Abstract
There are online videos that appear to show electrically powered prosthetic (artificial) hands to be near-perfect replacements for a missing hand. However, for many users, the reality can be quite different. Prosthetic hands do not always respond as expected, which can be frustrating. A prosthetic hand is controlled by muscle signals in the remaining part of the person’s affected arm, using sensors called electrodes. The electrodes are embedded within the socket, which is the part of the prosthetic arm that connects it to the person’s arm. When they activate their muscles, the hand can open, close, or change its grip. If the socket moves, it can pull the electrodes away from the skin. As a result, the muscle activity signaling the person’s intention cannot be properly detected, and the hand will not work very well. In this article, we explain why socket fit may be the most important part of a prosthetic arm.
Introduction
Imagine you are carrying a drink and your hand suddenly opens, or that you are reaching for your glass and your hand will not open. It is not hard to see how disruptive it is when you cannot rely on your hands to do what you want. Unfortunately, this is the reality for some prosthesis users. A prosthesis is an artificial (prosthetic) body part. In this article we are referring primarily to prosthetic hands.
When someone is missing a hand (either from being born without one or losing it through amputation) they are often provided with a prosthesis. The prosthesis is usually designed to look like a real hand, and it aims to provide some of the capabilities of the missing hand.
There are three common types of prosthetic hands [1–4].
- Passive—the hand can either be rigid, or capable of being shaped open or closed using the other hand, to help perform tasks;
- Body-powered—uses shoulder or arm movements to pull a cable and make the hand open or close; and
- Externally powered—uses batteries to actively open and close the hand; most are controlled by muscle signals (Figure 1).
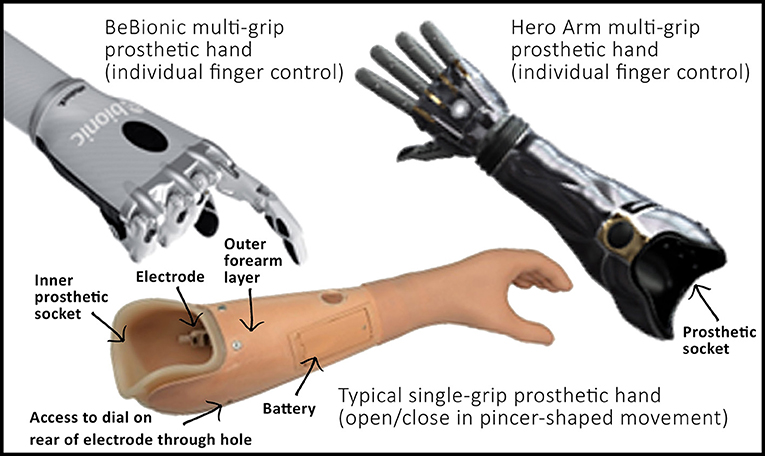
- Figure 1 - Examples of myoelectric prosthetic arms.
- Some hands simply open and close in a pincer-shaped movement. Others have individual control over each finger, allowing the hand to form different grasp shapes (for example, a pointed finger allowing you to click a computer mouse button). You can see that the hand is connected to a forearm section (outer layer), which has an inner socket for the person to slot their arm into. This inner socket is molded based on the person’s own arm, either using a plaster cast mold or a scanner and computer software.
In this paper we focus on the third type, which are called myoelectric prostheses. Online videos sometimes give the impression that myoelectric prostheses are a near-perfect replacement1. But, for many users, they do not always do what is expected; sometimes myoelectric prostheses open when they should not, or do not open when they should.
How Does A Myoelectric Prosthesis Work?
Myoelectric prosthetic hands rely on small electrical signals generated by the muscles (“Myo” comes from the Greek word for muscle). When you want to move part of your body, signals are sent from your brain to the skeletal muscles, through nerves. This triggers a chemical reaction that causes the muscle to shorten or contract (you may be familiar with the term “flexing” a muscle). As a side effect, a small amount of electrical current flows through the muscle, which can be detected by metal plates (called electrodes) placed on the skin. If the electrodes sense that the person is attempting to contract the muscle, they can activate the prosthetic hand to move. You may be surprised to learn that this technology has been around since 1944 [5], although there have been many advances in the design of prosthetic hands since then.
How Do We Detect Muscle Signals?
Figure 2 shows an electrode used in a prosthetic arm. The three metal plates sit against the skin to capture the electric signal. They work best when a layer of sweat has built up between them and the skin, reducing the electrical resistance. Inside, there are electronics that clean up and magnify the electric signal.
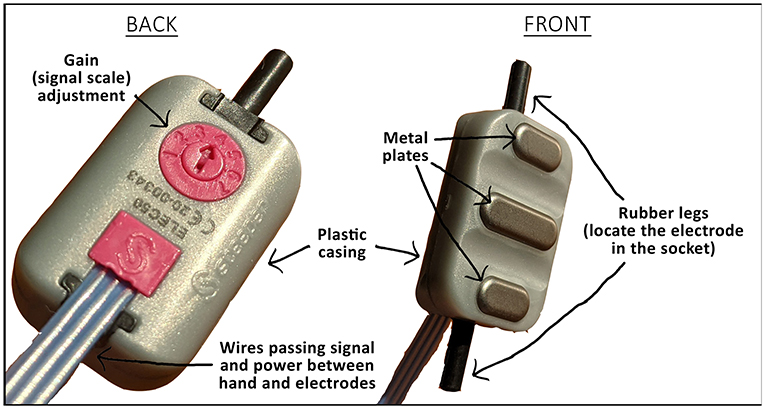
- Figure 2 - An electrode used to control a myoelectric prosthetic hand.
- The three metal plates must keep a good level of contact with the skin to conduct the signal. On the back of the electrode is a dial that allows the scale of the signal to be adjusted, like adjusting the volume on a TV or the sensitivity on a video game controller. There are also wires that transmit the signal to the controller in the hand. The black rubber “legs” hold the electrode in the socket.
Professionals trained to make well-fitting prostheses are called prosthetists. A prosthetist takes a plaster cast of the person’s arm and creates a 3D model of it. Then, working with a technician, a prosthetic socket is built using this model (usually from a semi-rigid material such as plastic or carbon fiber). The socket is the part of the prosthesis that the person slips their arm into (Figure 1). The socket can be designed to be tighter around certain parts of the arm, to hold it in place, and looser around other, more sensitive parts (such as scar tissue or the area over bones); this is a bit like fitting a shoe. It is very important for the prosthetist to get the right fit.
The electrodes are held in place in the prosthetic socket by little rubber legs (Figures 2, 3). During normal use, the socket can move around slightly over the muscles and bones. Since the electrodes are connected to the socket, they may move around too. If the socket is loose and moves around too much, the electrodes may be dragged to a position where they are less able to pick up the muscle signals, or they may lose contact with the skin completely.
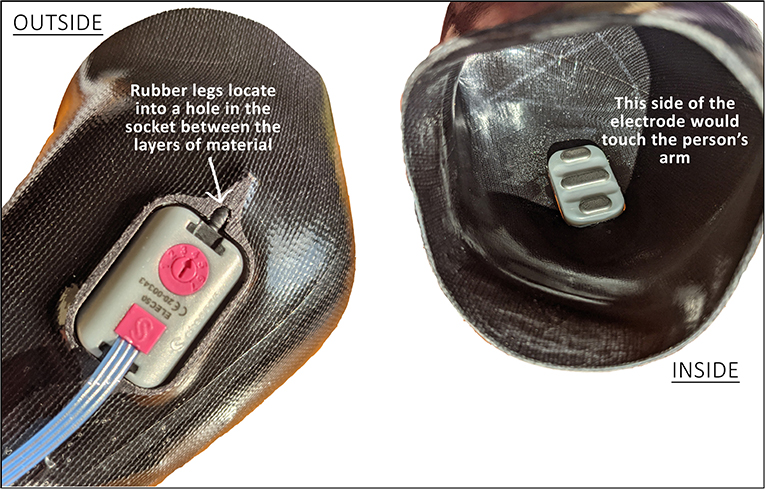
- Figure 3 - The electrodes fit into a hole in the inner socket that is shaped around the person’s arm.
- From the outside (left image) you can see one of the black rubber “legs” that slot into the material to hold the electrode in place. The outer, forearm-shaped layer (shown in Figure 1) connects over the top of this inner socket, covering the back of the electrode.
Why Is Movement of the Electrodes a Problem?
We live in an electrically “noisy” environment, surrounded by power lines and radio signals. The human body acts like an antenna, picking up electricity and becoming charged. That is why we sometimes get static shocks. These noisy signals on the surface of the skin can be up to a thousand times larger than muscle signals [5]. Therefore, the electrode’s electronics must be able to filter them out.
If the contact between the skin and the electrodes changes, the filter does not work as well. The unwanted noisy signals may be mistaken for a muscle contraction, causing the prosthetic hand to open or close unexpectedly. This could cause someone to drop something or grip something without warning. If the electrode loses contact with the skin completely or moves so that it is no longer above the correct muscle, it may also be unable to detect intended signals. This may leave someone stuck holding onto something. There is a good example in Tara Simms PhD thesis where a student was embarrassed that they could not let go of their headmaster’s hand after shaking it [6]. This unpredictability can limit how much the person trusts the prosthesis and how well they can control it.
Effects of Unexpected Hand Movements
To understand how common the problem of unexpected hand movements is, data were collected from 20 people in the United Kingdom, wearing prostheses provided to them by the National Health Service [7]. They were asked to move their arm up and down, as if reaching up to a shelf or down into a drawer. During the movements (24 in total) they did not attempt to open or close their prosthetic hand. Seventy percent (14 people) experienced their prosthetic hand moving when it should not be.
If the hand opens/closes when it should not, although frustrating, does it actually relate to how well someone can use their prosthesis? To measure this, we asked the prosthesis wearers to do a simple task: pick up a cylinder and put it into a tube. We recorded whether the task was completed smoothly (without dropping the cylinder), how long it took, and where they looked. We found that people whose hands opened/closed unexpectedly in the previous task were also less successful at and slower to perform the cylinder task. They also spent more time looking at the hand, which suggested that they did not have much confidence in its performance.
We wanted to explore how people chose to use their prostheses in their daily lives. For 1 week, participants wore a small sensor, like a Fitbit or the sensors in a smart watch, on each wrist, to measure their arm movement. We used these sensors to calculate how many hours the prosthesis was worn and how much each arm was used. For someone with both arms and no health problems, we observed about 50% use of each arm. With prosthesis users wearing their prostheses, many were still around 80% reliant on their unaffected arm. This means that, even though they were wearing prostheses designed to help them complete everyday tasks, they chose to use their healthy arms instead.
Interestingly, the number of times the hand activated unexpectedly in our earlier laboratory study had no relation to the amount of time people wore their prostheses at home, or how reliant they were on their prostheses. This suggests that some people may put up with an unpredictable hand if it can help them to achieve their other goals.
Possible Ways to Reduce Electrode Movement
With the current design of the electrodes and the socket of prosthetic arms, it is unlikely that we will be able to remove unwanted movements. People are looking into other solutions. One rather complex and expensive solution is to implant the electrodes beneath the skin, in the muscle itself [8–10]. Other solutions involve placing the electrodes within a stretchy, elastic sock, which would hold them against the skin [11]. Other new ways of holding the electrodes in the socket to prevent them from moving around as the socket moves are also being developed [12]. Some of these new technologies need more research before they can become widely available.
Summary
In summary, we have described some upper-limb prostheses, including myoelectric devices. We have explained how these are controlled and used some of our research studies to illustrate the problems which can be experienced by users in the real world. Finally, we have introduced some of the ideas which are being investigated to overcome those problems. Whatever the solution, we hope we have convinced you how important it is to get a good socket fit, so that the signals generated by the user can be reliably detected by the electrodes.
Glossary
Prosthesis: ↑ A prosthesis is an artificial (prosthetic) body part. You may also see this used in the text in the plural form, prostheses.
Amputation: ↑ Amputation is the surgical removal of a limb. This may occur following an accident or due to certain illnesses.
Rigid: ↑ Something rigid is not flexible, it cannot be bent out of shape. Semi-rigid means that it has some flexibility to it, but will still hold its shape well.
Myoelectric Prosthesis: ↑ A myoelectric prosthesis uses electrical signals generated within the muscles, to control the movement of a prosthetic hand.
Electrodes: ↑ Electrodes involve metal plates which conduct the electrical signals generated in the muscles, and electronics which clean up and magnify the signals. The signal can then be sent to a controller to tell the prosthetic hand how to move.
Prosthetist: ↑ A prosthetist is a clinically trained professional who works with a person who has lost a limb. They guide the person in choosing the right prosthesis, and design, make and fit the socket.
Conflict of Interest
The authors declare that the research was conducted in the absence of any commercial or financial relationships that could be construed as a potential conflict of interest.
Acknowledgments
The authors would like to acknowledge help from Charlie Prince (age 13) who read through the manuscript and provided us with useful feedback relating to the language we were using.
Footnote
1. ↑For an example, see https://youtu.be/F_brnKz_2tI.
References
[1] ↑ Engels, L., and Cipriani, C. 2019. Nature’s masterpiece: how scientists struggle to replace the human hand. Front. Young Minds. 7:83. doi: 10.3389/frym.2019.00083. Available online at: https://kids.frontiersin.org/articles/10.3389/frym.2019.00083
[2] ↑ Plettenburg. D. H. 2006. Upper Extremity Prosthetics: Current Status & Evaluation, 1st Edn. Delft: VSSD. ISBN: 978-9-071-30175-9.
[3] ↑ Muzumdar, A. 2004. Powered Upper Limb Prostheses - Control, Implementation and Clinical Application, 1st Edn. Berlin: Springer. ISBN: 978-3-642-62302-8
[4] ↑ Maat, B., Smit, G., Plettenburg, D., and Breedveld, P. 2018. Passive prosthetic hands and tools: a literature review. POI 42, 66–74. doi: 10.1177/0309364617691622. Available online at: https://journals.sagepub.com/doi/full/10.1177/0309364617691622
[5] ↑ Childress, D. 1992. Upper-Limb Prosthetics: Control of Limb Prostheses. Chapter 6D - Atlas of Limb Prosthetics: Surgical, Prosthetic, and Rehabilitation Principles, 2nd Edn. American Academy of Orthopedic Surgeons. Available online at: http://www.oandplibrary.org/alp/chap06-04.asp
[6] ↑ Simms, S. 2014. A Participatory Design Approach to Developing Upper Limb Prostheses for Children and Young People. (PhD Thesis), University of Southampton, Southampton, United Kingdom. Available online at: https://eprints.soton.ac.uk/367055
[7] ↑ Chadwell, A., Kenney, L., Thies, S., Head, J., Galpin, A., and Baker, R. 2021. Addressing unpredictability may be the key to improving performance with current clinically prescribed myoelectric prostheses. Sci. Rep. 11:3300. doi: 10.1038/s41598-021-82764-6. Available online at: https://www.nature.com/articles/s41598-021-82764-6#citeas
[8] ↑ Salminger, S., Sturma, A., Hofer, C., Evangelista, M., Perrin, M., Bergmeister, K., et al. 2019. Long-term implant of intramuscular sensors and nerve transfers for wireless control of robotic arms in above-elbow amputees. Sci. Robot. 4:aaw6306. doi: 10.1126/scirobotics.aaw6306 Available online at: https://www.science.org/doi/10.1126/science.abb1073
[9] ↑ Nathan, S. 2019. Improving control of amputees’ prostheses with implanted electrodes. Engineer. Available online at: https://www.theengineer.co.uk/improving-control-prostheses-implanted-electrodes/
[10] ↑ Zhang, J., and Hoshino, K. 2019. Molecular Sensors and Nanodevices: Principles, Designs and Applications in Biomedical Engineering. Chapter 8 - Implantable and Wearable Sensors, 2nd Edn. Cambridge, MA: Academic Press. ISBN: 978-0-128-14862-4. Available online at: https://www.sciencedirect.com/science/article/pii/B9780128148624000089
[11] ↑ Reissman, T., Halsne, E., Lipshutz, R., Miller, L., and Kuiken, T. 2018. A novel gel liner system with embedded electrodes for use with upper limb myoelectric prostheses. PLoS ONE 13:e0198934. doi: 10.1371/journal.pone.0198934. Available online at: https://journals.plos.org/plosone/article?id=10.1371/journal.pone.0198934
[12] ↑ Head, J., Kenney, L., Nazarpour, K., Dyson, M., Varley, T., and Howard, D. Grant From the National Institute for Health Research Invention for Innovation (2020-2023). A Smart Electrode Housing to Improve the Control of Upper Limb Myoelectric Prostheses. ID: NIHR201310. Available online at: https://fundingawards.nihr.ac.uk/award/NIHR201310