Abstract
Do living beings need brains to do extraordinary things? The human brain manages many tasks at the same time, from keeping the body working to thinking and dreaming, thanks to the electrical signals it produces. But what about organisms that survive pretty well without brains, like plants? You might be surprised to learn that plants can produce electricity as well, and they use electrical signals for communication, learning, self-preservation, and as a rapid alarm system against potential threats. All this without any brain cells! With scientific equipment, it is possible to study the hidden electrical activity of plants and discover the secrets of their lives in a challenging world. Plant scientists have developed gadgets like those used to study the brain to explore plant electricity. They have made astonishing discoveries about hidden aspects of plant life. Join us in exploring the electrifying world of plants and uncovering their remarkable powers.
Plants and Electricity
Have you ever wondered about living beings with electric powers? Maybe you have heard of electric eels—fish that can zap other animals with electric shocks to defend themselves or catch prey. Other organisms have this superpower, but not in such an obvious way. This includes humans! We have electricity inside us, powering our brains so that we can think, talk, and remember. Even walking needs electricity!
Speaking of moving, there are some plants that also use bioelectricity to move. Do you remember the Venus flytrap that features in Mario Bros? It snaps shut when an insect lands on it, thanks to a type of electrical signal called an action potential—similar to what happens in our brains. What about most plants, which do not move? They also use electrical signals for communication between their parts. And they do all this without a brain! But how?
Scientists have found that plants produce electricity all the time, and this electrical activity changes when plants notice what is happening around them, from shifts in lighting to the presence of another plant [1]. Imagine if a plant gets too hot or too cold, or if pesky bugs start chewing on it, or even if it gets too much salt in its roots. All these things can cause problems inside the plant (Figure 1A). Just like us, plants have special parts in their cells that make this electricity happen. When some of the electrical activity moves, carrying information, it is called electrical signaling.
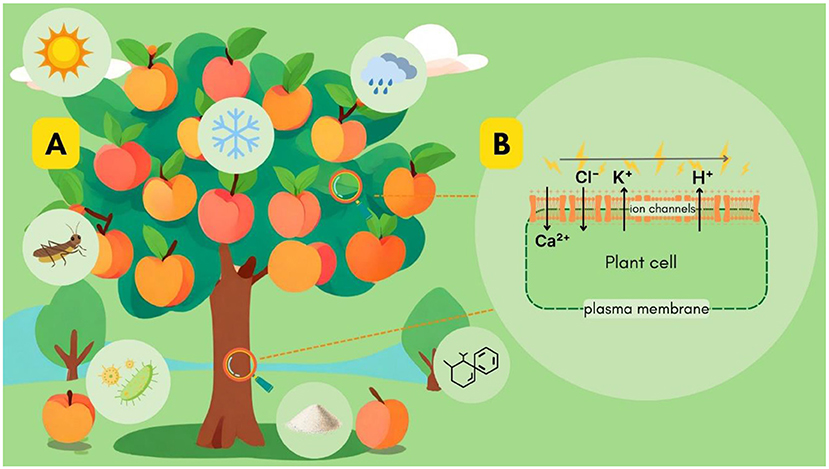
- Figure 1 - The influence of the environment on the generation of a plant’s electrical signals.
- (A) Plants, like trees, receive and perceive different types of stimuli, from sunlight to insects taking small bites. (B) Focusing on a single cell, you can see the components involved in generating an electrical signal that makes part of the plant’s electrome. The ions involved include calcium (Ca2+), potassium (K+), chloride (Cl-), and protons (H+).
How are Electrical Signals Made?
Electrical signals are created by tiny, tiny particles called ions and their strong pull toward each other. Have you heard the phrase “opposites attract”? Well, some ions have positive charges, and some have negative charges, and oppositely charged ions really want to be together. Normally, ions are separated by the plasma membrane, which keeps many ions either inside or outside the cell, preventing them from getting in or out. When the cell allows these ions to move in or out of the cell across the plasma membrane, this creates an electrical signal. You have probably heard of some of these ions: calcium (found in milk), potassium (found in bananas), sodium and chloride (found in kitchen salt), for example (Figure 1B). In addition to the plasma membrane, the membrane of the vacuole—which is like the cell’s storage unit—also plays a big role in the plant cell’s electrical activity, because the vacuole manages many ions. Membranes contain lots of different proteins that help ions move around. Some act like channels, opening and closing to let ions through, while others work like pumps, pushing ions against the flow [2].
Electrical Signals Together: The Plant’s Electrome
Many scientists study specific types of electrical signals in plants. These signals can give valuable information about what the plant is perceiving or doing. However, inside plants, everything happens at the same time. Studying signals individually might not give scientists the whole picture, and they could miss important details about what is happening in the plant. This is where electrome analysis comes in! A scientist named Arnold De Loof defined the electrome as “all the electrical currents in different parts of any living thing” [3]. Other scientists, including some authors of this article, applied the electrome concept to plants [2].
Inside plants, many processes create electrical activity. This electrical activity is used to send messages between parts of the plant (like roots and leaves), to activate photosynthesis (which is how plants make their own food), to regulate transpiration (basically plant perspiration—sweating), and to control the uptake of nutrients from the soil by the roots. In all these processes, ions are moving around. This movement changes throughout the day and during specific stages of the plant’s growth and development, and it also varies between species, making the electrome of each plant unique [2].
What Can a Plant’s “Electrical Signature” Tell Us?
Things happening around the plant, like animals trying to eat it or sudden changes in temperature, can affect how the plant works, creating specific “electrical signatures” that can be grouped into specific characteristics. How do scientists record and analyze plants’ electrical signatures? To collect electrical data from plants, we use a technique very similar to the one used to measure the electrical activity of brains. After getting the data, we need to interpret it using mathematical calculations and statistics, along with artificial intelligence, which helps us to understand large amounts of data. As a result, we can gather a lot of information about the electricity of plants. And what can we do with all this information?
The analysis of the plant electrome can help scientists to understand plants better. Scientists have learned how electrical activity influences plants’ ability to memorize, learn, and communicate. They have also found practical uses for the plant electrome in agriculture, such as identifying diseases caused by fungi before symptoms even appear and detecting if pests are attacking plants [4, 5]. In the future, scientists might be able to attach gadgets to plants to understand what is happening inside them (Figure 2). This could help us to conserve natural resources, like water, and to use less chemicals, like insecticides and fungicides, to control pests. So, understanding the plant electrome can help produce more sustainable food and decrease the damage we cause to our planet!
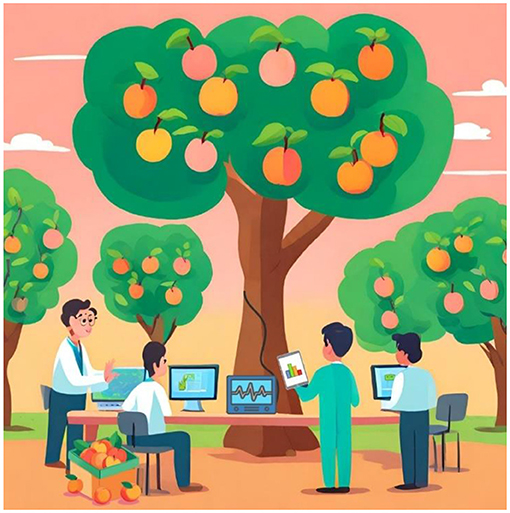
- Figure 2 - Scientists and researchers of the future, recording the electrome of peach trees and analyzing the data to detect diseases early and check if the plant has enough water and nutrients.
Glossary
Electrical Signal: ↑ When ions move in and out of a cell, they change the electrical charge on the cell’s surface. This creates electrical signals that carry messages from one cell to another.
Action Potential: ↑ A quick electrical signal that travels through cells in plants and animals. It always has the same strength and helps cells communicate, allowing things like movement or reactions to happen.
Ions: ↑ Tiny particles with an electric charge, either positive or negative. They move in and out of cells and help plants and animals do important things like send signals or grow.
Plasma Membrane: ↑ A thin layer that surrounds and holds everything inside a cell. It controls what goes in and out of the cell, like ions and water.
Vacuole: ↑ A storage space inside cells. In plants, it stores water, ions, and waste. It helps the cell stay healthy and keep its shape.
Electrome: ↑ The collection of all the electrical signals and activities that happen in plants and animals over time.
Artificial Intelligence: ↑ A computer process designed to act like humans to solve problems. It learns from lots of information to do things like answering questions, playing games, or sorting information.
Sustainable: ↑ Something created without harming the environment or using up resources, ensuring they last a long time. It is about protecting the planet so we can keep living here.
Conflict of Interest
The authors declare that the research was conducted in the absence of any commercial or financial relationships that could be construed as a potential conflict of interest.
Acknowledgments
GR and TO dedicate this work to their beloved daughter Éris, with the sincere hope that she, along with many other young individuals, may have access to a science that is inclusive, igniting their curiosity, and empowering them to explore its wonders.
Original Source Article
↑de Toledo, G. R. A., Parise, A. G., Simmi, F. Z., Costa, A. V. L., Senko, L. G. S., Debono, M.-W., et al. 2019. Plant electrome: the electrical dimension of plant life. Theor. Exp. Plant Physiol. 31:21–46. doi: 10.1007/s40626-019-00145-x
References
[1] ↑ Parise, A. G., Reissig, G. N., Basso, L. F., Senko, L. G. S., Oliveira, T. F. C., de Toledo, G. R. A., et al. 2021. Detection of different hosts from a distance alters the behaviour and bioelectrical activity of Cuscuta racemosa. Front. Plant Sci. 12:594195. doi: 10.3389/fpls.2021.594195
[2] ↑ De Toledo, G. R. A., Parise, A. G., Simmi, F. Z., Costa, A. V. L., Senko, L. G. S., Debono, M.-W., et al. 2019. Plant electrome: the electrical dimension of plant life. Theor. Exp. Plant Physiol. 31:21–46. doi: 10.1007/s40626-019-00145-x
[3] ↑ De Loof, A. 2016. The cell’s self-generated “electrome”: the biophysical essence of the immaterial dimension of life? Commun. Integr. Biol. 9:e1197446. doi: 10.1080/19420889.2016.1197446
[4] ↑ Simmi, F. Z., Dallagnol, L. J., Almeida, R. O., Dorneles, K. R., and Souza, G. M. 2023. Barley systemic bioelectrical changes detect pathogenic infection days before the first disease symptoms. Comput. Electron. Agric. 209:107832. doi: 10.1016/j.compag.2023.107832
[5] ↑ Reissig, G. N., Oliveira T. F. C., Oliveira, R. P., Posso, D. A., Parise, A. G., Nava, D. E., et al. 2021. Fruit herbivory alters plant electrome: evidence for fruit-shoot long-distance electrical signaling in tomato plants. Front. Sustain. Food Syst. 5:657401. doi: 10.3389/fsufs.2021.657401