Abstract
Climate change can occur when chemicals called greenhouse gases enter the Earth’s atmosphere. Carbon dioxide is one important greenhouse gas. A new technology to reduce the amount of greenhouse gases from entering Earth’s atmosphere involves putting large amounts of carbon dioxide underground. Microbes, such as bacteria, are found almost everywhere on Earth, including in places many kilometers underground. Scientists combine skills from microbiology (the study of microbes), geology, and chemistry to understand how underground bacteria can help reduce greenhouse gases in the atmosphere and keep our planet healthy.
There Are Bacteria Living Underground That Are Being Exposed to Gases From the Atmosphere
People need petrol (gasoline) or electricity to drive cars, watch television, or play games on their iPads. Chemicals called greenhouse gases are produced when we make petrol and electricity for people to use. Greenhouse gases can be harmful when they enter the Earth’s atmosphere in large amounts, and they can cause problems for the environment. A large increase in greenhouse gases over a short period of time can cause changes in the world’s weather patterns. These changes can cause the polar ice caps to melt and sea water levels to rise, which can flood cities close to the sea and leave people without homes.
Carbon dioxide (CO2) is an important greenhouse gas. Scientists have noticed increases in CO2 in the atmosphere, due to human activity. One way that scientists can help reduce greenhouse gases in the atmosphere is known as “carbon capture and storage.” Scientists can use special equipment to “catch” carbon dioxide before it enters the atmosphere, and then store the harmful gas deep underground.
There are places underground that can store large volumes of CO2 gas. These places are the nearly infinite number of tiny holes inside of rocks (Figure 1). The underground rocks form layers that go on for many kilometers in all directions and can be many meters thick. These layers are called aquifers. Aquifers contain water that can move around freely through the rocks, through the tiny holes. When the CO2 gas is injected into one of these aquifers, it can be stabilized so that it does not “leak” back into the atmosphere. The CO2 is stabilized when it becomes trapped inside the tiny holes of the rocks. Big outdoor experiments have shown that these aquifers can remain stable over long periods of time.
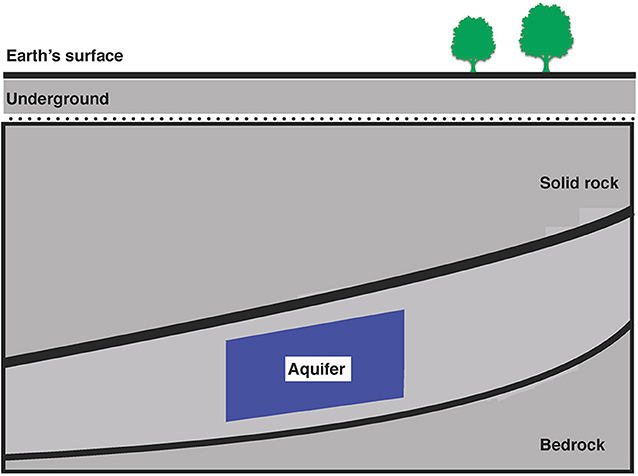
- Figure 1 - Aquifers are located deep under Earth’s surface.
- An aquifer is an underground layer of rock that contains water. The aquifer used in this study was 1.4 km under Earth’s surface. Water samples collected from this aquifer were used in experiments to study whether injection of CO2 into the aquifer changed the populations of bacteria that live there.
However, very little is known about how the CO2 affects the microbes that live underground. Microbes are microscopic organisms, including bacteria, which are the type of microbe we investigated in our experiments. It is important to understand how bacteria respond to underground CO2, because, through their metabolism, the bacteria can change the CO2 into a more harmful greenhouse gas called methane (Figure 2). Scientists do not want these underground bacteria to produce methane, because the methane can escape up into the atmosphere and make climate change worse. Therefore, the aim of our study was to understand how bacteria that live underground respond to CO2 gas being pumped into their “home” in a deep aquifer.
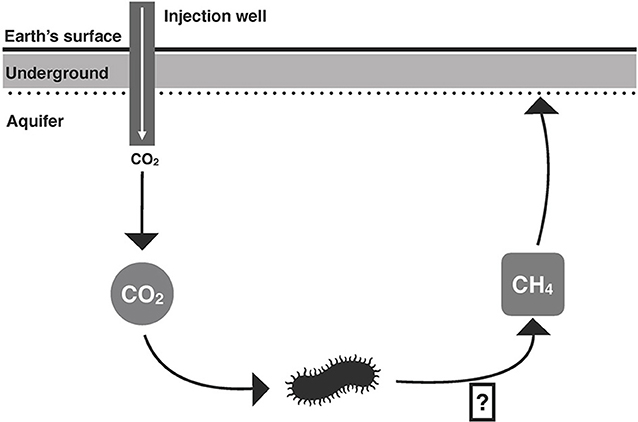
- Figure 2 - How do bacteria react to CO2? One of the key questions we asked in our study was: “How will the bacteria in the aquifer respond to injection of CO2?” While injection of CO2 into the aquifer may remove this greenhouse gas from the atmosphere, it is also possible that the bacteria living deep in the aquifer could convert the CO2 into a more harmful greenhouse gas, methane (CH4), that might then be released into the atmosphere.
DNA From Bacteria Helps Scientists Understand How They Respond to CO2 Many Kilometers Under the Ground
Drs. John Moreau and Andre Mu are researchers at the University of Melbourne in Australia. John is trained in environmental chemistry, while Andre is a microbiologist who studies microbes, including bacteria. Together, they are experts at understanding how bacteria respond to large amounts of CO2 below Earth’s surface. There are two main ways to determine how underground bacteria respond to CO2. We can (1) measure the changes in water chemistry in the aquifers; and (2) look at the different types of bacteria that are present before and after injecting CO2 gas underground (Figure 3). It is often difficult to grow these underground bacteria in the laboratory, because we do not fully understand what conditions they require to grow. Instead, we look at the DNA from these bacteria, which can be collected without the need to grow them in the lab. DNA is a basic building block of life on Earth. Scientists use a combination of chemicals, very small glass beads, and a machine to carefully break the bacterial cells apart. The DNA is then collected from the cells and prepared for sequencing, which is a technique used to identify the order of the four different chemicals (abbreviated G, A, T, and C) that make up DNA (Figure 3). Understanding the DNA from our samples will give us an idea of the types of bacteria that live underground. The bacteria each have a unique DNA sequence that can be used to identify them, like a “fingerprint.” The bacteria we identify can then be classified into groups. For example, some bacteria are autotrophs, which produce complex organic compounds from simple substances, and others are heterotrophs, which use the complex compounds produced by autotrophs for food/energy. It is important to understand how these two groups of bacteria interact with each other during the storage of CO2 gas in underground aquifers.
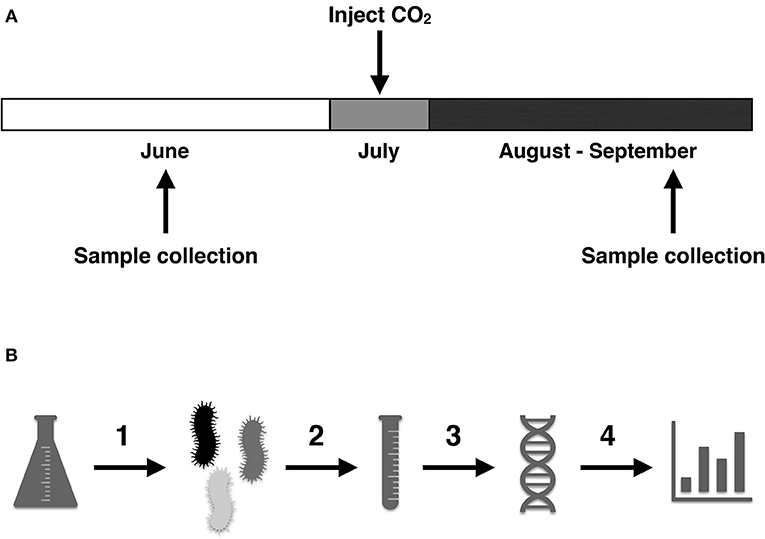
- Figure 3 - What did we do and when did we do it? (A) We collected water samples from the aquifer before injection of CO2, during the month of June, to understand the “baseline” conditions of the aquifer.
- Large amounts of CO2 were injected during the month of July and were allowed to settle underground. Samples were then collected for testing during August and September, to understand what CO2 did to both the water of the aquifer and the bacteria that live there. (B) DNA was extracted from the bacterial samples by: (1) filtering the water samples to collect the bacteria; (2) using a mixture of chemicals and glass beads to extract the DNA; (3) sequencing the DNA; and (4) using computers to help scientists understand which bacteria were present before and after CO2 injection.
John and Andre teamed up with a group of scientists who are trained in geology and engineering, to perform a large experiment in the Otway Basin in Southeastern Australia. The experiment involved pumping 150 tons of CO2 gas more than one kilometer underground into an aquifer. That is almost the same distance as 10 football fields (Australian-rules football), and the weight of more than 2,000 football players! Water from the aquifer was collected before and after injecting the CO2 gas. These water samples were tested for different chemicals and conditions, such as pH and temperature. We also used high-powered computers to study the DNA of the bacteria present during the different stages of the experiment. We wanted to know if we would see the same groups of bacteria, or whether new groups would appear after the CO2 was injected deep into the aquifer.
Some Bacteria Respond Well to the Injected CO2, While Others Are Not Too Happy
Two types of bacteria were present in the water samples in very low amounts before injecting the CO2 gas. These bacteria are called Comamonadaceae and Sphingomonadaceae. These two types of bacteria stayed at low numbers until large volumes of CO2 gas were pumped into the ground. Changes in the water chemistry occurred after injecting all the CO2 gas, and 41 days after CO2 injection, the numbers of Comamonadaceae and Sphingomonadaceae both increased. These two types of bacteria have the ability to produce gel-like communities, called biofilms, that act as a barrier to protect the bacterial cells from a changing environment. Increasing proportions of Comamonadaceae and Sphingomonadaceae after the injection of CO2 gas suggests that these types of bacteria can adapt to changes in water chemistry. Scientists believe that biofilms produced by bacteria can block holes and weak points in the aquifer, preventing CO2 gas from leaking back into the atmosphere, which is really important in order for this technique to be effective in reducing the amount of CO2 contributing to climate change.
We also found a type of bacteria called Carboxydocella in the aquifer water samples. Carboxydocella grows well in the conditions of the aquifer, where there is almost no oxygen, the pressure of the overlying rocks is 137 times more than what we feel on the surface of Earth, and temperatures are as high as 60°C (140°F). Carboxydocella is a type of autotroph. These bacteria can convert carbon monoxide (CO) from the environment to CO2, and they also produce hydrogen (H2) molecules. The CO2 and H2 are then used by other, heterotrophic bacteria as energy sources. Looking at the DNA we collected from our samples, we discovered that the percentage of Carboxydocella decreased and remained at low levels after the CO2 gas was injected into the Otway Basin. The decrease in Carboxydocella can change the bacterial community in ways that disrupt the storage of CO2 gas and may have negative impacts on the aquifer. This is an area of research that needs to be explored further by designing specific experiments in the laboratory. These experiments might include growing Carboxydocella under different concentrations of CO2 gas in the lab to determine what happens to this bacterium—can they slowly adapt to high CO2 concentrations, or will they disappear? What is clear, however, is that Carboxydocella plays an important role in cycling carbon, and supporting the growth of other bacteria living in the aquifer.
Bacteria Work Together As a Team to Support the Community
Different types of bacteria in the environment tend to work together as a team. Bacteria support each other by making compounds that they share in order to stay alive. From our data, we hypothesize that when the groundwater becomes more acidic as a result of the CO2 mixing with water, the acidic environment promotes the growth of a group of bacteria that can use a chemical compound, called sulfide, as a source of energy. When this reaction combines with other chemicals in the aquifer, the conditions underground change, and promote the growth of a different group of bacteria, called sulfate-reducers. Sulfate-reducers need H2 to make energy. Autotrophs, such as Carboxydocella, are a source of H2 for sulfate-reducers. Carboxydocella can also supply a group of bacteria known as methanogens with the CO2 and H2 they require to make methane. The injection of CO2 into aquifers may change the community of bacteria that live there. For example, Carboxydocella might disappear. If Carboxydocella disappears, then the ability of methanogens and sulfate-reducing bacteria to function might be lost as well. This might mean a change in how the CO2 is being stored, long term, in the aquifer.
What Is Next?
By doing big experiments in the environment, we can understand what happens in the real world. We can then use this knowledge to create simple experiments in the laboratory to understand better what happens to CO2 gas, and how bacteria respond to CO2 that is injected underground. The next steps in this research are to perform experiments in the laboratory, in which we grow a certain group of bacteria found in many different aquifers, and expose them to high pressure, high temperature, and lots of CO2 gas. Through these experiments, we will learn how biofilms from Comamonadaceae and Sphingomonadaceae react to CO2. When we have a better understanding of how CO2 gas interacts with these bacteria, we can then make faster progress toward reversing the damaging effects of climate change.
Glossary
Greenhouse Gasesk: ↑ Greenhouse gases are a type of gas that contributes to climate change. Carbon dioxide is an example of a greenhouse gas.
Carbon Capture and Storage: ↑ Carbon capture and storage is a technique being investigated by scientists as a way to reduce the impacts of climate change. The technique involves “catching” greenhouse gases before it enters Earth’s atmosphere, and storing the gas deep underground.
Aquifer: ↑ An aquifer is an underground layer of rock that contains water.
Bacteria: ↑ Bacteria are microscopic organisms, and consist of just a single cell with simple structures.
DNA: ↑ Deoxyribonucleic acid is a molecule carrying the genetic instructions for life on Earth.
Conflict of Interest Statement
The authors declare that the research was conducted in the absence of any commercial or financial relationships that could be construed as a potential conflict of interest.
Acknowledgments
The authors acknowledge the support of the CO2CRC, and funding from the Commonwealth of Australia through the CO2CRC program for the original experiments.
Original Source Article
↑ Mu, A., Boreham, C., Leong, H. X., Haese, R. R., and Moreau, J. M. Changes in the deep subsurface microbial biosphere resulting from a field-scale CO2 geosequestration experiment. Front. Microbiol. (2014) 5:209. doi: 10.3389/fmicb.2014.00209