Abstract
Continents are constantly moving, and sometimes they collide. When continents collide, they crumple, and thicken. Mountain ranges form in this “crash zone.” Deep rocks at the bottom of a crash zone are hot because they are so deep. Hot materials—even rocks—become weak. Hot rocks deep underground can move by flowing, even though they are mostly solid. First, they flow sideways and then upwards in large blobs. When upward-moving blobs are only a few kilometers below the surface of the Earth, they cool and harden into bell shapes (domes). Flowing rocks cause the crash zone to collapse and spread out. Continents go back to their pre-collision thickness. They are not exactly the same as before collision, though: some rocks that used to be at the bottom of the continents are now at the top! We can see these formerly deep parts of continents in rock domes all over the world.
Crashing Continents
Recent research has proposed that many rocks that we see on the outermost surface of continents used to be deep underground [1]. How did they get to the surface—that is, the part of the Earth that we live on? What can we learn from these rocks about the formation of continents and mountain ranges?
To answer these questions, we must first understand what happens when continents collide with each other. The continents slowly move around the planet as part of tectonic plates (Figure 1A). Sometimes continents collide with each other. Continent collision forms large mountain ranges in the “crash zones” where the two continents impact each other (Figure 1B). Away from crash zones, continents are about 30 kilometers thick. In crash zones such as the Himalayas, the crust is more than 70 kilometers thick. Continents double in thickness in crash zones!
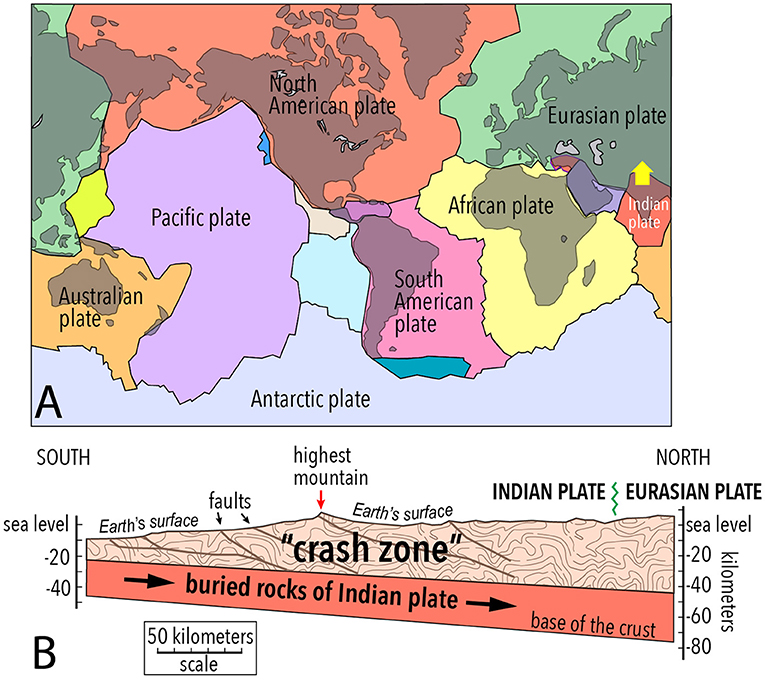
- Figure 1 - (A) A map of the Earth’s tectonic plates.
- The yellow arrow at the boundary of the Indian and Eurasian plates marks the collision of these two plates, where the Himalaya Mountains formed and continue growing today. (B) A side-view of a collision (“crash”) zone, showing what is happening underground. In this example, the Indian plate (dark orange) is sliding under the Eurasian plate (light orange). In the crash zone, rocks are being smashed, buried, and heated (metamorphosed).
Now let us consider what happens in the deep parts of the crash zones of colliding continents.
Rocks Under Pressure
Temperature increases with depth inside the Earth. Why? Because the center of the Earth is extremely hot, and the surface of the Earth is much cooler. In between, temperatures are cooler near the surface and hotter in the deeper parts. So, the rocks in the deep underground parts of crash zones are much hotter than the outermost parts of the Earth that we walk around on. The rocks in the deep parts of crash zones are also under a lot of pressure from the weight of all the rocks above them. This type of deep, hot rock is called metamorphic rock (Figures 2A–C).
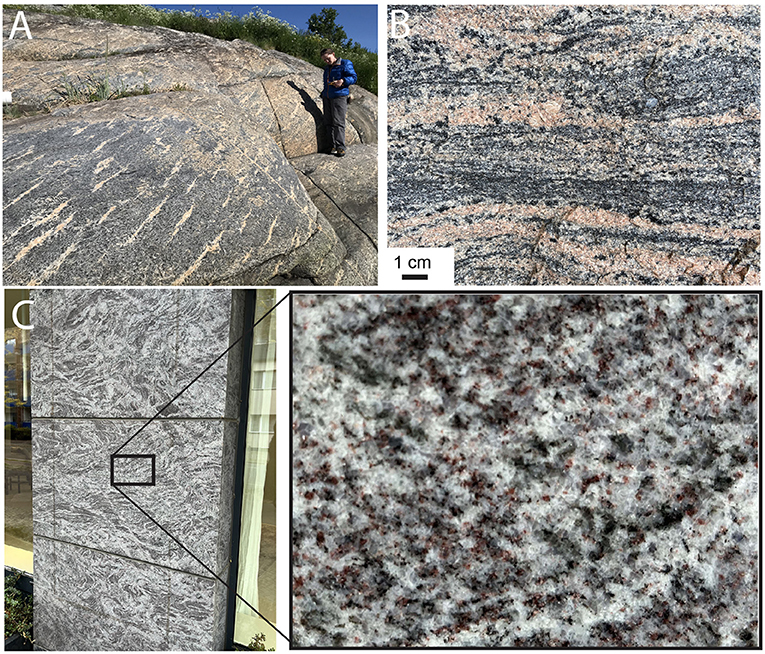
- Figure 2 - (A) Metamorphic rock exposed at the Earth’s surface, which contains a small amount of previously melted rock (light-colored streaks).
- This rock is in Norway. (B) A metamorphic rock in Minnesota USA. (C) Metamorphic rocks (gneiss) on the outside wall of a building in Berlin, Germany. The small red spots are garnets, which you may be familiar with as red gemstones.
Metamorphism means change. Metamorphic rocks are rocks that have changed by being heated and pressed. That is exactly what happens in crash zones: rocks are buried (pressed) and heated. Many buildings have metamorphic rocks on their walls, floors, countertops, and columns (Figure 2C). So, you may not have to travel very far to see some beautiful metamorphic rocks. Some examples of metamorphic rocks and their starting materials are:
- slate: metamorphosed shale or mudstone;
- marble: metamorphosed limestone; and
- quartzite: metamorphosed sandstone.
Why Are Metamorphic Rocks Interesting to Study?
There are practical reasons why it is important and interesting to study the composition and conditions of metamorphic rocks. How hot and how deep the rocks were affects which minerals form. Minerals in metamorphic rocks are used for building materials, technologies (computers, phones), and many other things. Understanding how and where metamorphic rocks formed also helps us figure out how the planet works. We will explore this aspect in the rest of the article.
Metamorphism occurs at temperatures hotter than even the very hottest day on the surface of the Earth. And, as you have learned, it occurs underground. So, when we see metamorphic rocks at the Earth’s surface, we know that they have moved and cooled down. This raises a lot of questions about what happened to the rocks before they got to the Earth’s surface. Geologists study metamorphic rocks to answer questions such as: How deep were the rocks at their very deepest and how hot did they get? When were the rocks deep underground and when did they get to the Earth’s surface? How quickly did they move (flow) from deep underground to the surface? How far can rocks travel underground? Why do metamorphic rocks flow? And how much of the continent used to be at great depth but is now exposed at Earth’s surface?
These questions can be answered by identifying the minerals that make up the rocks and analyzing the amounts of the various elements they contain. For example, in some minerals, the ratio of iron and magnesium is an indicator of how hot the rocks were during metamorphism. The ratio of uranium and lead in some minerals is an indicator of age. From these methods, we know that many metamorphic rocks at the Earth’s surface today came from the very deepest and hottest parts of continents. So, we have an answer to the “how deep” question: some rocks now at the Earth’s surface came from near the base of the thickest regions of continental crash zones: approximately 70 kilometers down. We also know the answer to the “how hot” question. Knowing the temperature is important because it helps us understand where the rocks were and how weak they were. That tells us how far the rocks could have flowed. Some metamorphic rocks reached temperatures of 800°C. This is hot enough for most types of rocks to start to melt.
Rocks in Motion
Hot rocks are weak and can move by flowing. These hot rocks are not lava; they move as mostly solid rock. They may have a small amount of melted rock in them (Figures 2A, B), which makes the rocks even weaker and helps them flow. Why do they move? Imagine what would happen if you made a big pile of something weak like jam or porridge. It would flow out and away from the pile. Something similar happens with thick crash zones that have hot, weak rocks under them (Figure 3A). Some of the flow of hot rocks is sideways, that is, it stays at about the same depth. Some of the flow is upward. Rocks can flow upward if they are less dense than the rocks around them. Hot rocks can flow very fast —for rocks [2]. “Fast” for metamorphic rocks is very slow for a human. A metamorphic rock that moves a centimeter or two in a year is racing along!
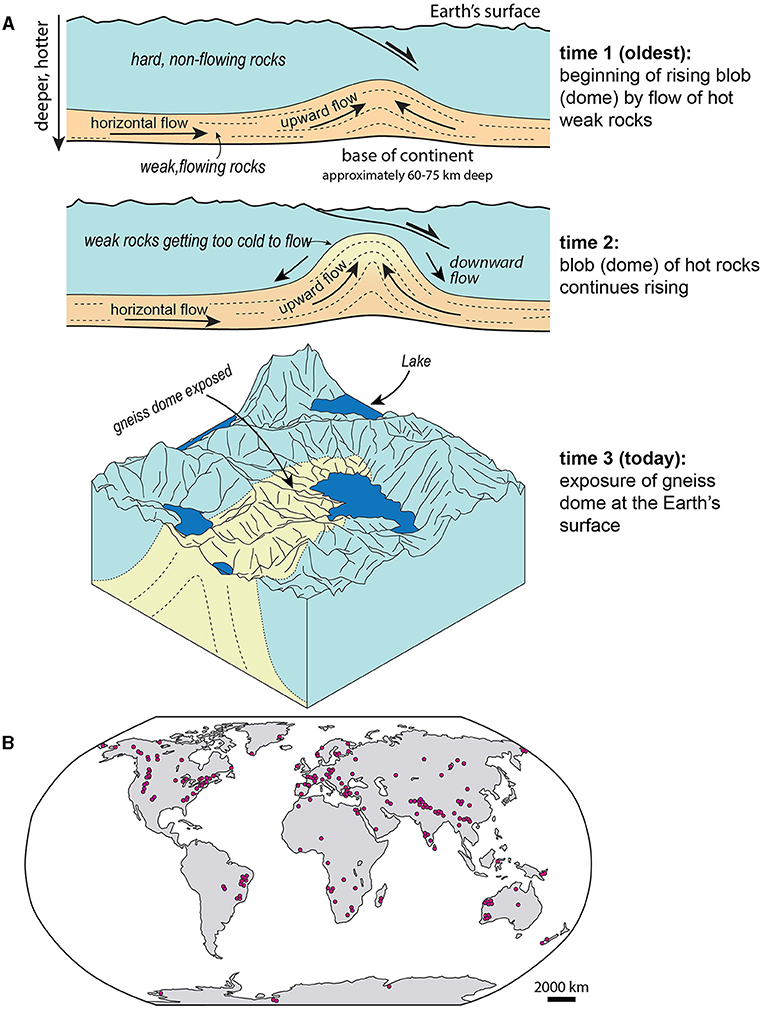
- Figure 3 - (A) A side-view of flowing metamorphic rocks that rise to form gneiss domes, showing how the dome develops through time.
- The top panel shows an early stage—the hot rocks start to rise. The middle panel shows a later stage—the dome is closer to the Earth’s surface. The last panel is how the gneiss dome looks today. We can only see the top part where it is exposed. Geologists guess what the rest of the dome might look like by studying the metamorphic rocks that are exposed. (B) Some locations of gneiss domes in the world today.
Rock Domes: Clues to Flowing Rocks
Hot rocks flow upward in large blobs toward the outer surface of the Earth. To picture these blobs of rock, imagine the rising blobs in a lava lamp or giant balloons floating upwards. When hot rocks flow upwards in blobs, the blobs do not flow all the way to the Earth’s surface. They cool down too much when they start to get near the surface. When they get too cold, they cannot flow anymore. However, the rising blobs can get within a few kilometers of the Earth’s surface. With time, erosion wears away the soil and rock on top of the rock blobs (Figure 3A). Then we can finally see them!
The domes of rocks that we see on the Earth’s surface are called gneiss domes. Gneiss, pronounced “nice,” is a very common kind of metamorphic rock that experiences a lot of heat and pressure. “Dome” refers to the blob shape that forms as the gneiss flows upward. Gneiss domes can be seen in continents all over the world (Figure 3B) [1, 3]. They contain clues about what happens deep underground when continents collide and form large mountain ranges.
One way to study the deep parts of continents is to find places where these rock blobs have flowed upwards and been exposed. This allows us to see rocks that formed deep underground where continents collided to form mountain ranges. We have learned a number of things from gneiss domes—here are just a few. First, many rocks at the surface of continents today were originally very deep underground [1]. Second, gneiss domes may not look dome-shaped if erosion has carved the land surface into peaks and valleys (Figure 3A). However, geologists can still recognize gneiss domes by looking at how rock layers are tilted away from each other to form a dome. Third, we have learned that the rocks in some gneiss domes flow upwards from near the base of a continent to near the surface. They travel upward for nearly the entire thickness of a continent—from approximately 70 kilometers to within a few kilometers of the Earth’s surface! Finally, before the rocks in gneiss domes flow upward, they may flow horizontally in large sheets for tens to hundreds of kilometers [4]. Picture a wide and deep underground river of flowing rock.
Summary
Metamorphic rocks are the key to learning what happens in the deep parts of the collision zones between continents. Recent studies show that rocks that used to be deep underground and very hot moved rapidly in blobs toward the Earth’s surface. The blobs flowed as mostly solid rock. In many places around the world today, we can see these blobs as domes made of metamorphic rocks. They have traveled very far from deep underground. Rock (gneiss) domes help us understand how deep rocks get to the Earth’s surface. By studying gneiss domes, we can understand how continents form and change with time.
Glossary
Continent: ↑ A large region of land above sea level; there are six continents today but there have been many different continents in the past.
Tectonic Plate: ↑ A large slab of solid rock that forms the outermost solid layer of the Earth. Many plates contain continents as well as the rocks under the oceans.
Continent Collision: ↑ When two continents move toward each other and smash together, forming a mountain range.
Metamorphic Rock: ↑ A type of rock that forms at temperatures and pressures higher than those found at the Earth’s surface; metamorphism occurs in solid rocks, without melting.
Gneiss Dome: ↑ A dome-shape feature made of a metamorphic rock called gneiss; domes range from a few kilometers to more than a hundred kilometers wide.
Conflict of Interest
The authors declare that the research was conducted in the absence of any commercial or financial relationships that could be construed as a potential conflict of interest.
Acknowledgments
This research was funded by the US National Science Foundation, grant NSF-EAR-1946911 to DW and CT.
Original Source Article
↑Whitney, D. L., Hamelin, C., Teyssier, C., Raia, N. H., Korchinski, M. S., Seaton, N. C. A., et al. 2020. Deep crustal source of gneiss dome rocks revealed by coeval eclogite and migmatite in the Montagne Noire dome, French Massif Central. J. Metamor. Geol. 38:297–327. doi: 10.1111/jmg.12523
References
[1] ↑ Whitney, D. L., Hamelin, C., Teyssier, C., Raia, N. H., Korchinski, M. S., Seaton, N. C. A., et al. 2020. Deep crustal source of gneiss dome rocks revealed by coeval eclogite and migmatite in the Montagne Noire dome, French Massif Central. J. Metamor. Geol. 38, 297–327. doi: 10.1111/jmg.12523
[2] ↑ Guevara, V. E., Smye, A. J., Caddick, M. J., Searle, M. P., Olsen, T., Whalen, L., et al. 2022. A modern pulse of ultrafast exhumation and diachronous crustal melting in the Nanga Parbat Massif. Sci. Adv. 8:2689. doi: 10.1126/sciadv.abm2689
[3] ↑ Teyssier, C., and Whitney, D. L. 2002. Gneiss domes and orogeny. Geology 30:1139–1142. doi: 10.1130/0091-7613(2002)030%3C1139:GDAO%3E2.0.CO;2
[4] ↑ Clark, M. K., and Royden, L. H. 2000. Topographic ooze; building the eastern margin of Tibet by lower crustal flow. Geology 28:703–706. doi: 10.1130/0091-7613(2000)28%3C703:TOBTEM%3E2.0.CO;2