Abstract
When you ride the bus to school in the morning, your journey is probably powered by diesel or gasoline, which is both made from petroleum. Petroleum is a fossil fuel, which means that it is made from decomposed, fossilized organisms – such as ancient plants, plankton, and algae – that have been buried under the Earth’s surface for millions of years.
Fossil fuels like petroleum, natural gas, and coal are taken from deep within the earth, and used to drive cars, heat buildings, and generate electricity. Petroleum can also be used to make petroleum-based chemicals (petrochemicals), which are found in many everyday things like the soles of your shoes or the plastic cover of your school bus seat.
The good thing about fossil fuels is that they are very energy dense, i.e., they contain a lot of energy per unit of volume. This means that fossil fuels are very good at powering cars and generating heat. The not-so-good thing about fossil fuels is that the earth has a limited amount of them. Because fossil fuels take millions of years to form, we will eventually use them up before more are made. Additionally, burning fossil fuels or petrochemicals releases the gas carbon dioxide (CO2). CO2 is known as a greenhouse gas, because it can trap the sun’s rays inside the earth’s atmosphere, acting just like the glass roof on a greenhouse. Burning fossil fuels raises CO2 concentrations in the atmosphere, and this can lead to climate disruptions including global warming (1).
Because of these problems, scientists and engineers are working hard to find new kinds of fuels and chemicals that do not add CO2 to the atmosphere, and that can be renewed when supplies run low. Fuels and chemicals that meet these requirements are referred to as “sustainable.” In an environmental sense, a material is sustainable if it can be used over the long term, without running out or having an overall negative environmental impact.
Biofuel is one type of fuel that shows a lot of promise for our energy future, because it is both renewable and environmentally friendly. In other words, biofuel is sustainable.
Biofuels are usually produced from plant materials that cannot be eaten by humans, such as corn stalks, grasses, and wood chips. Biomass is another name for the plant materials that are used to make biofuels. When biomass is harvested and processed, scientists can break down and convert the plant cells into renewable fuels or chemicals. So, instead of waiting a million years for nature to change plants into fossil fuels, scientists are trying to speed up this process by using clever chemistry to make biofuel from plants that are alive today.
Now, wait a second. If burning fossil fuels, which are made from ancient organic matter, pumps CO2 into the atmosphere … does not burning biofuels create the same problem? Fortunately, the answer is no. Burning biofuel does indeed release CO2, but remember that the plants used in biofuel are not ancient – they were living on the earth at the same time as you and me. And while we, as humans, breathe oxygen to stay alive, plants instead breathe CO2. This means that because the plants used for biofuel consume CO2 as they grow, there is no total increase in the amount of CO2 in the atmosphere when they are burned. They are only replacing what they have taken. In addition, unlike petroleum, we can always grow new plants for biofuel when we need them.
So, if biofuels are sustainable and environmentally friendly, then they must be the perfect solution to our energy problems, right? Unfortunately, the processes that scientists use to turn biomass into biofuel can be very expensive. Costly chemical reactions mean costly biofuels and bioproducts, and most consumers would rather choose regular gasoline or plastic over more expensive “green” products. In addition, some biofuel reactions require harsh chemicals that can create their own environmental problems, leaving us right back where we started in terms of sustainability (2).
To see how plants are turned into useful fuels and chemicals, we must first understand what they are made of. The walls of plant cells are responsible for almost all of the weight of a plant and are composed of three complex molecules called cellulose, hemicellulose, and lignin (Figure 1).
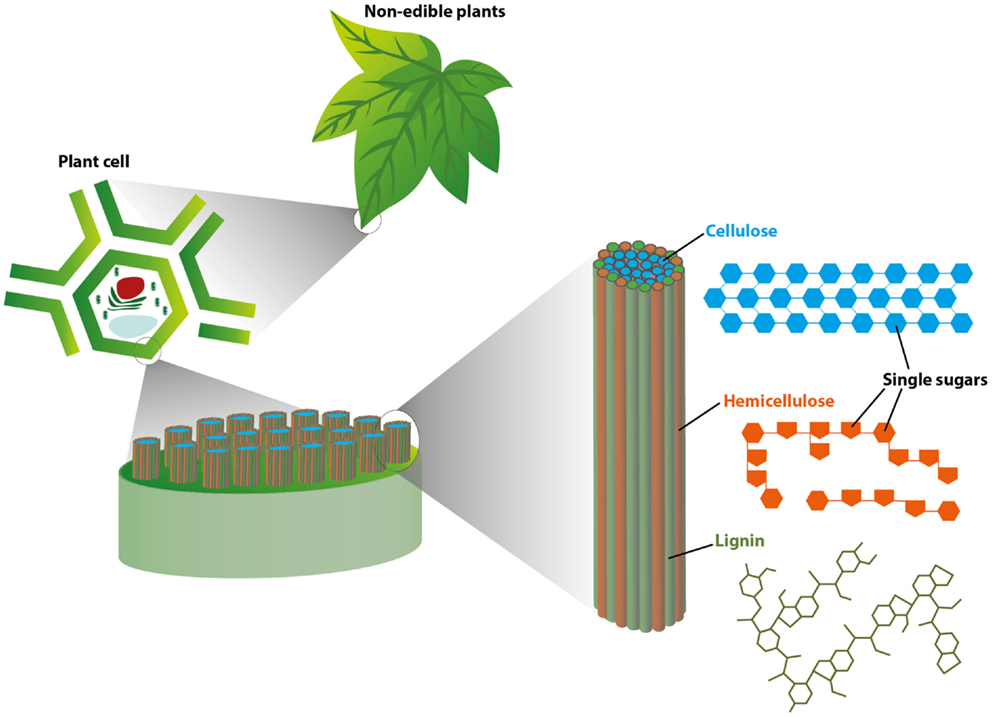
- Figure 1 - This figure illustrates the basic structure of plant tissues, starting at the level of the leaf (Top: “non-edible plants”) and zooming in to the cellular level (Left: “plant cell”).
- As you can see, at the cellular level, long cellulose molecules (shown in blue) are tightly packed into bundles surrounded by hemicellulose (orange) and lignin (green). This tightly packed structure helps make plant tissues strong and durable.
The first two molecules, cellulose and hemicellulose, are bursting with simple sugar building blocks, all bound together in a compact structure that is supported by the third molecule – lignin (Figure 1). All three complex molecules in plants must be broken apart to access the sugar building blocks within, which can then be converted to biofuel.
One way to accomplish this biomass breakdown is to use a lot of harsh chemicals to break apart the plant tissues. However, these chemicals can be expensive – even toxic (2). Ideally, we would like to make breaking down plants easier, so we do not have to rely as much on these chemicals.
One possible solution is to use a solvent – a liquid with chemical properties that allow it to dissolve other materials … like plants. Most of us use solvents every day, even if we are not aware of it. For example, you use water as a solvent every time you wash your hands or make instant hot chocolate.
Sometimes, only a certain kind of solvent can get the job done. For example, water may dissolve cocoa powder to make hot chocolate, but it would not remove nail polish – for that, you need chemicals called acetone, or ethyl acetate.
Unfortunately, until recently, energy researchers could not find a solvent that was (a) cheap, (b) sustainable, and (c) good at breaking down plants. But now, we have discovered a very interesting new solvent called γ-valerolactone (GVL for short) that can make biofuel production much cheaper and more efficient (3). GVL is such an interesting solvent because it is not only cheap – it is renewable, because it is made from biomass itself.
We have discovered that we can use GVL to extract over 70% of the original sugars trapped in the dense structure of biomass, to produce simple sugars that are much easier to transform into fuel. This process is illustrated in Figure 2, which shows the chemical reaction as it proceeds inside a biofuel reactor. Biofuel reactors are metal vessels that contain biofuel-processing reactions. They are specially designed to withstand the heat, pressure, and chemicals involved.
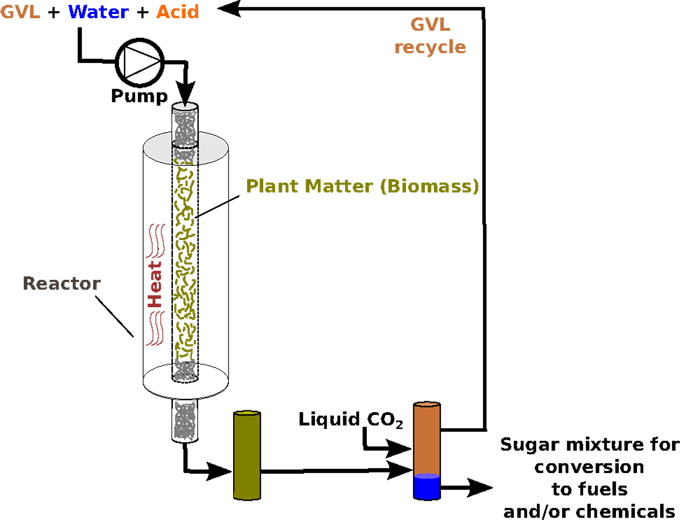
- Figure 2 - Illustration of sugar production from plants, using GVL as a solvent.
Two main properties of GVL make it an excellent solvent for sugar extraction:
(1) GVL gives acids a big boost.
For any chemical reaction to begin, the ingredients involved (the reactants) must first gather enough energy. The smallest amount of energy needed to kick-start a reaction is called the “activation energy” (Figure 3). In common biofuel production reactions, lots of acids are mixed with water to help break down the biomass. This can take awhile, especially for very tough or woody plants, but adding GVL to the reaction gives the acids a big energy boost. This boost helps the system gather its activation energy faster, so the reaction can proceed more quickly (4, 5) (Figure 3).
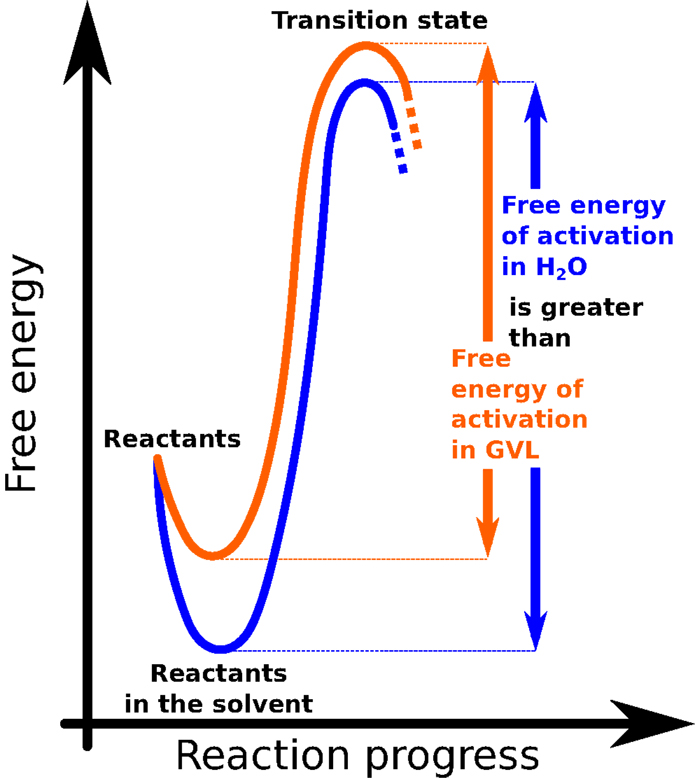
- Figure 3 - This graph illustrates the evolution of a chemical reaction.
- “Free energy” is a fancy way of referring to the energy that is relevant to the chemical reaction. The “reaction progress” represents the state that the reactants must go through in order to turn into products.
To illustrate this phenomenon, imagine that two girls, Gemma and Valerie, are about to race each other to the top of a steep hill. Usually, both runners must stand behind a starting line to make sure that the race is fair. But in this race, Gemma is actually allowed a big head start: when the buzzer goes off, she gets to start running halfway up the steep hill, while Valerie must begin from the very bottom. Who do you think will win? You guessed it – Gemma gets to the top of the hill way before Valerie. Just as the head start puts Gemma closer to the top of the hill in the race analogy, GVL brings the acid closer to the point of reacting with the biomass, allowing the reaction to proceed much faster.
(2) GVL gets lignin out of the way.
To plants, lignin is really important: it gives them their shape and structure, and helps them grow healthy and strong. But to scientists, lignin is just a nuisance. It is a tough and stubborn molecule that is very hard to break down, and it interferes with obtaining simple sugars from cellulose and hemicellulose molecules. One day, scientist hope to be able to break down lignin itself to make useful things, but for now, they just want it out of the way. GVL has the unusual ability to dissolve lignin, and to keep it from blocking the big prize: the energy-rich sugar building blocks.
Perhaps, the best thing about it GVL that it is can be recycled. At the end of a biofuel reaction, liquid CO2 can be added to the reactor to separate each reactant into a distinct layer (Figure 2). Think of a bottle of fancy salad dressing: the oil and vinegar, instead of mixing with each other, stay completely separate until the bottle is shaken. Likewise, when CO2 is added to the biofuel reactor, the GVL and sugar solution become just like that salad dressing. The sugars all move into one layer and become concentrated (see Figure 2), while the GVL forms its own separate layer. The GVL can then be easily removed and used again, while the sugar solution that scientists end up with is around five times more concentrated than it would be without GVL. This increased concentration is very important, because it means that you need to spend less energy purifying the final product, making the whole process more efficient and less wasteful.
After the GVL has been removed, a concentrated – and very useful – sugar solution is left behind. Scientists have two options for using this energy-rich solution:
- They can upgrade the sugars via further chemical reactions to other useful molecules, which are used to make many items that are derived from petrochemicals today. This means that GVL could be used to produce sustainable alternatives to plastics, soaps, paints, and many other common materials.
- They can “feed” the sugars to microorganisms, such as yeast or bacteria, which then metabolize it and produce fuel. The biofuel ethanol is one example: it can power cars and trucks and other machines almost as efficiently as gasoline. Some microorganisms have a particularly good appetite for the sugars produced using GVL, because they are free of the harsh chemicals that are often used in other biofuel reactions. The fact that microorganisms can not only survive but also thrive on GVL-treated sugars means that GVL is suitable for use in other biological reactions – not just chemical ones. In this work, microorganisms were used to produce ethanol concentrations so high that it did not cost very much to purify the ethanol into usable fuel.
For all these reasons, using GVL gives scientists hope for creating biofuels and chemicals that can compete with petroleum products in the marketplace. For centuries now, humans have been inventing new technologies and developing industry at an astounding rate – sometimes at a serious cost to the environment. A biofuel production process that meets all the requirements of affordability, renewability, and sustainability has the potential to benefit both humans and the earth. With the discovery of GVLs role in biofuel processing, we believe that we are one step closer to a sustainable future.
Glossary
Biofuel: ↑ Certain types of plant matter (see biomass) can be processed into liquid or gaseous fuels called biofuels. Some biofuels can provide renewable alternatives to fossil fuels, such as gasoline.
Biomass: ↑ Biomass is a general term referring to any organic (carbon-containing) material that comes from living matter, such as plants. Plant biomass is made up of three main molecules: cellulose, hemicellulose, and lignin. Types of biomass used for biofuels include plants and plant wastes, such as grasses, corn stalks, and wood chips.
Fossil fuel: ↑ Fossil fuels are formed underground over millions of years, and are composed of organic matter from the tissues of ancient plants and animals. Fossil fuels include coal, natural gas, and petroleum. Petroleum can be refined into other fuels, such as diesel and gasoline.
Global warming: ↑ When too much of the gas carbon dioxide (CO2) gets into the atmosphere, it can trap the sun’s rays inside the atmosphere. This phenomenon is called the greenhouse effect, and it can lead to an overall increase in global temperatures called global warming.
GVL: ↑ GVL is short for γ-valerolactone. It is a chemical that can be easily made from plants. In our experiment, we used GVL as a solvent to dissolve plants. In the past, GVL has been used in the perfume industry, because it has a sweet herbal odor. GVL has also been used in pharmaceutical products.
Reaction: ↑ A chemical reaction occurs when atoms in a substance become rearranged, leading to a chemical change in the substance. A chemical reaction can only proceed once it has gathered enough energy. This minimum amount of energy needed to start the reaction is called the activation energy.
Solvent: ↑ In chemistry, a solvent is a liquid or a gas that can dissolve another substance, called the solute. When you add a solvent to a solute, you end up with a solution.
Sustainable: ↑ In an environmental sense, a material is sustainable if it can be used over the long term, without running out or having an overall negative environmental impact. For example, renewable energy is sustainable because we can produce more of it without causing significant damage to the environment. On a larger scale, an ecological system is sustainable if it can survive over time with healthy levels of biodiversity, productivity, and resources.
Original Source Article
↑ Luterbacher, J. S., Rand, J. M., Alonso, D. M., Han, J., Youngquist, J. T., Maravelias, C. T., et al. 2014. Nonenzymatic sugar production from biomass using biomass-derived γ-valerolactone. Science 343:277–280. doi:10.1126/science.1246748
References
[1] ↑ Tester, J. W. 2005. Sustainable Energy. Cambridge, MA: MIT Press.
[2] ↑ Luterbacher, J. S., Martin Alonso, D., Dumesic, J. A. 2014. Targeted chemical upgrading of lignocellulosic biomass to platform molecules. Green Chem. 16:4816–38. doi: 10.1039/C4GC01160K
[3] ↑ Luterbacher, J. S., Rand, J. M., Alonso, D. M., Han, J., Youngquist, J. T., Maravelias, C. T., et al. 2014. Nonenzymatic sugar production from biomass using biomass-derived γ-valerolactone. Science 343:277–80. doi: 10.1126/science.1246748
[4] ↑ Mellmer, M. A., Sener, C., Gallo, J. M. R., Luterbacher, J. S., Alonso, D. M., Dumesic, J. A. 2014. Solvent effects in acid-catalyzed biomass conversion reactions. Angew Chem. Int. Ed. 53:11872–5. doi: 10.1002/anie.201408359
[5] ↑ Mellmer, M. A., Alonso, D. M., Luterbacher, J. S., Gallo, J. M. R., Dumesic, J. A. 2014. Effects of γ-valerolactone in hydrolysis of lignocellulosic biomass to monosaccharides. Green Chem. 16:4659–62. doi: 10.1039/C4GC01768D