Abstract
Bacteria and viruses may enter our bodies through mucous membranes of the airways or the gut. To prevent infections, one defense mechanism of our immune system is antimicrobial peptides (AMPs). Most AMPs are composed of 10–50 amino acids and insert into bacterial cell membranes to destroy the cell. Some AMPs are also active against viruses and fungi. AMPs can be generated by chopping up bigger proteins like hemoglobin. The hemoglobin fragments can inactivate bacteria and viruses, while the whole hemoglobin protein cannot. To identify new AMPs, peptide libraries consisting of thousands of different peptides can be generated from human body fluids and organs. These libraries are tested for antibacterial or antiviral activity and can be further purified to identify the responsible peptide. This method may lead to the development of new antimicrobial substances with a potential for treating infections.
How Do Bacteria and Viruses Get Into Our Bodies?
We are constantly in contact with bacteria and viruses that are present in the surrounding environment or other people. Some of these bacteria and viruses may cause human infections and are called microbial pathogens. Two important ways for microbial pathogens to get into our bodies are through the respiratory and gastrointestinal tracts. The oral cavity, the nose, and the throat belong to the upper respiratory tract, while the bronchi and the lungs are part of the lower respiratory tract. Through the constant exposure of the respiratory tract to the air that we breathe, which may contain little droplets of fluid harboring bacteria or viruses, microbial pathogens can get into the lungs and cause a severe infection called pneumonia. The mouth, esophagus, stomach, and intestines are part of the gastrointestinal tract. Food and fluids continuously transport microorganisms into the gastrointestinal tract. Most bacteria and viruses that we take up through food are killed or inactivated in the very acidic gastric fluid of the stomach. However, some may reach the gut and can cause gastrointestinal infections and diarrhea. While the respiratory and gastrointestinal tracts are the most common entry sites for microbial pathogens, there are also other ways to infect humans, including transmission through blood, open wounds, sexual contact, and insect bites, to name just some.
How Does the Body Defend Itself?
To successfully fight off bacteria and viruses, the human body has developed multiple ways to prevent infections. These include physical barriers like the skin, which limits access of microorganisms to deeper tissues, as well as the mucus and fluids on our mucous membranes, which wash away bacteria and viruses and also contain molecules that kill and inactivate them. A more specific defense against unwanted microbial pathogens is called the immune system, which consists of two branches. The innate immune system is ready to attack microbial pathogens the very first time we see them, by recognizing specific microbial patterns. To be better prepared for a second attack, the adaptive immune system learns from the first contact with a microbial pathogen and can remember microbes it has seen before, responding more quickly and powerfully if the pathogen returns.
In this article, we will concentrate on one important part of the innate immune system, called antimicrobial peptides (AMPs), which can be found in many different organs and tissues or on the surfaces of mucous membranes or the skin. On human skin alone, more than 20 different AMPs have been found.
What Are Antimicrobial Peptides?
AMPs are an ancient defense mechanism that can be found in almost all living organisms [1]. Most AMPs are rather small, consisting of about 20–50 amino acids, which are the building blocks of peptides and proteins, which are larger than peptides. AMPs can be generated by skin cells and cells of the immune system that are present on our mucous membranes or skin. Some cells of the innate immune system make their own AMPs, but AMPs can also be generated by chopping up other large proteins that normally perform entirely different functions in the body. The small fragments of larger proteins gain the ability to attack microbial pathogens, so these larger proteins, called AMP precursors, serve two functions.
Examples of Human AMP Precursors
To ensure that enough AMPs are generated when the precursor is chopped up, the precursor protein itself should be present at high concentrations. Indeed, all proteins known to release AMPs are highly abundant and can be found almost everywhere in the human body. One example is hemoglobin, the iron-containing red pigment in red blood cells, which gives rise to many AMPs with antibacterial and antiviral activity [2]. Another important example is fibrinogen. Fibrinogen is involved in blood clotting and wound healing, which means that fibrinogen might speed up the wound-healing process by both helping with clotting and producing AMPs to help keep the wound uninfected. Thrombin is another important source of AMPs. Thrombin plays an important role in the blood coagulation pathway. Other examples of AMP precursors include prostatic acid phosphatase and semenogelins, which are highly abundant in human semen. When these semen proteins are cut up into AMPs, they form fibers that can capture bacteria in the female reproductive tract, helping the cells of the immune system to more effectively destroy bacteria [3].
How Are the Protein Fragments Generated?
The large AMP precursors are chopped up into AMPs by special cutting proteins called proteases. Proteases are enzymes that cut proteins into smaller fragments. Protein fragments are called peptides if they are less than ~100 amino acids long. Proteases are mainly found in the gut, for the digestion of proteins in food. Proteases cannot distinguish between the proteins of the human body and food proteins that need to be chopped up. Therefore, protease activity is tightly regulated to prevent uncontrolled digestion of our own proteins. However, several proteases also cleave the hemoglobin that gets into our tissues when injury or bleeding occur. The AMPs produced from chopped-up hemoglobin have antibacterial and antiviral activity, which the complete hemoglobin protein does not have.
How Do AMPs Stop Bacteria?
AMPs often have a positive charge, and bacterial membranes often have a negative charge. Positive and negative charges attract each other, making it easy for AMPs to bind to the bacterial surface. Furthermore, AMPs are often amphipathic, meaning part of the peptide molecule is attracted to water, while another part is more attracted to lipids (fats). The amphipathic nature of AMPs helps them to bind to the bacterial membrane, because the membrane consists of both lipids and some water-like parts.
After attaching, the AMPs insert into the bacterial membrane and may form a small tunnel, called a pore. This process resembles punching a hole into the bacterial cell, which leads to leakage of the cell’s contents and death of the bacterium. The whole process can be seen using an electron microscope (Figure 1). There are also AMPs that do not attack the bacterial cell membrane directly, but instead disturb the function of important molecules inside the bacterial cell, such as the molecules that help build the bacterial cell wall. Some AMPs can bind directly to bacterial DNA, destroying the DNA’s ability to pass on genetic information for the building of new proteins or bacterial cells.
![Figure 1 - Electron microscopy pictures of bacteria (Pseudomonas aeruginosa) with and without treatment with an AMP originating from hemoglobin [2].](https://www.frontiersin.org/files/Articles/567875/frym-09-567875-HTML/image_m/figure-1.jpg)
- Figure 1 - Electron microscopy pictures of bacteria (Pseudomonas aeruginosa) with and without treatment with an AMP originating from hemoglobin [2].
- (A,B) Bacterial cells that were not treated with the AMP are healthy and whole. (C–E) Bacteria that were treated with the AMP from hemoglobin for 1 h. Black arrows indicate destroyed bacterial cell membranes and the spilling of the content from inside the bacterial cells.
How Do AMPs Inhibit Viruses?
Viruses are different from bacteria in that they can only multiply within cells—so viruses must get into human cells before they can replicate and make us sick. Some AMPs can interfere with the various steps of virus multiplication. AMPs called magainins, from frog skin, interact with the outer surface of the virus and disrupt the virus’s structure. A human peptide called VIRIP, isolated from blood, is an AMP that can inhibit the entry of human immunodeficiency virus into human cells. Some AMPs can block the attachment of viruses to cells. One example is a blood-derived peptide that inhibits the initial binding of a virus called cytomegalovirus to human cells. Human AMPs called defensins and cathelicidins are also known to help prevent viral entry into cells, but they also interfere with later steps of virus multiplication.
Finding New AMPs
While many different human AMPs have been identified in recent years, there are certainly many that we have not yet found. Microbial pathogens are becoming more and more resistant to common antibiotics and antiviral drugs, making these drugs useless to fight infections. It is therefore important to find additional AMPs with antibacterial or antiviral activity, so that these may eventually be developed into new drugs. To detect new human AMPs, it is possible to generate large peptide libraries from various organs and body fluids, containing hundreds of thousands of different peptides. With the consent of the donor, tissues removed during surgeries and body fluids collected during medical procedures are used to generate peptide libraries for research purposes.
To make a library from tissues, the tissues must first be liquified to make an extract. Body fluids or tissue extracts are immediately cooled, acidified, and then frozen to prevent the peptides within them from breaking down. A special filter is used to concentrate the peptides, and larger proteins are removed. The peptides are then separated using a method called chromatography. Following chromatography, many thousands of different peptides are available as a peptide library, which can then be tested for peptides that inhibit bacterial growth or virus infection [4, 5] (Figure 2). A fraction of the peptide library that shows antibacterial or antiviral activity can be further purified, retested, and eventually the identity of the AMP can be determined. Finally, the AMP can be recreated in the lab and analyzed for its level of activity, its role in preventing infections, and its ability to be used as a medical treatment.
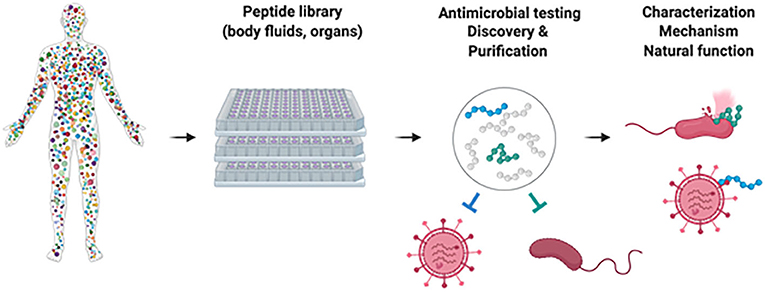
- Figure 2 - How to find new antimicrobial peptides: human organs or body fluids are homogenized and small molecules extracted.
- Peptides are separated by chromatography, yielding hundreds of fractions. Antiviral and antibacterial activity of these fractions is evaluated and those with antimicrobial activity are further separated. Eventually, the antimicrobial peptide is discovered and its mechanism of action can be determined. Figure created with biorender.com.
Conclusion
Multiple antimicrobial peptides are present in our bodies that help prevent infections. They are part of our innate immunity and can be generated from larger human proteins that have other purposes. To find new AMPs large peptide libraries can be searched and AMPs may eventually be developed into antibacterial or antiviral treatments.
Glossary
Microbial Pathogens: ↑ Germs, infectious microorganisms, or agents, such as viruses, bacteria, parasites, and fungi that can cause infections.
Innate Immune System: ↑ Part of the immune system that attacks microbial pathogens upon first contact.
Adaptive Immune System: ↑ Part of the immune system that has been acquired through first contact with microbial pathogens, mounting a specific and efficient response to eliminate microbial pathogens.
Antimicrobial Peptides: ↑ Small peptides that can destroy bacteria and viruses.
AMP Precursors: ↑ Larger protein that is cut down to a fragment with antimicrobial activity.
Protease: ↑ Enzyme that cuts proteins.
Amphipathic: ↑ Characteristic of a molecule that has a part being attracted to water and a part being repulsed by water and attracted to lipids (fats).
Chromatography: ↑ Method to separate a mixture of different substances.
Conflict of Interest
The authors declare that the research was conducted in the absence of any commercial or financial relationships that could be construed as a potential conflict of interest.
References
[1] ↑ Bastos, P., Trindade, F., da Costa, J., Ferreira, R., and Vitorino, R. 2018. Human antimicrobial peptides in bodily fluids: current knowledge and therapeutic perspectives in the postantibiotic era. Med Res Rev. 38:101–46. doi: 10.1002/med.21435
[2] ↑ Gross, R., Bauer, R., Kruger, F., Rucker-Braun, E., Olari, L. R., Standker, L., et al. 2020. A placenta derived C-terminal fragment of beta-hemoglobin with combined antibacterial and antiviral activity. Front. Microbiol. 11:508. doi: 10.3389/fmicb.2020.00508
[3] ↑ Rocker, A., Roan, N. R., Yadav, J. K., Fandrich, M., and Münch, J. 2018. Structure, function and antagonism of semen amyloids. Chem. Commun. 54:7557–69. doi: 10.1039/c8cc01491d
[4] ↑ Bosso, M., Standker, L., Kirchhoff, F., and Munch, J. 2018. Exploiting the human peptidome for novel antimicrobial and anticancer agents. Bioorg. Med. Chem. 26:2719–26. doi: 10.1016/j.bmc.2017.10.038
[5] ↑ Munch, J., Standker, L., Forssmann, W. G., and Kirchhoff, F. 2014. Discovery of modulators of HIV-1 infection from the human peptidome. Nat. Rev. Microbiol. 12:715–22. doi: 10.1038/nrmicro3312