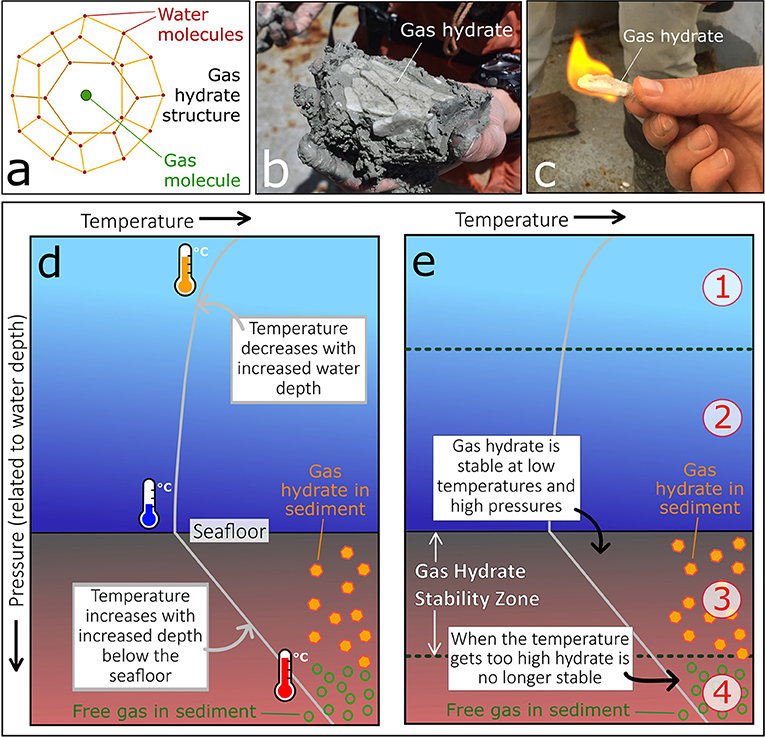
- Figure 1 - (a) The structure of a gas hydrate molecule.
- (b) Thin layer of white gas hydrate collected in a sediment core from beneath the seafloor. (c) A scientist holds a small piece of gas hydrate that is on fire. (d) Water temperature (gray line) decreases as the ocean water gets deeper, and then increases again beneath the seafloor. (e) Gas hydrates are only stable at high pressures and low temperatures, so stability is controlled by the temperature (gray line), and the depth beneath the sea surface. In zone 1, the pressure is too low, so hydrates are not stable; in zone 2, there is too much water and not enough gas to form hydrate; in zone 3, the conditions are perfect for hydrates to form, so they are stable; and in zone 4, it gets too hot, so hydrates cannot form.
Where and How Do We Find Gas Hydrates?
Gas hydrates are similar to ice, because they are stable at certain temperatures and pressures (Figures 1d,e). If you take ice out of the freezer it melts and becomes water, because it is only stable at very low temperatures. Gas hydrates are stable at low temperatures (2–20°C) and high pressures. This means we find them underwater on continental shelves and slopes, and in permafrost on land. Continental shelves and slopes are shallow underwater regions that surround most of the land masses on Earth. The water in these regions is around 100–2,000 m deep (Figure 2). Permafrost regions are where thick layers of soil remain below freezing temperature throughout the year, and they are found in the Arctic where the temperature of the ground is very low.
![Figure 2 - Locations where we have identified gas hydrates using seismic or well data (red circles), and where we have collected gas hydrates from samples (blue circles) [1, 3].](https://www.frontiersin.org/files/Articles/457058/frym-07-00096-HTML-r1/image_m/figure-2.jpg)
There are several different ways that we can find gas hydrates in the sediments under the ocean. The most commonly used technique for finding gas hydrates is collecting seismic data. Seismic data uses sound waves to create an image of the sediments beneath the ground (Figure 3a) [2]. It can be used on land and at sea, but here we will talk about collecting seismic data at sea. To collect seismic data at sea, a ship travels along towing a sound source (like a large speaker) and a series of hydrophones, which listen and record the sound waves that are reflected off the seafloor and the sediment layers under the seafloor. The sound waves reflect, or bounce back, like an echo when you shout in a big empty room. We can use the reflected sound waves to build up an image of the layers of sediment below the seafloor, by measuring how long it takes for the sound waves to travel down, be reflected, and travel back to the hydrophones. Depending on the type of sediments being imaged, we can gather data from as deep as several kilometers beneath the seafloor [2]. The sound waves will reflect off any surface where there is a change in the density of the sediment. So, if you have a muddy layer sitting on top of a sandy layer, the sound waves will reflect off the contact between the two layers. We can use seismic data to find gas hydrates because they slightly alter the density of the sediment in which they are found. They are also frequently found with gas bubbles trapped underneath the layer of hydrate in the sediment. Gas bubbles change the density of the sediment quite a lot, so they are easy to see in the seismic data. In the data, we see this change from hydrate to gas bubbles in the sediment as a line, which is called a bottom simulating reflection or BSR [1] (Figure 3a). It is called “bottom simulating” because it will roughly follow the depth of the seafloor above it. This is because the water depth controls the pressure and temperature, and therefore controls where the hydrates are stable [1] (Figure 1e).
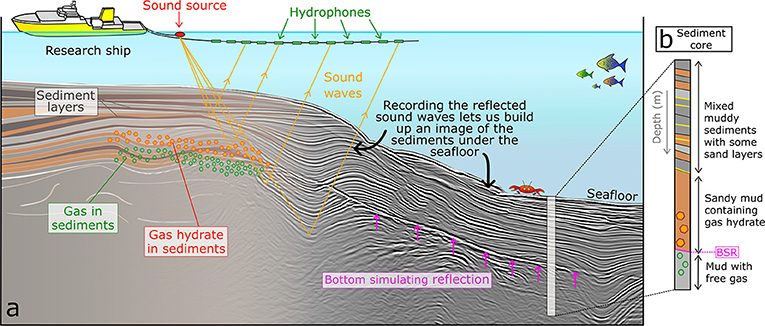
- Figure 3 - (a) A research ship acquiring seismic data using a sound source and hydrophones towed behind the ship.
- The sound waves reflect off the seafloor and layers of sediment beneath the seafloor, which builds up an image of the layers of sediment. (b) Sediment cores help us see what makes up the layers beneath the seafloor.
Sometimes we collect sediment samples to see if they contain gas hydrates, but this is not very easy when the sediments we want to sample are in very deep water. To do this, we need to use a ship with specialized equipment on board that can be lowered down to the seafloor to collect what is called a sediment core (Figures 1c, 3b). This is like taking a hollow straw and sticking it into a layered cake. When you pull the straw out, it will have a “sample” of the cake stuck in it, and you can tell what the different layers are made of if you look at this sample. Collecting a sediment core takes a lot of time, and it only tells us whether there are gas hydrates in a very small area, because the cores are usually only about 10 cm wide and a few meters long.
We can also identify gas hydrates using a technique known as well logging. When a well is drilled into the seafloor, instruments can be lowered down into the hole to measure and record various properties of the sediments and rocks beneath the seafloor. One of the commonly used instruments measures the resistivity of the sediments. This measurement tells us whether the sediments can conduct electricity or not, which tells us what is in the tiny spaces between the grains of sediment. If there is gas hydrate in the spaces, the resistivity measurement will be very high, because gas hydrates do not conduct electricity well. If there is water in the pore spaces, the resistivity measurement will be low, because water conducts electricity quite well. This technique is useful but can only be used where there is a well and like the core sample, it only provides information about a small area around the well.
Why Are We Interested in Gas Hydrates?
Gas hydrates are interesting because they might be a very useful source of natural gas in the future, but we also study them because they are possibly dangerous. There is far more methane in gas hydrates around the world than there is in normal oil and gas reservoirs [1]. But it is not as easy to get the gas out of gas hydrates as it is from normal gas reservoirs. To get the gas out of a gas hydrate reservoir, we need to either heat up the sediment, or reduce the pressure. This is quite difficult to do, and at the moment we do not produce gas from gas hydrates at any large scale, although some countries, such as Japan, have tested this idea [4].
Gas hydrates are also a possible hazard, because they can result in landslides at the seafloor. This is because hydrates change the way the sediment behaves and can make that sediment unstable [5]. Gas hydrates can act like a cement and hold the sediment grains together, which makes it stronger. But if the gas hydrate then becomes unstable because the sea level changes or the temperature rises, the sediment loses its cement and it will be weak and more likely to move, causing a landslide. If the gas hydrate becomes unstable, a large amount of gas will be released into the sediment, which makes it even more unstable as the gas expands and pushes the sediment around it. Depending on where these landslides happen and how big they are, they might cause tsunamis. Tsunamis are huge waves that can travel very long distances and cause a lot of damage and flooding when they hit land. Even small landslides can be a problem, because they can damage anything that is on the seafloor nearby, such as pipelines and cables.
What Else Do We Need to Know?
There is still a lot that we do not fully understand about gas hydrates. One of the big questions is exactly how much gas there is in gas hydrate reservoirs around the world. At the moment, it is difficult to measure this accurately—we can only estimate. Scientists are trying to answer this question using new technologies and different ways of looking at data. We also do not know how gas hydrates will react if the ocean temperature continues to rise, or if the sea level changes in the future. It is possible that, if a large amount of the gas hydrates beneath the seafloor become unstable, they could release a lot of methane into the oceans. This would add to problems, such as ocean acidification and some of the methane could make it into the atmosphere, contributing to global warming. Scientists are trying to find out more about this by looking at older sediments to see what might have happened in the past when the climate was colder or hotter, and when sea levels were higher or lower. We can also use computer simulations to try to find out what might happen if these conditions change and the gas hydrates become unstable. Through exploring how gas hydrates are formed, we can better understand how they might be used and what might happen to these gas hydrate reservoirs if the conditions in the ocean change.
Glossary
Gas Hydrate: ↑ A solid form of water, similar to ice, which contains gas molecules locked up in a “cage” of water molecules.
Methane: ↑ A gas that is found in small quantities in Earth's atmosphere. Methane is flammable and is used as a fuel worldwide.
Density: ↑ The density of a substance is the relationship between the mass of the substance and how much space it takes up (volume).
Reflection: ↑ The change in direction of a wave (sound, light, or water waves are the most common) at a boundary between two different substances, so that the wave moves back in the direction that it came from. An echo is an example of a sound wave reflecting off a surface.
Sediment Core: ↑ A cylindrical sample of sediment that is collected from beneath the ground surface or seafloor.
Well Log: ↑ A detailed record of the geological formation during the drilling of a well. A well log is made by either taking samples or by using measuring instruments lowered into the well to record changes in sediment properties, such as resistivity.
Resistivity: ↑ A measure of how much a substance resists or stops the flow of electricity through it. The unit for measuring resistivity is the Ohm meter (Ωm).
Reservoir: ↑ A large supply or store of something.
Conflict of Interest Statement
The author declares that the research was conducted in the absence of any commercial or financial relationships that could be construed as a potential conflict of interest.
References
[1] ↑ Collett, T., Bahk, J.-J., Baker, R., Boswell, R., Divins, D., Frye, M., et al. 2015. Methane hydrates in nature—current knowledge and challenges. J. Chem. Eng. Data 60:319–29. doi: 10.1021/je500604h
[2] ↑ Petroleum Exploration and Production Association of New Zealand. 2019. Seismic Surveys: Exploring What Lies Beneath. Available online at: http://www.seismicsurvey.co.nz/
[3] ↑ Kvenvolden, K. A., Ginsburg, G. D., and Soloviev, V. A. 1993. Worldwide distribution of subaquatic gas hydrates. Geo-Mar. Lett. 13:32–40. doi: 10.1007/BF01204390
[4] ↑ Ruppel, C. 2007. Tapping methane hydrates for unconventional natural gas. Elements 3:193–9. doi: 10.2113/gselements.3.3.193
[5] ↑ Crutchley, G. J., Mountjoy, J. J., Pecher, I. A., Gorman, A. R., and Henrys, S. A. 2016. “Submarine slope instabilities coincident with shallow gas hydrate systems: insights from New Zealand examples,” in Submarine Mass Movements and their Consequences, eds G. Lamarche, J. J. Mountjoy, S. Bull, T. Hubble, S. Krastel, E. Lane, et al. (Cham: Springer International). p. 401–9. doi: 10.1007/978-3-319-20979-1_40