Abstract
Secretion is a fundamental process in which cells release substances to their external environments. Secretion is essential for many body functions, including growth, digestion, and cell communication. To be secreted, proteins must pass through various stations inside cells, which together form what is called the secretory pathway. In this article, I will tell you about the secretory pathway and the stages that proteins go through from their production up until their secretion. Then, I will present the original work that we did in our lab to identify genes related to the secretory pathway in yeast cells. Finally, I will explain the importance of our work to the overall study of the secretory pathway, both in yeast and mammalian cells.
Professor Randy Schekman won the Nobel Prize in Physiology or Medicine 2013, jointly with Prof. James Rothman and Prof. Thomas Südhof, for their discoveries of machinery regulating vesicle traffic, a major transport system in our cells.
The Secrets of Secretion
Our complex bodies perform various functions, many of which require the transportation of substances from one place to another. One key process involved in moving substances around in the body is called secretion [1]. Secretion is the controlled release of substances from inside a cell to the blood or to other cells. For example, cells in the digestive tract secret digestive enzymes that help break down the foods that we eat, and cells in our glands secrete hormones that support our growth and development. Another type of secretion occurs in the brain, where nerve cells secrete messengers called neurotransmitters to communicate with other nerve cells.
Before a cell secretes a substance, the substance must first be produced within the cell and then transferred from inside the cell across a membrane to be released outside the cell. Sounds simple, right? Well, this process is actually quite complex. As you might already know, the cells of all eukaryotes including humans are built in a compartment-like structure and contain various “organs”, called organelles (Figure 1A). Each organelle performs specific functions and requires its own special environment to function optimally. This environment can be very different, and often compete with the environments of other organelles or other areas within the cell. Therefore, every organelle is enclosed by a membrane that separates it from the rest of the cell. This membrane is like a barrier that prevents the free movement of substances into and out of the organelle, in the same way that the cell’s membrane prevents the free movement of substances into and out of the cell. So, it seems that we have a problem—how do substances move through those membranes to be secreted, if the membrane’s job is to prevent the substances from passing (Figure 1B)?
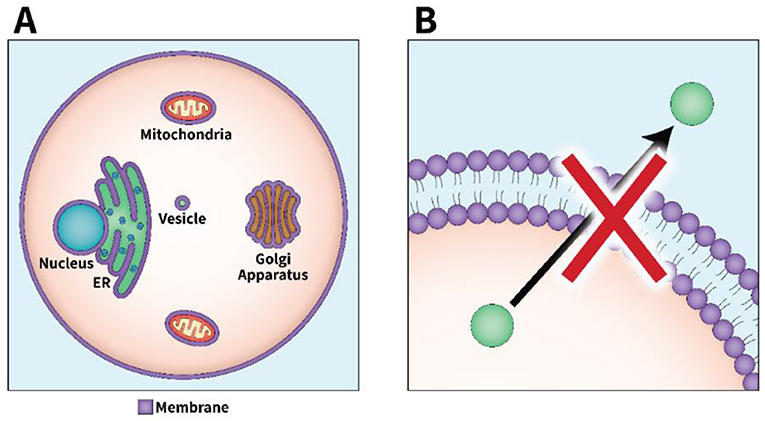
- Figure 1 - A cell’s organelles are important for transporting materials.
- (A) A eukaryotic cell is defined as a cell with a nucleus and multiple organelles that are separated from one another by membranes. Cells also have a plasma membrane that separates the contents of the cell from the outside environment. A cell’s membranes block many substances from passing through them. (B) The process of secretion requires materials to be passed between organelles and to the outside of the cell. But most materials cannot freely pass through the membranes covering the organelles and the cell, so cells must have a special process for secretion.
It turns out that there is a special mechanism that allows the passing of substances between organelles and out of the cell. This mechanism involves small carriers called vesicles, which are like little cars that take a passenger substance to its destination [1, 2]. In the next section, I will tell you about the production and action of the vesicles that take part in the secretion of molecules called proteins, which are the “workers” that drive many processes in the body.
The Secretory Pathway for Proteins
About 30% of the proteins made in the human body are secretory proteins, which are proteins designed to be secreted from cells. Like all proteins, secretory proteins are produced in sewing machine-like particles called ribosomes (the Nobel Prize in Chemistry in 2009 was awarded to three researchers who studied the structure and function of ribosomes). The ribosomes that produce secretory proteins are located on a special channel in the membrane of an organelle called the endoplasmic reticulum (ER). After secretory proteins are produced, they pass through a channel embedded in the ER membrane into a canal-like network of ER tubules that are spread around the cell. There, other proteins called coat proteins form a special structure on a small surface of the ER membrane, kind of like a dome. This dome collects the secretory proteins and then pinches off small spheres of the membrane—the vesicles—which contain the secretory protein cargo produced in the ER membrane. This process of vesicle formation, in which part of the ER membrane is pinched off, is called budding [1, 2]. After budding, coat proteins fall off the vesicles, and the naked vesicles containing the secretory proteins are passed on to an organelle called the Golgi apparatus. The Golgi sorts and directs the secretory cargo vesicles to their final destination—the cell membrane (also called the plasma membrane). In the plasma membrane, with the help of additional proteins (such as SNARE and RAB), the vesicles go through the last two stages before protein secretion—docking and fusion [3]. In these stages, the vesicles come very close to the membrane in a specific alignment, then finger-like structures on the vesicles and the membrane intertwine and pull the vesicles and the membrane toward each other, kind of like Velcro. When a vesicle and the membrane are close enough together, they fuse spontaneously, somewhat like two soap bubbles merging. Then, the secretory protein cargo is released to the other side of the membrane, outside of the cell—a process called exocytosis.
As you can see, the process of producing, transporting, and secreting proteins is quite complex. You can think of it like an automobile assembly plant, in which the automobile is put together piece-by-piece along an assembly line before it can be sent out of the plant. The “assembly line” for secretory proteins is called the secretory pathway [4], and the process by which vesicles carry substances between compartments within the cell and out of the cell is called vesicle trafficking (Figure 2). You might be surprised to know that many of the mechanisms that control vesicle trafficking in the secretory pathway have existed in cells for more than 2 billion years! This means that cells in our bodies, including the nerve cells in our brains, share basic secretion machinery with simpler organisms, such as yeasts [5, 6]. In fact, the first discoveries about the machinery that operates the secretory pathway were made using yeast [7–9]. In the next section, I will tell you about the early discoveries that were made in yeast in my lab, which earned me the Nobel Prize in Physiology or Medicine.
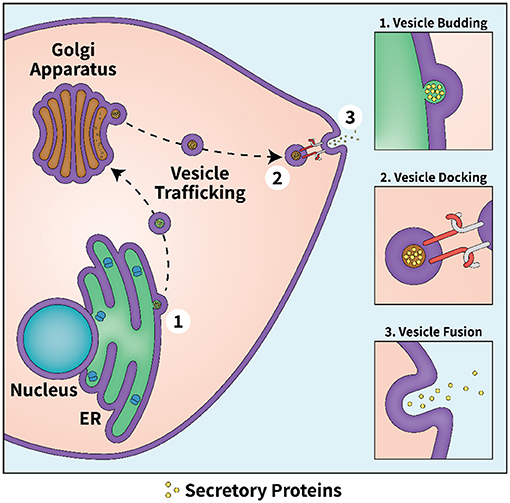
- Figure 2 - The secretory pathway.
- The secretory pathway in cells involves several steps. First, secretory proteins are produced by ribosomes on the membrane of the ER. Vesicles containing the proteins bud off from the ER membrane (step 1) and travel to the Golgi apparatus, which directs them to their final destination: the plasma membrane. The vesicle’s membrane and the plasma membrane hook together like Velcro, in a step called docking (step 2), and then fuse like two soap bubbles, releasing the vesicle’s contents to the outside of the cell (step 3).
Secretory Pathway in Yeast
When I started studying the secretory pathway with my students, there were some hypotheses about the machinery controlling vesicle trafficking, but no one had identified the genes involved in this machinery. We believed that studying the genes involved in the secretory pathway would be a powerful way to learn about vesicle trafficking. We chose to work on baker’s yeast, a simple microorganism that is used to make bread and beer. Baker’s yeast was attractive to us because we could easily grow it in the laboratory, and we had simple techniques for accurately analyzing its genes.
To identify genes related to the secretory pathway in yeast, we exposed the yeast cells to chemicals that cause random changes in the DNA, called mutations. Random mutations affect one gene randomly, out of the approximately 5,000 genes in yeasts. How, then, did we manage to identify one specific secretory pathway gene in this haystack of genes? First of all, we knew we were looking for essential genes, which are genes that cells need to survive. We knew that if we introduced a mutation into an essential gene that inactivated that gene completely, the cell would die and we would have no cells to work with. Therefore, we needed to somehow introduce a mutation that would allow the cell to stay alive long enough for us to study it. Among the many mutations that we introduced randomly, some by chance change the protein encoded by that gene to make it unstable at human body temperature. Normal yeast cells are perfectly happy at human body temperature but these particular mutations can only function at room temperature. These are called temperature-sensitive lethal mutations [10]. So, using temperature-sensitive lethal mutations, we could narrow our search down to about 1,500 essential genes out of the 5,000 total genes of yeast. This is still a large number, so we needed another parameter to help us find the genes responsible for vesicle trafficking. For that, we based our search on a hypothesis made by George Palade, a Nobel Prize winner who pioneered the study of the secretory pathway. Palade hypothesized that secretion is linked to cell growth through vesicle fusion. That is a logical assumption because when vesicles fuse with the plasma membrane, the membrane should get bigger. This allowed us to use another search parameter in our study—we looked for mutations that prevented the yeast cells from getting bigger, and that also caused secretory proteins to build up inside the cells.
Specifically, we were searching for the piling up of a protein called invertase, which is the enzyme that breaks sucrose (table sugar) into two smaller sugars—fructose and glucose. Normally, invertase is found in the cell wall of yeast cells, so when the secretion process works properly, we expect to see the same amount of fructose and glucose products when we incubate whole cells in sucrose as we do when we break the cells open and incubate the pieces in sucrose. However, when the secretion process does not function properly and invertase piles up inside the cells, we should get a larger amount of fructose and glucose when we incubate broken cells compared to whole cells. That is the exact result we got in an experiment led by my brilliant student Peter Novick, which we published in 1979 [7]. We called the gene that was changed by this mutation sec1. When we examined cells with mutated sec1 using a powerful microscope called an electron microscope, we saw that many vesicles containing invertase had accumulated inside the cell (Figure 3A). This was very exciting—it was the first time that vesicle accumulation inside a cell was ever seen. This finding also supplied strong evidence to support George Palade’s hypothesis that secretion and cell growth are related. From there, we had to keep working to understand the specific role that the sec1 protein (produced from the instructions in the sec1 gene) played in vesicle trafficking.
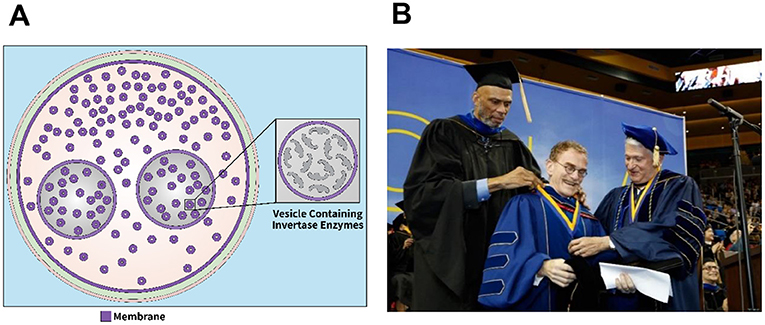
- Figure 3 - The Nobel Prize-winning discovery of Sec1 in Baker’s yeast.
- (A) The discovery that led to the Nobel Prize involved the piling up of vesicles containing a protein called invertase, inside a baker’s yeast cell with a mutation in a gene called sec1 (image adapted from [7]). (B) After I won the Nobel Prize, I was invited by my colleagues at UCLA, where I received my undergraduate degree, to give the commencement address. I arrived at the very same pavilion where my basketball idol, Kareem Abdul Jabbar (previously named Lewis Alcindor), used to play. He introduced me in front of 20,000 people, and then placed an honorary medal around my neck. This was a surreal moment that I will never forget (Image credit: UCLA).
Eventually, we found out that the sec1 protein is involved in the final step of the secretion process, namely docking and fusion. Soon after we identified the sec1 mutant, we identified more mutants involved in the yeast’s secretion process [8], and we began to understand the order of the events that occur in the secretory pathway of yeast [9]. Our discoveries of genes involved in the yeast secretory pathway also advanced the understanding of how this process occurs in mammals, including humans [6]. More specifically, many of the genes and proteins we discovered were found to have counterparts in mammalian cells. One of the leading scientists who found some of the counterparts of the genes we discovered is Prof. James Rothman, with whom I shared the Nobel Prize in Physiology or Medicine in 2013. After winning the Nobel Prize, I even got to meet my basketball idol Kareem Abdul Jabbar! (Figure 3B).
Our work was pioneering for two reasons: first, due to our specific findings of genes involved in the secretory pathway; but also due to our novel, successful use of microbial genetics to study the secretory pathway. Our findings opened a new line of research, which many of my students and their students have followed over the years. Once the secretory pathway in yeast was well-understood, people started using that knowledge for medical applications. One important example in which I took part is the use of the yeast secretion pathway to produce human insulin to treat diabetes [10]. As secretion is such a fundamental process in the human body, there are many more examples of medical conditions that could be treated based on our increasing understanding of the secretory pathway—such as heart diseases and digestion problems. Though I never intended to be involved in the development of medical applications, I am glad that our work contributes to treatments that help people all over the world.
Recommendations for Young Minds
I think that one of the most fundamental traits that a scientist should have and try to develop is the excitement of discovery. In my view, science is the process of discovery—not just a list of facts about what is already known and established. If you have a passion for science, you should find an independent way of exploring—do not limit yourself to the material you learn in class. When it comes to expanding your knowledge, I believe that independent study such as in a laboratory is just as important as reading from textbooks. To understand whether you can be a good research scientist, you must experience research yourself. Research is frequently very frustrating—lots of things fail and you must learn to persevere in the face of constant failure. You can only equip yourself for that by experiencing laboratory research firsthand. This is also how you can develop faith in yourself and in your skills, which will allow you to believe that things can work out and that you can reach your goal, even when the process is very complicated. That is why I advise every young student to start working in the lab or in some form of independent study straight away.
Another aspect of a good scientist is the ability to identify an exciting problem to study and to fully commit to it. This means that you must keep focusing on that specific problem and think independently about the design and execution of experiments, observation or theory that can help you solve it. Even if you experience challenges along the way, you should keep thinking about the problem, turning it over in your head until you find a new way to approach it. I know that focus can be hard, especially for creative people who have many new ideas all the time. But each of us has only a certain amount of time and energy, and if we do not focus, we are not able to make good progress. Therefore, my advice is to keep your eyes on the prize and persevere until you find a solution to one important problem to which you have decided to dedicate yourself.
Additional Materials
- A pathway of a hundred genes starts with a single mutant: Isolation of sec1-1.
- Publishing important work in the life sciences: Randy Schekman at TEDxBerkeley.
- Rothman and Schekman: Uncovering the Secretory Pathway - Youtube.
Glossary
Secretion: ↑ A critical process by which cells release substances, such as hormones and enzymes, in a controlled way.
Eukaryotes: ↑ Organisms whose cells contain a nucleus.
Vesicles: ↑ Membranous sacs that carry substances inside the cell.
Endoplasmic Reticulum (ER): ↑ The organelle in which secretory proteins are produced and placed into vesicles.
Budding: ↑ A process where part of the ER membrane is pinched off and form a vesicle.
Exocytosis: ↑ A process where a substance is released from within the cell to the outside environment by the fusion of a vesicle with the cell’s plasma membrane.
Secretory Pathway: ↑ The pathway used to secrete proteins from inside the cell to the outside environment. It includes organelles such as the ER and Golgi apparatus, as well as the cell membrane and vesicles.
Vesicle Trafficking: ↑ The process in which vesicle carry substances between compartments in the cell and between the cell and its outer environment.
Temperature-Sensitive Lethal Mutations: ↑ Cells that act normally at low temperatures but die at higher temperatures.
Cell Wall: ↑ The outermost layer of the cell that covers the cell’s membrane.
Acknowledgments
I wish to thank Noa Segev for conducting the interview which served as the basis for this paper, and for co-authoring the paper, and Netta Kasher for providing the figures.
Conflict of Interest
The author declares that the research was conducted in the absence of any commercial or financial relationships that could be construed as a potential conflict of interest.
References
[1] ↑ Jena, B. P. 2007. Secretion machinery at the cell plasma membrane. Curr. Opin. Struct. Biol. 17:437–43.? doi: 10.1016/j.sbi.2007.07.002
[2] ↑ Schekman, R., and Orci, L. 1996. Coat proteins and vesicle budding. Science 271:1526–33. doi: 10.1126/science.271.5255.1526
[3] ↑ Verhage, M., and Sørensen, J. B. 2008. Vesicle docking in regulated exocytosis. Traffic 9:1414–24. doi: 10.1111/j.1600-0854.2008.00759.x
[4] ↑ Shikano, S., and Colley, K. J. 2013. “Secretory pathway,” in Encyclopedia of Biological Chemistry, ed J. Jez (New York, NY: Elsevier). p. 203–9. doi: 10.1016/b978-0-12-378630-2.00507-7
[5] ↑ Bennett, M. K., and Scheller, R. H. 1993. The molecular machinery for secretion is conserved from yeast to neurons. Proc. Natl. Acad. Sci. 90:2559–63. doi: 10.1073/pnas.90.7.2559
[6] ↑ Rothman, J. E., and Orci, L. 1992. Molecular dissection of the secretory pathway. Nature 355:409–15. doi: 10.1038/355409a0
[7] ↑ Novick, P., and Schekman, R. 1979. Secretion and cell-surface growth are blocked in a temperature-sensitive mutant of Saccharomyces cerevisiae. Proc. Natl. Acad. Sci. 76:1858–62. doi: 10.1073/pnas.76.4.1858
[8] ↑ Novick, P., Field, C., and Schekman, R. 1980. Identification of 23 complementation groups required for post-translational events in the yeast secretory pathway. Cell 21:205–15. doi: 10.1016/0092-8674(80)90128-2
[9] ↑ Novick, P., Ferro, S., and Schekman, R. 1981. Order of events in the yeast secretory pathway. Cell 25:461–9. doi: 10.1016/0092-8674(81)90064-7
[10] ↑ Schekman, R. A. N. D. Y. 2013. Genes and Proteins That Control the Secretory Pathway. Nobel Lecture, 7.