Abstract
Antarctica’s ice sheet is constantly on the move, flowing from the deep, frozen interior of the continent toward the ocean, where it melts. In fact, because the oceans are getting warmer, the Antarctic ice sheet melts faster, and therefore, the sea level is rising. However, predicting how the ice sheet will flow differently from place to place is complicated. The landscape beneath the ice sheet helps to control how fast the ice moves. For example, the ice can stick and deform, or slide smoothly across the land under the ice. Naturally occurring heat from inside the Earth can cause the base of the ice sheet to melt and soften so that it flows more easily, sliding on the meltwater formed. The amount of this geothermal heat varies across Antarctica and is difficult to measure. However, scientists with various expertise can collaborate to understand how much heat there is and how it shapes the ice sheet.
Why Does the Base of the Ice Sheet Melt?
The Antarctic ice sheet covers most of Antarctica. It flows from the interior of the continent toward the surrounding ocean due to gravity (Figure 1). The speed at which the ice sheet flows depends on the slope of the surface and how easily the ice can move over the rocks, sand, and clay beneath it. The ice moves slowly, usually <50 m in a year, when the subglacial landscape is rough, and the ice is frozen to the rocks. However, the ice can move more easily if the subglacial surface is slippery. Water makes surfaces very slippery, so if ice melts at the base of the ice sheet, the ice can slide (Figure 2). Lakes can even form underneath a glacier, where meltwater is trapped under the ice sheet. Some lakes are connected with subglacial rivers that flow toward the ocean, not unlike lakes on other parts of the Earth [1].
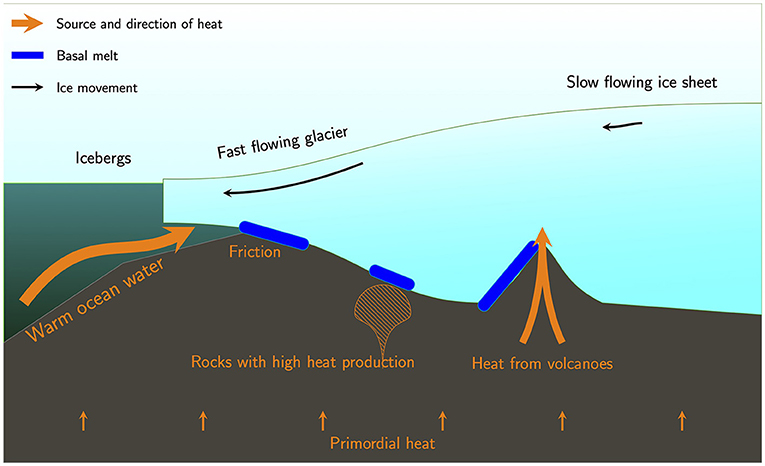
- Figure 1 - Several heat sources can contribute to melt the ice sheet from beneath.
- Warm ocean currents and friction impact the fast flowing glaciers. Geothermal heat under the ice sheet can come from, for example, radiogenic heat and volcanoes, causing the ice to melt at the base and the ice sheet to deform more easily.
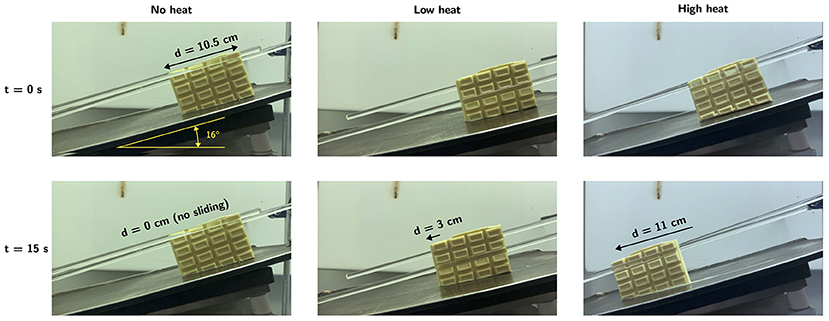
- Figure 2 - The impact of basal (bottom) melting, tested here using white chocolate on a slanted, heated plate.
- The upper row shows the position of the chocolate at the start of the experiment, and the lower row shows the position after 15 s. When heated, the bottom part of the chocolate melts, causing the block of chocolate to flow down the slope. The higher the heat, the more quickly it flows. The same mechanism applies to the Antarctic ice sheet.
Antarctica has the coldest air temperatures on Earth, but due to the geothermal heat, the base of the ice sheet can melt in some places. The melting temperature depends on the pressure, and under kilometers of ice, the base can melt at temperatures colder than 0°C [2, 3]. Even if the ice remains frozen, geothermal heat can still affect how easily it flows. If the ice is very cold, it is hard and rigid and does not flow well, but if it is less cold, ice can deform and start to move. This is similar to the way you may have seen honey flow more easily when it is heated.
Closer to the coast, the ice sheet forms ice rivers called glaciers. Glaciers flow fast, up to many meters a day! With such speed, the ice in the glacier grinds against the rocks beneath, generating heat from friction. The heat from friction is much greater than the geothermal heat and can melt a lot of ice, forming meltwater streams!
Once the ice sheet or glaciers reach the ocean, the ice starts to float and melt, and icebergs can break off. Ocean currents carry much more heat than geothermal heat can provide, so the melting of the ice sheet by the warmer ocean water is much more than the melting of the base caused by geothermal heat and friction. Due to global warming, the melting of ice is speeding up as the Earth’s oceans heat up and sea currents are changing.
Will the ice sheet collapse due to the increased melting at the coast, or will it respond slowly and remain fairly stable? Knowing how much geothermal heat there is at the base of the ice sheet helps us understand how the ice sheet is flowing and how it responds when the oceans and atmosphere get warmer. Geothermal heat is natural, but it impacts how the Antarctic Ice Sheet responds to climate change.
Where Does Geothermal Heat Come From?
It has been known for a long time that the interior of the Earth is warmer than the surface. People have observed hot lava from volcanoes and hot water from thermal springs. In deep mines, it can get very hot! Typically, for every 100 metres deeper into the Earth, the temperature increases by 2–3°C; however, the exact temperature depends on what kinds of rocks and sediments there are.
Heat is energy that makes something else warmer. For example, a hot radiator heats a cold room, and the sun heats the oceans. Scientists usually measure the amount of heat transferred in a certain time using the unit Watt, shortened to W. We can use this unit to measure, for example, the power of an electric kettle that can heat water for tea. For geothermal heat, which varies from place to place, we want to know the rate of heat transferred for every part of the surface area, and the unit we use is Watts per square meter (W/m2). In continental regions, the geothermal heat flow is small; on average, about 70 mW/m2 (one milli Watt is one thousandth of a Watt). This is similar to having a 1,000 W electric kettle every 120 m deep inside Earth to generate heat.
To map how much geothermal heat there is, we must first understand where this heat comes from. Like any form of energy, the natural laws of thermodynamics state that heat must have a source; it cannot appear from nowhere! Heat is either moved from a hotter location or generated from another kind of energy, like the friction of glaciers we described earlier. Geothermal heat comes from several sources (Figure 1); therefore, scientists with diverse skills must collaborate to understand what is generating geothermal heat under the ice.
Some geothermal heat originates deep inside the Earth’s mantle and core; it is left over from when the Earth formed from colliding gas and dust. Because the Earth is so large, the cooling is very slow and has been going on for the entire age of the Earth, about 4.5 billion years. However, this remaining primordial heat is probably not the most significant contribution to the heat under the Antarctic ice sheets today.
Most geothermal heat comes from rocks in the Earth’s crust that contain minerals with elements that can generate heat by low-level radioactivity, called radiogenic heat. The main heat producing elements are uranium, thorium, and potassium. The natural concentrations of these elements are generally low, but some rocks in the crust contain relatively large amounts. A typical value of crustal heat production in Antarctica is 1.3 microWatts per cubic meter (a micro–Watt is one millionth of a Watt) [4]. Assuming that the crust is 40 km thick and that heat production is constant throughout (which it is not!), the radiogenic heat generated in the crust would equal the same amount of heating power as one nuclear power plant every 310 km—that is a lot of heat! However, as radiogenic heat production varies with the type of rock, geologists try to understand which rocks generate the most heat and where those rocks might exist in Antarctica. This is somewhat tricky when the rocks are hidden under thick ice!
Volcanoes and volcanic regions with geothermal hot springs also generate a lot of heat that causes the base of the ice sheet to melt and can even cause the ice to collapse above. Many such volcanoes exist in Antarctica. Some poke their heads up above the ice, while others lie below it https://zenodo.org/record/6535775.
Studying Subglacial Geothermal Heat in Antarctica
Geothermal heat can be calculated by drilling a hole into the ground, measuring temperatures at various depths, and then using the thermal conductivity of the rocks and sediments to calculate the heat flow. However, it is not very easy (or cheap!) to drill through thick ice in Antarctica, so there has not been much data collected this way [5]. Instead, scientists are constructing computer models of the temperatures or heat transfer beneath the Antarctic Ice Sheet.
Some scientists use seismic waves from e.g., earthquakes to calculate how hot the Earth is at various depths. Seismic waves are vibrations similar to sound waves, and they can bounce, twist, and turn depending on the materials they travel through. Typically, seismic waves travel slower in warmer rocks, so by measuring how fast the seismic waves travel through the rocks, seismologists can map the temperature inside the Earth and compute how much heat reaches the surface. Other scientists use variations in Earth’s magnetic field to calculate temperature. When heated to about 580°C, rocks lose any magnetic properties, so measurements of the magnetic field taken by satellites or aircraft can help scientists calculate deep temperatures. Unfortunately, magnetic and seismic studies give, in some places, very different results [6]. The described methods cannot estimate variations in radiogenic heat or thermal conductivity in crustal rocks. We can, apparently, not capture variations in geothermal heat by only using one kind of observation [3].
To get the full picture of all the heat sources, some scientists try to combine seismic data, magnetic data, locations of volcanoes, and other observations to show how the geothermal heat might change from place to place [7]. Combining data from multiple techniques can produce more accurate, detailed results.
However, many places in Antarctica remain unexplored, so the amount of geothermal heat in those areas is uncertain. To get a better map, we need more and better data, we also need the combined knowledge from experts in several scientific fields. Mapping geothermal heat flow in Antarctica is a difficult task because even small variations in the underlying land can significantly impact how the ice sheet flows [8]. The effort is worthwhile because maps of geothermal heat are urgently needed to help us predict ice sheet flow and melting, and how it will change in the future.
Glossary
Ice Sheet: ↑ An ice sheet is a big, dome-shaped layer of ice and snow covering entire lands, like a giant icy blanket, presently found only in Antarctica and Greenland.
Subglacial: ↑ Beneath the ice. The subglacial landscape contains rocks, sand, clay, and water and can be studied using ice-penetrating radar or seismic waves.
Geothermal Heat: ↑ Heat from inside the Earth. “Geo” means Earth, and “thermal” relates to heat.
Glaciers: ↑ Glaciers are massive rivers of ice that move relatively fast toward the oceans and shape the land.
Friction: ↑ The force created when two objects move against each other with resistance. Friction generates heat.
Primordial Heat: ↑ The leftover heat from the intense collisions and impacts of rocks, dust, and gas during the Earth’s formation about 4.5 billion years ago.
Radiogenic Heat: ↑ Radiogenic heat is the heat produced deep within the Earth when elements in certain rocks undergo radioactive decay. This process gradually releases heat energy over incredibly long periods.
Thermal Conductivity: ↑ The ability of a material to conduct heat. Thermal conductivity varies for different kinds of rocks, depending on how much water they contain and how warm they are.
Conflict of Interest
The authors declare that the research was conducted in the absence of any commercial or financial relationships that could be construed as a potential conflict of interest.
Acknowledgments
Discussions informing this review were facilitated by the Scientific Committee on Antarctic Research, Instabilities and Thresholds in Antarctica, sub-committee on Geothermal Heat Flow (SCAR, INSTANT). This research was supported by the Australian Research Council through ARC DP190100418, ARC Special Research Initiatives, Australian Centre for Excellence in Antarctic Science (Project Number SR200100008) and Securing Antarctica’s Environmental Future (SR200100005), ARC DP180104074, and ARC DE210101433. No chocolate was wasted during the experiment presented in Figure 2.
References
[1] ↑ Livingstone, S. J., Li, Y., Rutishauser, A., Sanderson, R. J., Winter, K., Mikucki, J. A., et al. 2022. Subglacial lakes and their changing role in a warming climate. Nat. Rev. Earth Environ. 3:106–24. doi: 10.1038/s43017-021-00246-9
[2] ↑ Noble, T. L., Rohling, E. J., Aitken, A. R., Bostock, H. C, Chase, Z., Gomez, N., et al. 2020. The sensitivity of the antarctic ice sheet to a changing climate: past, present, and future. Rev. Geophys. 58:e2019RG000663. doi: 10.1029/2019RG000663
[3] ↑ Reading, A. M., Stål, T., Halpin, J. A., Lösing, M., Ebbing, J., Shen, W., et al. 2022. Antarctic geothermal heat flow and its implications for tectonics and ice sheets. Nat. Rev. Earth Environ. 3:814–31 (2022). doi: 10.1038/s43017-022-00348-y
[4] ↑ Sanchez, G., Halpin, J. A., Gard, M., Hasterok, D., Stål, T., Raimondo, T., et al. 2021. PetroChron Antarctica: a geological database for interdisciplinary use. Geochem. Geophys. Geosyst. 22:e2021GC010154. doi: 10.1029/2021GC010154
[5] ↑ Burton-Johnson, A., Dziadek, R., and Martin, C. 2020. Geothermal heat flow in Antarctica: current and future directions. Cryosphere Discuss. 14:1–45. doi: 10.5194/tc-2020-59.
[6] ↑ Lösing, M., Ebbing, J., and Szwillus, W. 2020. Geothermal heat flux in Antarctica: assessing models and observations by Bayesian inversion. Front. Earth Sci. 8:105. doi: 10.3389/feart.2020.00105.
[7] ↑ Stål, T., Reading, A. M., Halpin, J. A., and Whittaker, J. M. 2020. Antarctic geothermal heat flow model: Aq1. Geochem. Geophys. Geosyst. 22:1–22. doi: 10.1029/2020GC009428
[8] ↑ McCormack, F. S., Roberts, J. L., Dow, C. F., Stål, T., Halpin, J. A., Reading, A. M., et al. 2022. Fine-scale geothermal heat flow in Antarctica can increase simulated subglacial melt estimates. Geophys. Res. Lett. 49:e2022GL098539. doi: 10.1029/2022GL098539