Abstract
What can you do when you feel hungry? You could go to the kitchen and prepare a sandwich, or you could buy yourself a snack. But what if you are fixed to the ground? Plants are champions at reaching food that is far away from them while they are standing still. Below a plant, roots branch out into the soil in many directions, looking for the nutrients plants need for survival and growth. Iron is a very important nutrient for plants because it is essential for growth and development, and it also helps plants to face stresses in the environment. Even if iron availability in soil is very low, plants have developed two strategies for efficiently taking up iron and storing it. In this article, we will explore the importance of iron in plants’ lives, explaining how plants take up it and how balanced iron levels are important for plants’ (and our) survival.
What Do Plants Eat?
Like animals, plants also need to “eat:” “mineral nutrition” is how plants acquire and absorb essential inorganic ions. Plants take up such nutrients from the soil using their roots. Importantly, microorganisms like fungi and bacteria living either in the nearby soil (called the rhizosphere) or inside plants’ roots can sometimes help the plants obtain nutrients. Certain nutrients are very important for plants’ survival, and a shortage of them can cause serious problems: indeed, nutrients, along with sunlight, are required to produce everything that plants need for their life. We can classify nutrients into two main groups: “macronutrients” if plants need them in large quantities and “micronutrients” which are only needed in small amounts.
Iron (Fe) is an essential micronutrient for many organisms, including humans: indeed, without iron, we would not have hemoglobin, which is the protein that transports oxygen in the blood. Iron also plays a key role in plants, where it participates in vital processes like photosynthesis. If plants do not get enough nutrients, they start to get “sick,” and you can even see their sickness from their leaves. For example, a plant’s leaves can become pale green/yellow (a phenomenon called “chlorosis”) if they lack iron (Figure 1): cells located far from the veins (“interveinal cells”) get the lowest amount of iron and become very pale (“chloros” means light green/yellow in ancient Greek). The lack of iron limits a plant’s ability to perform photosynthesis and to produce the right amount of chlorophyll, which is the pigment that gives the green color to leaves.
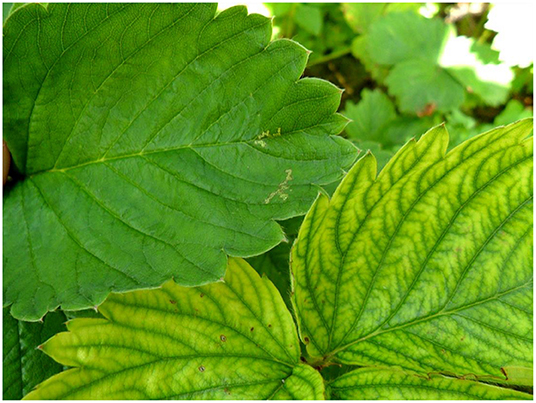
- Figure 1 - A chlorotic strawberry leaf (right) compared to a healthy one (left).
- Iron deficiency causes problems with photosynthesis and the production of chlorophyll. A lack of chlorophyll makes leaves appear light green or yellow instead of healthy green (photo credit: Paolo Guarinoni).
Iron is one of the most important nutrients for plants, and its absence dramatically affects crop growth [1]. Despite iron’s abundance in soil, its availability to plants is very reduced due to its limited solubility (iron is normally present in the chemical form Fe3+; however, this chemical form poses problems in Fe uptake as it is not readily available to plants). For this reason, plants have developed two different strategies for the uptake of this micronutrient.
How Do Plants Acquire Iron?
Non-grass plants, such as tomato (whose scientific name is Solanum lycopersicum), take up iron using Strategy I, while grass-type crops, such as Zea mays (maize) and Triticum aestivum (bread wheat), use an alternative strategy named Strategy II (Figure 2) [2]. Both strategies are based on well-regulated molecular mechanisms involving two main actors: the root (particularly the plasma membrane of its cells) and the rhizosphere (the soil closest to roots). Special proteins called “transporters” are located in the plasma membranes, and these transporters help plants to move molecules either into or out of the roots as needed: plants could not “eat” without transporters, since they could not import nutrients inside their roots! You can imagine the transported molecules as cars and the transporters as tunnels that the cars use to get through mountains. These tunnels are often “one way,” which means that they allow molecules to move either into the cells or out of them, but not both.
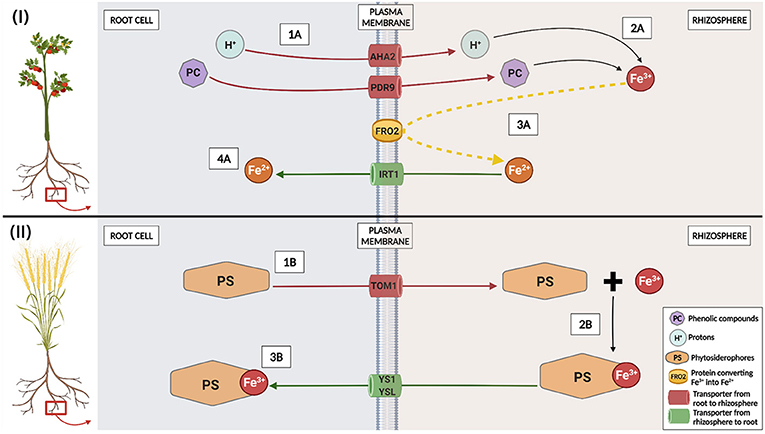
- Figure 2 - Strategies adopted by plants to take up iron from soil.
- Top—Strategy I (adopted by non-grass plants like tomato): phenolic compounds PC and protons H+ exit from roots using transporters AHA2 and PDR9, respectively (1A, red arrows) and, in the rhizosphere, they increase iron Fe3+ levels (2A). Fe3+ is converted into Fe2+ by the plasma membrane protein FRO2 (3A, yellow dashed arrow). Fe2+ enters roots using transporter IRT1 (4A, green arrow). Below—Strategy II (used by grass plants like wheat): phytosiderophores PS exit from roots using transporter TOM1 (1B, red arrow); in the rhizosphere, they form a complex together with Fe3+ (2B). The complex PS-Fe3+ enters roots by YS1 or YSL transporters (3B, green arrow).
In soils, iron in the chemical form of Fe3+ is not readily available to plants because it forms chemical complexes which are poorly soluble and cannot be easily absorbed. Figure 2 shows strategies adopted by plants to overcome this problem and acquire iron. In Strategy I plants, protons H+ and phenolic compounds (PC in Figure 2) are transported out from roots to the soil by using the transporters named AHA2 (H+-TRANSLOCATING P-TYPE ATPase-2) and PDR9 (PLEIOTROPIC DRUG RESISTANCE 9; step 1A in Figure 2): both H+ and phenolic compounds increase Fe3+ solubility (step 2A). AHA2 and PDR9 are “pumps,” which means they are energy-driven transporters. Then, FRO2 (FERRIC REDUCTION OXIDASE 2), a protein localized inside the plasma membrane, converts iron Fe3+ into Fe2+ (step 3A): in such form, iron can be transported by IRT1 (IRON-REGULATED TRANSPORTER 1) inside plants’ roots (step 4A). In Strategy II plants, there is no conversion of Fe3+ into Fe2+: phytosiderophores (PS in Figure 2) are transported out from roots by TRANSPORTER OF MUGINEIC ACID 1 (TOM1; step 1B). Once in the rhizosphere, PS are able to directly bind iron in the form of Fe3+ (step 2B). The complex PS-Fe3+ can finally enter roots through YS1 (YELLOW STRIPE 1) or YSL (YELLOW STRIPE LIKE) transporters (step 3B). Although these different details on how iron is carried inside roots, both strategies are turned on once plants start lacking iron. Moreover, roots’ shape changes a lot under iron deficiency conditions! The number of root branches increases, and roots become richer in root hairs, helping plants to take up iron from soil.
Iron As A Superhero
Once iron enters root cells, it can be transported far from roots to be used in different parts of the plant. If cells “feel” that an excess of iron is present, iron is safely stored within a “big” protein called “ferritin,” formed from 24 subunits arranged in a form of a cage and that can house iron in its central hollow cavity. Interestingly, iron can also help plants to fight stresses: indeed, plants are fixed to the ground, and they cannot run away when some environmental conditions become harsh for them or enemies attack. The right supplies of iron can help plants to face environmental problems and to cope with pathogens or insects.
Fungi are among the worst plant pathogens, as they devastate cultivations all over the world and cause extensive yield losses. Iron seems to control and reduce many fungal diseases in wheat and barley, such as the ones caused by rusts fungi, pathogens that are not dangerous for us but are terrible for many economically important plants [3]. Plant defense strategies can be really complicated! In some cases, iron deficiency can help pathogens to infect plants, but in other cases, iron deficiency can help plants to fight their enemies. Pathogens also need nutrients to survive, and they try to steal the iron they need from plants: in some cases, plants reduce iron levels at the site where pathogens have attacked, trying to keep on fighting and preventing pathogens to use their nutrients [4, 5].
Iron in plants is not just a superhero for the plants themselves. Many useful bacteria and fungi in the rhizosphere are positively influenced by the iron levels in plants, and iron deficiency can cause big problems in these important communities [4]. Moreover, iron in plants can also help all of us: when we do not get enough micronutrients from our diets, we may become sick! Micronutrient deficiency is also known as “hidden hunger” because, even if you feel full after a meal, you may not be getting sufficient nutrients from your food. Iron deficiency is the most widespread micronutrient deficiency in humans all over the world, and it causes a blood disease called “iron deficiency anemia” (IDA). According to the World Health Organization (WHO), more than 1.5 billion people suffer from IDA, which is around 25% of the worldwide population! Unfortunately, children and teenagers are among the most affected [6]. For this reason, scientists are studying how plants acquire and accumulate nutrients so that they can try to increase the amounts of nutrients in plants: “biofortification” is exactly the process of increasing the nutrients’ content of plants by genetic means and not by direct supplementing of food [7]. An example of a biofortified crop with high levels of iron is the “iron bean,” which was recently introduced in developing countries of Asia, South America, and Africa [8].
The Dark Side of Iron
Since iron seems to be so helpful, you might wonder why plants do not accumulate as much iron as possible to avoid the consequences of iron deficiency. Unfortunately, too much iron can be very dangerous to plants, weakening and eventually killing them. Iron levels must be well-controlled to prevent both deficiency and excess. Just imagine iron in plants as a sort of “Dr. Jekyll and Mr. Hyde” or think about iron levels for plant health as a “libra scale:” equilibrium is good, while extremes are deadly (Figure 3).
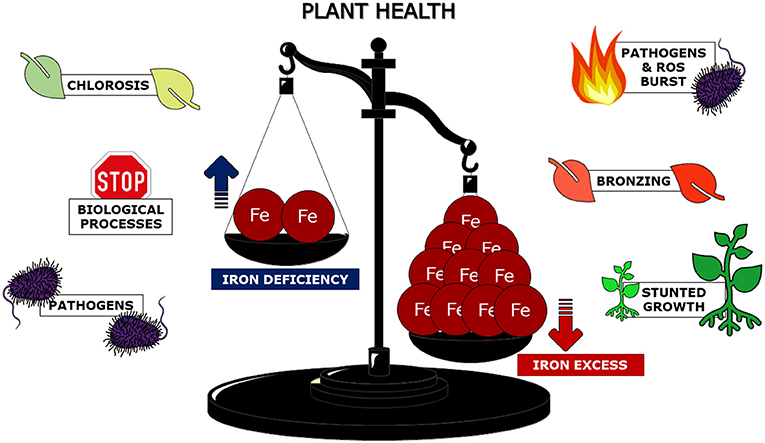
- Figure 3 - The proper balance of iron within plant cells is necessary for plant health.
- Both iron deficiency and iron excess can affect the health of plants, interfering with their abilities to face environmental stresses, to grow, and to survive.
Iron deficiency can dramatically weaken plants, but iron excess causes “stunted growth,” which is when plants appear small and sick, and can also cause “bronzing of leaves,” which is when leaves turn brownish-red. These dramatic events are linked to the generation of Reactive Oxygen Species (ROS), unstable oxygen-containing molecules that can damage many cellular molecules and that can cause cell death. Indeed, iron participates in the production of these dangerous molecules [5].
Conclusion
The world’s population is growing. Increasing the amount of iron in plants could help improve human health through better diets. We have begun to understand the importance of iron (and other nutrients) in plants, and we have seen how iron deficiency and iron excess can both negatively affect plants’ lives. In the future, there are sure to be many new scientific findings explaining how plants take up nutrients and how we could produce nutrient-rich crops. This research will benefit people, especially poor people, all over the world and will help us to defeat the “hidden hunger” of iron once and for all.
Glossary
Inorganic Ion: ↑ An atom or a molecule with a positive or negative charge needed for vital cellular activity.
Rhizosphere: ↑ The area of soil around roots colonized by bacteria and fungi.
Plasma Membrane: ↑ Simply known as “membrane,” it is the thin barrier, made out of lipids, between the inside and the outside of a cell.
Phenolic Compounds: ↑ Chemical compounds characterized by an aromatic ring with an -OH group collectively called “phenol.”
Phyto-siderophores: ↑ Organic compounds produced by plants under nutrient deficiency conditions and used to favor the uptake of nutrients in the rhizosphere.
Root Hairs: ↑ Hairlike structures developed by roots to help in the acquisition of water and minerals from the soil.
Conflict of Interest
AM was employed by the company Enerzyme Srl.
The remaining authors declare that the research was conducted in the absence of any commercial or financial relationships that could be construed as a potential conflict of interest.
Acknowledgments
Figures were created using BioRender (Figure 2) or modifying Servier Medical Art templates (which are licensed under a Creative Commons Attribution 3.0 Unported License; https://smart.servier.com) and using PowerPoint (Microsoft Office 365; Figure 3). All authors deeply thank Paolo Guarinoni for giving the photo of Figure 1 to PM, and all the reviewers for their work and effort to improve this paper. FM sincerely acknowledges Alessandro for his critical reading of the manuscript.
References
[1] ↑ Rout, G. R., and Sahoo, S. 2015. Role of iron in plant growth and metabolism. Rev. Agric. Sci. 3:1–24. doi: 10.7831/ras.3.1
[2] ↑ Kobayashi, T., and Nishizawa, N. K. 2012. Iron uptake, translocation, and regulation in higher plants. Annu. Rev. Plant Biol. 63:131–52. doi: 10.1146/annurev-arplant-042811-105522
[3] ↑ Dordas, C. 2017. Role of nutrients in controlling the plant diseases in sustainable agriculture. Agric. Important Microb. Sustain Agric. 2:217–62. doi: 10.1007/978-981-10-5343-6_8
[4] ↑ Verbon, E. H., Trapet, P. L., Stringlis, I. A., Kruijs, S., Bakker, P. A. H. M., and Pieterse, C. M. J. 2017. Iron and immunity. Annu. Rev. Phytopathol. 55:355–75. doi: 10.1146/annurev-phyto-080516-035537
[5] ↑ Naranjo-Arcos, M. A., and Bauer, P. 2016. “Iron nutrition, oxidative stress, and pathogen defense,” in Nutritional Deficiency, eds P. Erkekogu and B. Kocer-Gumusel (Intech Open). p. 63–98. doi: 10.5772/63204
[6] ↑ De Benoist, B., McLean, E., Cogswell, M., Egli, I., and Wojdyla, D. 2008. Worldwide prevalence of anaemia 1993-2005. Public Health Nutr. 12:444–54. doi: 10.1017/S1368980008002401
[7] ↑ Murgia, I., Arosio, P., Tarantino, D., and Soave, C. 2012. Biofortification for combating “hidden hunger” for iron. Trends Plant Sci. 17:47–55. doi: 10.1016/j.tplants.2011.10.003
[8] ↑ Mulambu, J., Andersson, M., Palenberg, M., Pfeiffer, W., Saltzman, A., Birol, E., et al. 2017. Iron beans in Rwanda: crop development and delivery experience. Afri. J. Food Agric. Nutr. Dev. 17:12026–50. doi: 10.18697/ajfand.HarvestPlus010