Abstract
The world around us is filled with odors (smells), some pleasant, calming, or bringing back memories; others stimulating, scary, or disgusting. How many odors do you think you can recognize? You might be surprised to know that humans can recognize hundreds of thousands of different odors—not an easy task to accomplish. So, how do we do it? In this article, we will smell our way through the olfactory system, look at the connections between the nose and the brain, and see how odors are processed in the brain to evoke unique responses.
Professor Richard Axel won the Nobel Prize in Physiology or Medicine in 2004, jointly with professor Linda B. Buck, for their discoveries of odorant receptors and the organization of the olfactory system.
How Do We Smell Things?
When you see a beautiful bouquet of flowers or pass by a perfume store, you often lean in to smell them. Have you ever wondered what it is, exactly, that you are smelling? And how do you recognize the smell? When you smell a flower, you inhale molecules that are released from the flower and then build an internal representation of the flower’s odor, through the electrical activity that happens in the brain (Figure 1).
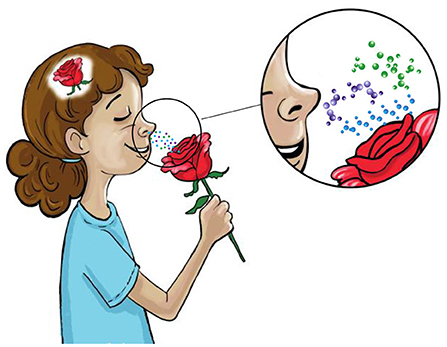
- Figure 1 - Representation of smelling.
- A variety of chemicals (odor molecules) are released from the rose to the air. These molecules reach the nose, where they activate electrical signals that are transmitted to the brain. In the brain, multiple regions are electrically activated. This activity enables us to identify a particular scent—in this case, that of a rose.
Before we dive into the complexities of smelling, let us look at how the olfactory system works. Odors are composed of molecules that are released from the object you smell (like an orange or a rose). The odor molecules, which are called odorants, travel through the air and enter your nose. Inside the nose, in the upper-rear part called the nose epithelium, there are cells that have special molecules called receptors. Each receptor has a unique shape, so that it “likes” certain odorants more than others and is more activated by their presence (Figure 2). These receptors are present on nerve cells called olfactory sensory neurons (OSNs). The interaction between odor molecules and OSNs is translated by the OSNs into electrical signals, which then travel to the brain. Due to this brilliant design, each odorant (such as beta-ionone when you smell a rose, limonene when you smell a lemon, or benzyl acetate when you smell strawberries), activates a unique combination of OSNs [1] out of more than one thousand OSN types that you have, and this in turn causes a unique pattern of electrical activity that enables your perception of a specific odor. To sum up, the coding of odors in the brain is based on the activation of a unique subset of OSNs. Each OSN is activated electrically when its receptors interact with specific odorant molecules, but not with other molecules. This electrical activity then travels into various regions in the brain that process and represent smell information.
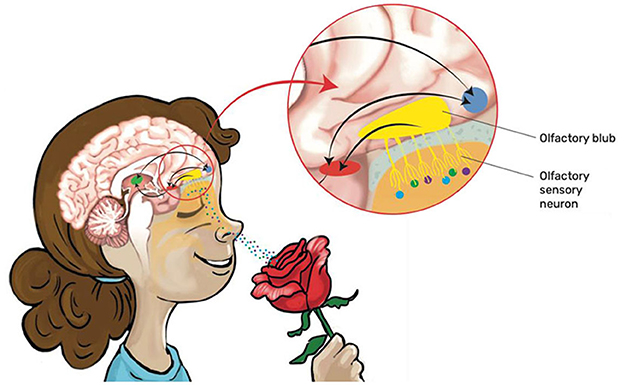
- Figure 2 - Smell receptors in the nose.
- At the inner, upper-rear part of the nose, called the nose epithelium, there are cells called olfactory sensory neurons (OSNs) that have protein molecules called odorant receptors. The receptors on OSNs interact with molecules that are floating around in the air, and the interaction of odorant molecules with OSNs generates an electrical signal that moves through nerve fibers (axons) to a brain area called the olfactory bulb. From there, the electrical activity continues on to additional regions in the brain (colorful circles) that process smell information.
Identifying Odor Receptor Genes
When I started studying the olfactory system, it was known that animals can tell apart extremely large numbers of different odors, but it was not clear how. What was clearly implied was that there must be a brain mechanism that enables an animal to recognize this vast diversity of odor molecules. This led to the idea that there must be a very large number of genes that code for the odor receptors. Also, the OSNs, which translate the odorant receptors, must have a way to turn the odorant-receptor interaction into electrical signals.
In our research, we wanted to find the genes that code for (or dictate the nature of) odorant receptors. To do that, we made three assumptions that simplified our search: (1) there should be a large family of genes coding for odorant receptors; (2) odorant receptors have a mechanism for turning interactions with odor molecules into electrical signals; and (3) genes coding for odorant receptors are expressed only in OSNs in the nose epithelium. Using these assumptions, we were able to efficiently search for a family of genes that code for odorant receptors in mice. We isolated their OSNs and found a new gene family in the mouse DNA that consists of about 1,000 different receptor genes [2]. This was very exciting, since it was the first time that odorant receptor genes had been identified. About 23 years later, in 2004, this discovery earned me and my colleague Prof. Linda Buck a Nobel Prize in Physiology or Medicine [3].
After identifying odorant receptor genes, we could then use sophisticated techniques (including molecular genetics and neural imaging) to ask more complex questions about the organization and activity of OSNs in the nose and brain. For example, how many of the receptor genes does every OSN express? Does each OSN contain only one type of odorant receptor or multiple types of receptors? These two possibilities imply two totally different structures and functional mechanisms of the olfactory system. As it turned out, the first possibility was correct: each OSN expresses only 1 out of about 1,000 possible receptor genes.
Organization of Olfactory Sensory Neurons
Now let us look at how OSNs are organized in the nose and in the brain, where odor recognition occurs. In mice, there are 10 million OSNs in the nose and 1,000 different odor receptors. Since each OSN expresses only one receptor type, this means that each receptor type is expressed in 10,000 neurons (10 million/1,000 = 10,000). But how are the 10,000 neurons expressing the same odorant receptor organized in the nose epithelium? Are they spread out over a large area, or are they clustered close together? And what happens to the electrical information that they generate when they interact with their favorite odorant? Does the information for a specific odorant converge (or come together) to a specific region of the brain?
We found the answer to these questions by using an advanced technique that selectively colors neurons expressing the same receptor gene. In the nose epithelium, the OSNs are randomly distributed over a large area (Figure 3). The OSNs then send their extensions (fibers called axons) into the first relay station in the brain that processes smell, called the olfactory bulb. All the 10,000 OSNs expressing a particular receptor converge at a specific region in the olfactory bulb, called a glomerulus (Figure 3, right). Overall, there are 1,000 separate glomeruli in the olfactory bulb and each receives information from all the (10,000) OSNs expressing a specific receptor gene [4].
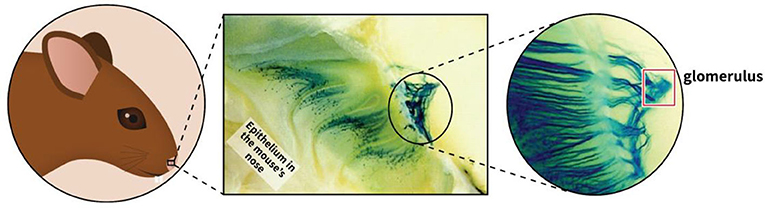
- Figure 3 - Organization of olfactory sensory neurons.
- In the mouse’s nose, there are one million OSNs. Each OSN expresses only one specific odorant receptor, so each type of odorant receptor is expressed by ~10,000 OSNs spread throughout the nose epithelium (small blue dots in central image). Each OSN sends its electrical information via an axon (blue fibers) to a single location in the olfactory bulb, called a glomerulus (red square on the right). The fibers of all 10,000 OSNs expressing the same receptor converge in the same glomerulus. Sensory cells expressing different receptors converge in different glomeruli. (Image adapted from [4]).
Activity of Olfactory Sensory Neurons
What happens when an animal is exposed to a specific odor (Box 1)? Odors are composed of many molecules (e.g., more than 400 in the case of a rose [6]). As we saw, each odorant molecule activates a specific group of OSNs (10,000 of them), which converge in and activate a specific glomerulus in the olfactory bulb. Smelling a rose activates a different group of glomeruli than smelling chocolate does, so specific smells create specific patterns (or “maps”) of glomeruli activity in the brain.
Box 1 - Olfactory systems of animals.
The ability to sense and differentiate between smells is very important to many animals, and olfaction was probably the earliest sense to evolve in organisms. There are many similarities between the olfactory systems of various animals. For example, the olfactory system of fruit flies, similar to that of humans and rodents, contains specific cells that each identify a relatively small number of odor molecules [5]. In fruit flies, the odor receptor cells (located on their two antennae) expressing the same receptor also converge in the same glomerulus. However, fruit flies have fewer glomeruli (about 60, compared to 1,000 in humans). The similarity between the olfactory systems of flies and humans allows scientists to draw important conclusions about the human olfactory system by studying fruit flies, which are easier to study than humans. There are also key differences between the olfaction systems of humans and flies, and smells that are considered pleasant or unpleasant for humans will not necessarily be experienced for the same way by flies, and vice versa.
Nowadays, we can look down on the olfactory bulb of rodents using neural imaging techniques, and “read” the spatially organized patterns (the “map”) of neural activity, and decipher from this activity which odor was encountered. This is a great new method for scientists to recognize odors, but it is clearly not the way by which the animal recognizes odors as it does not have an imaging microscope in its olfactory bulb and cannot look from the outside on its own neural activity as we scientists do.
Although the fascinating ability of the brain to recognize odors is not yet fully understood, we do know that the neurons in the olfactory bulb project their axons to multiple brain regions (Figure 4). Some of these regions are responsible for automatic behaviors in response to odors. For example, when an animal encounters a specific odor that suggests the presence of danger, such as when a mouse encounters the odor of a cat, an automatic “run away!” response is activated. Other axons travel from the olfactory bulb to brain regions where learning takes place. There, an animal learns specific behavioral responses based on the odors it encounters [3].
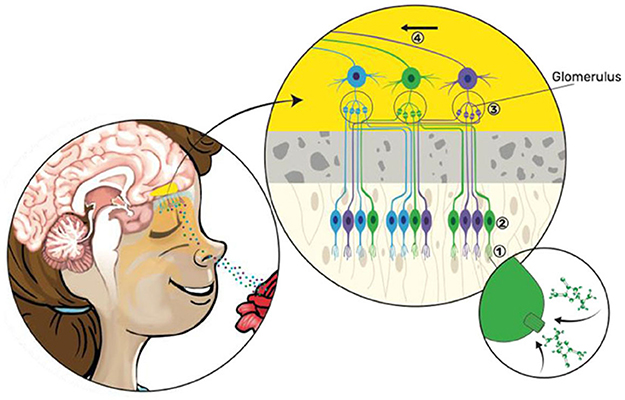
- Figure 4 - Anatomical and functional organization of the olfactory system.
- (Left) Odorant molecules enter the nose and bind to receptors on OSNs. Each ONS expresses only one type of olfactory receptor (differently colored “fingers”). OSNs’ axons cross the nose bone and enter the first relay station of the olfactory system in the brain—the olfactory bulb (yellow). (Right) Close-up of the area linked from the arrow on the left. (1) OSNs expressing the same receptor bind only to certain odor molecules (enlarged example is shown for the “green” OSN). (2) OSNs expressing the same receptor (indicated by color) are spread throughout the nose epithelium. (3) OSNs expressing the same receptor send their axons to the same glomerulus. (4) Information travels from the olfactory bulb to other regions of the brain, responsible for either automatic or learned behavior (Image adapted from: The Nobel Prize).
The vast majority of human responses to odors are of the second type, learned rather than automatic. People impose learned meanings upon specific odors, and those personal meanings affect their responses to the odors. For example, someone who has a romantic first date that includes wine could learn to associate the smell of that wine with the feeling of love. Afterwards, when they smell wine, they feel “excitement butterflies” and want to reach out to their loved one.
This means that individuals may have unique behavioral responses to a specific odor, depending on their past experiences with that odor (Box 2). For some of us, smelling a rose is associated with a beautiful emotional experience (like love), whereas for others a rose might be associated with the color red, which might be associated with blood and fear. But if our brains respond differently to the same odor molecule, then do we actually experience the same smell when we smell a particular odor?
Box 2 - Olfaction and age.
Olfaction tends to weaken as we get older: our ability to detect faint smells or to differentiate between smells decreases. Several factors lead to this, including decreasing numbers of odorant receptors and decreased function of certain brain regions. Interestingly, recent studies have shown that losing your sense of smell is a precursor to Alzheimer’s disease, and can help diagnose the disease more than 10 years before memory-related symptoms.
Does My Orange Smell Like Your Orange?
Imagine that someone who has never seen nor smelled an orange asks you to describe what an orange smells like. Could you put it into words? Probably not—an orange just smells like an orange, and you learn to know and recognize that smell by association. When you see an orange, you smell it at the same time; then, even if you smell it in the dark, you know that it is an orange by associating the smell with the image of an orange, or with the name “orange.” In this sense, olfaction is fundamentally different from vision. If someone has never seen an orange before and asks you what an orange looks like, you could say that it is round, has an orange color, is about the size of a baseball, has a smooth surface, etc. You can create an internal image of an object in your brain, but you cannot really create an internal image of an odor in your brain.
If we cannot describe odors using language, and cannot even build an internal image of odors, how do we know that you and I smell the same odor when we smell an orange? The answer is that we do not know! As we mentioned, it is very likely that, when you and I smell an orange for the first time, we are activating different patterns of neural activity in our individual brains—but both of us are associating that odor with the same fruit, because we are both seeing an orange. Apart from associating the odor with the object “orange,” are we actually having a similar experience of that odor? Maybe, but the odors we perceive could be relative to everything else we have smelled in our lives. For example, an orange smells more like a lemon than it smells like coffee, and that is true for all of us. This means that the similarities between the way individuals perceive odors might be purely relative—simply, that we all agree that a specific object smells more like some objects than others. This is a good reminder that our perception of odors is not absolute—unlike our perception of the color red, for example, which is based on the unchanging frequency of light.
The Riddle Of Perception—An Open Question For Future Scientists
There is one very important and complex question about perception that science has not yet answered. This question is valid for olfaction and all other senses and so is very fundamental—asking how interpretation of sensory information happens.
As we discussed, when the brain processes information from the senses, a specific pattern of electrical activity is generated in a particular set of neurons in the brain, representing the physical world in the brain through patterns of neural activity. These patterns of activity can vary in time (when they occur) and space (where in the brain they occur). So, the richness and variety of the whole physical world is somehow represented by the firing of specific groups of neurons at specific times and places in the brain.
For scientists, this implies two things: first, that physical reality is abstracted by the brain and, second, that the brain must interpret this abstract information to give it a meaning. For example, an object in the outside world, like an orange, is translated into a specific pattern of electrical activity that represents it in the brain, and then the brain “figures out” the meaning of this electrical activity (that there is an orange out there in the world) by interpreting and imposing meaning on this activity. But the brain must somehow associate this pattern of activity with a meaning like “this is the smell of an orange; it makes me feel good because it reminds me of the orchard I visited a few years ago…”. Currently, this surprising “jump” from electrical activity in the brain to the interpretation and meaning we place on it is a true mystery—we do not yet understand how it happens. I believe that this “magical” step is the next big riddle that future neuroscientists should address. Perhaps you will be one of them?
Recommendations For Young Minds
In my view, there is a very simple way to choose what to do in life. It does not matter what you choose—whether it is science or construction. But whatever it is, make sure to choose an area that you love, devote yourself to it, and work on it with intensity and passion. That is all! You must be passionate about what you are doing. This passion, fed by skill and knowledge, will drive you forward to excellence. So, you must discover your field of interest and then learn as much as you can about this field. When passion and knowledge are tied together, it often leads to creativity and happiness.
Additional Materials
Glossary
Olfactory System: ↑ The sensory system responsible for smelling.
Odorant: ↑ Odor molecules that are released from objects, travel through the air, and enter your nose.
Nose Epithelium: ↑ A tissue in the upper rear part of the nose involved in smelling.
Receptor: ↑ A molecule on a cell that interacts very specifically with another molecule, like a lock and a key, and translates the interaction into a signal within the cell.
Olfactory Sensory Neurons (OSNs): ↑ Nerve cells containing odor receptors that translate interaction with odorants into electrical signals which travel to the brain.
Neuron: ↑ A nerve cell; the main cell type in the brain. Neurons generate electrical signals and transmit them to other nerve cells.
Axons: ↑ Nerve fibers that carry electrical signals from one neuron to another.
Olfactory Bulb: ↑ The first relay station in the brain involved in smelling. It receives information from OSNs and transmits information about odors to other areas of the brain.
Glomerulus: ↑ A region in the olfactory bulb at which all OSNs expressing a particular receptor converge.
Conflict of Interest
The author declares that the research was conducted in the absence of any commercial or financial relationships that could be construed as a potential conflict of interest.
Acknowledgments
I wish to thank Noa Segev for conducting the interview which served as the basis for this paper and for co-authoring the paper, Sharon Amlani for providing the illustrations, and to Susan Debad for copyediting the manuscript. Special thanks to Haran Shani-Narkiss for his valuable comments on the manuscript.
References
[1] ↑ Duchamp-Viret, P., Duchamp, A., and Chaput, M. A. 2003. Single olfactory sensory neurons simultaneously integrate the components of an odour mixture. Euro. J. Neurosci. 18:2690–6. doi: 10.1111/j.1460-9568.2003.03001.x
[2] ↑ Buck, L., and Axel, R. 1991. A novel multigene family may encode odorant receptors: a molecular basis for odor recognition. Cell. 65, 175–87. doi: 10.1016/0092-8674(91)90418-X
[3] ↑ Axel, R. 2005. Scents and sensibility: A molecular logic of olfactory perception (Nobel lecture). Angewandte Chemie Int. Edn. 44:6110–27. doi: 10.1002/anie.200501726
[4] ↑ Mombaerts, P., Wang, F., Dulac, C., Chao, S. K., Nemes, A., Mendelsohn, M., et al. 1996. Visualizing an olfactory sensory map. Cell. 87:675–86. doi: 10.1016/S0092-8674(00)81387-2
[5] ↑ Wilson, R. I. 2013. Early olfactory processing in Drosophila: mechanisms and principles. Ann. Rev. Neurosci. 36:217. doi: 10.1146/annurev-neuro-062111-150533
[6] ↑ Shalit, M., Guterman, I., Volpin, H., Bar, E., Tamari, T., Menda, N., et al. 2003. Volatile ester formation in roses. Identification of an acetyl-coenzyme A. Geraniol/citronellol acetyltransferase in developing rose petals. Plant Physiol. 131:1868–76. doi: 10.1104/pp.102.018572