Abstract
The brain is made up of thousands of cells that make sense of what a person experiences. These cells, which are called neurons, all have different jobs depending on where they are in the brain. Scientists use multiple methods to collect information about how and when neurons react; this is sort of like taking a picture of the brain’s activity! To understand the jobs neurons do, researchers generally use methods that do not go directly into the brain. These approaches are commonly used because they are convenient; however, because they are “pictures” taken from outside the skull, the information they provide scientists may be incomplete or inaccurate. Fortunately, it is also possible for researchers to use a method that goes directly inside the brain tissue, called single-cell recording, to more accurately capture what a neuron is doing.
How Brain Cells Communicate
Neurons are brain cells that can “talk” to each other. Everything our brains do—all our thoughts, feelings, and experiences—arise because of these neuronal “conversations”. Communication between neurons is critical for brain functioning, but each neuron does not just pass along every single message it “hears”. Instead, it only decides to “speak” when it has received enough positive information from its neighbors; otherwise it remains “quiet”. Imagine you hear a rumor from your classmates that the class will have a pizza party the next day. If only one person tells you about the party, you may be cautious or skeptical about the information and choose not to spread the rumor yourself. But once you hear, say, 10 people tell you about the party, you will likely start to believe it, and then you may start to tell others about the upcoming event.
Neurons do more or less the same thing, but with action potentials (APs) instead of rumors (Figure 1). APs are bursts of electrical activity within a neuron that result in messages being sent from the neuron to its neighbors. Neuron communication is “all or none”, meaning that a neuron only performs an AP if it has received enough information from its neighbors to trigger the cell into communicating with other neighboring neurons. When neurons reach a certain threshold of activity, an AP travels down the neuron’s tail (called its axon), causing a release of chemical neurotransmitters that pass along information to neighboring cells. These chemical messages are received by the nearby neurons (kind of like you being close enough to a friend to overhear their rumor).
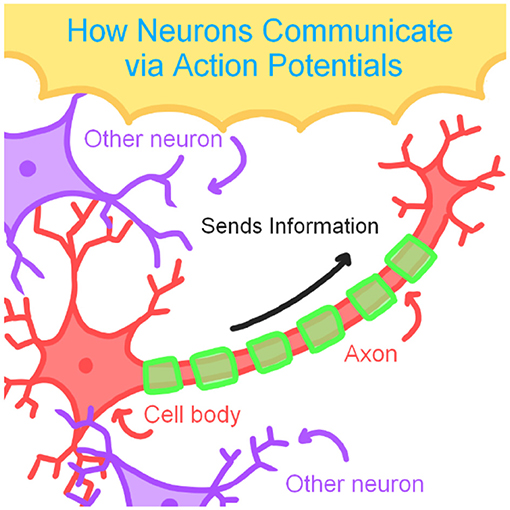
- Figure 1 - When a neuron receives enough stimulation from its neighbors, an action potential occurs, sending a pulse of electricity down the axon, which triggers the release of neurotransmitters that communicate to other nearby neurons.
How is Neuron Activity Measured?
There are many ways to collect information from neurons, but some of these methods have shortcomings that prevent scientists from getting as clear of a picture as they might like. An example of such a method is functional magnetic resonance imaging (fMRI). This method can record neuron activity in the brain by monitoring changes in the brain’s blood flow. When an area of the brain is active it consumes energy, so blood is sent to that area to “refuel” it with oxygen. The refueling process is what fMRI measures. Another common method is known as electroencephalography (EEG). Unlike fMRI, EEG specifically measures the activity of neurons rather than blood flow, so this method is commonly used to diagnose brain disorders [1]. Many EEG studies can be completed in an hour or less. In EEG, researchers attach an arrangement of sensors called electrodes to a person’s scalp to record neuron activity. The electrodes give researchers a rough idea of how the neurons communicate with each other in real time, kind of like eavesdropping on conversations happening at the cafeteria table next to you.
These methods collect data externally (from the outside of the skull), so the skull and scalp can interfere with the information that is gathered. For instance, when researchers measure neuron activity by EEG, it may be difficult to determine precisely where the activity originates from. Imagine trying to use a camera to take a picture of a tree through a heavily tinted window. You know you are taking a picture of a tree, but the picture may be unclear (e.g., you might not be able to make out individual leaves) because the window tint is interfering with getting a detailed picture.
The information scientists collect is more precise when it is collected directly from the brain using internal methods—from inside the skull. Researchers occasionally use a method known as single-cell recording to record the activity of an individual neuron or a small group of neurons. Because single-cell recording bypasses the skull and directly records from the neuron, neuron activity can be much more accurately recorded. With single-cell recording, the tinted window between the tree and the camera disappears, and a much clearer picture results.
When recording the activity of single neurons, direct access to the brain is needed (Figure 2). This unfortunately means that, to collect this type of data, the person’s skull must be opened. This method is therefore very invasive, requiring serious surgery. This method is also typically more expensive, which also makes it less available to researchers compared to EEG. While single-cell recording may be more precise than external methods, its invasive nature means that there must be a very good reason to do it in the first place!
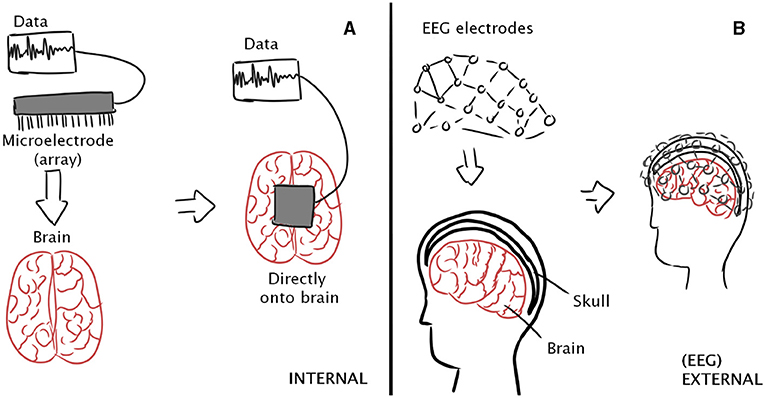
- Figure 2 - (A) In single-cell recording, researchers record signals directly from brain tissue, which provides a very clear picture of neuron activity.
- (B) In EEG, neuron activity is recorded from a bunch of electrodes placed on the outside of the skull. Because measurement is external, the skull and scalp can interfere with the picture, so the picture of neuron activity is less certain.
To listen to neurons using single-cell recording, researchers insert a tiny instrument called a microelectrode array into brain tissue [2]. The microelectrode array is similar to the electrodes placed onto the scalp in EEG, but microelectrodes are much smaller, enabling precise data to be collected from single neurons (or a small number of neurons). When the skull is opened surgically to expose the brain, the subject can have multiple microelectrodes implanted at once, in different areas of the brain [3]. This allows the researcher to “listen” to what the brain is saying in multiple places.
When and Why Do Scientists Use This Tool?
Single-neuron information is generally collected from patients experiencing epilepsy—a seizure disorder that affects the brain’s electrical activity. In some instances, epilepsy patients may already be undergoing surgery for their seizures. One way of thinking about seizures is that they occur when the normally clear conversations between neurons become unintelligible. Say your classmates screamed excitedly at you instead of calmly telling you about the pizza party rumor. Instead of understanding them, you might end up being confused. In some people, their neurons send a lot of conflicting information very close together in time. When neurons get overwhelmed, they can no longer do their jobs properly. They may increase or decrease the number of action potentials they send out, which can result in a seizure. Seizures can be isolated to one brain area so, for example, a seizure in the area that controls movement may result in a person shaking or jerking uncontrollably. Epilepsy patients may have single-neuron data collected to better pinpoint the exact location of their seizures. As a result, single-neuron data for other experiments can also be easily collected from these patients.
Studies like these performed on epilepsy patients have resulted in many important findings about neuron activity. In one study, researchers implanted electrodes into the brains of 19 epilepsy patients, placing the electrodes in various locations depending on where previous EEG recordings revealed that the patients’ seizures originated from [4]. The researchers recorded the patients’ neuron activity, including interictal epileptiform discharges (IEDs), which are large, random electrical spikes in neuron activity common in epilepsy patients. The researchers then had the patients perform a memory task. Patients were presented with a series of 100 images shown 1 second apart. Later, the images presented were mixed with entirely new images, and the subjects were asked to recall which of the images they saw previously. In addition to recording large spikes of neuron electrical activity typically found in EEG recordings of epilepsy patients, researchers also found that IEDs may impact memory if they happen up to 2 s before the images were shown. This kind of information provides the researchers with important clues about how specific brain activity relates to memory storage and later retrieval.
Listening to Brain Cells in the Future
Single-cell recording, while invasive, is an important tool that can help researchers gain a more accurate reading of how neurons communicate. This method is not widely used in current medical treatments, but it can be used alongside other tools, like EEG, to help with diagnosis of epilepsy. In the future, single-cell recording may give us crucial answers to important questions, such as which brain areas are involved in memory, or what precisely is happening in certain brain diseases. Furthermore, because single-cell recording collects the electrical impulses of single neurons, implants could be developed to help people suffering from movement disabilities (e.g., paralysis patients or those recovering from strokes), by sending the brain’s electric signals to brain-controlled robotic devices that assist with movement. Microelectrodes will soon be able to remain in the brain for a longer period of time, further helping patients to regain their independence and mobility. Clearly, single-cell recording is an important tool for studying the brain, but it is also great for studying the root causes of epilepsy and will likely lead to a variety of other medical advances.
Glossary
Neuron: ↑ A type of brain cell capable of communicating with other neurons via electrochemical messaging.
Action Potential: ↑ A change in electrical charge across a neuron’s cell membrane that results in the release of neurotransmitters.
Neurotransmitters: ↑ Molecules that allow neurons to communicate with each other.
Functional Magnetic Resonance Imaging (fMRI): ↑ A method that measures brain activity by observing changes in blood flow.
Electroencephalography (EEG): ↑ A test that measures electrical signals in the brain using small electrodes placed on a person’s scalp.
Single-cell Recording: ↑ A method of measuring activity in a single neuron by using a microelectrode.
Microelectrode Array: ↑ A device that contains many rows of tiny electrodes used to measure brain cell activity.
Interictal Epileptiform Discharges: ↑ Large, random spikes in electrical activity that occur in the neurons of epileptic patients.
Conflict of Interest
The authors declare that the research was conducted in the absence of any commercial or financial relationships that could be construed as a potential conflict of interest.
References
[1] ↑ Beres, A. M. 2017. Time is of the essence: a review of electroencephalography (EEG) and event-related brain potentials (ERPs) in language research. Appl. Psychophysiol. Biofeedb. 42:247–255. doi: 10.1007/s10484-017-9371-3
[2] ↑ Harris, K. D., Quiroga, R. Q., Freeman, J., and Smith, S. L. 2016. Improving data quality in neuronal population recordings. Nat. Neurosci. 19:1165–1174. doi: 10.1038/nn.4365
[3] ↑ Chari, A., Thornton, R. C., Tisdall, M. M., and Scott, R. C. 2020. Microelectrode recordings in human epilepsy: a case for clinical translation. Brain Commun. 2:2. doi: 10.1093/braincomms/fcaa082
[4] ↑ Reed, C. M., Mosher, C. P., Chandravadia, N., Chung, J. M., Mamelak, A. N., and Rutishauser, U. 2020. Extent of single-neuron activity modulation by hippocampal interictal discharges predicts declarative memory disruption in humans. J. Neurosci. 40:682–693. doi: 10.1523/JNEUROSCI.1380-19.2019