Abstract
Volcanic eruptions demonstrate that our planet is alive and evolving. Take photos of volcanoes around the Earth and you will see that they all look different, but also share important features. Some volcanoes come in perfect conical shapes, while others are much flatter and harder to spot. This is just like humans, some are tall and others are short, or some have dark hair while others have light hair. This is important because the shape of a volcano can tell scientists about what sort of eruption can occur—the volcano’s personality! Humans are different from volcanoes—they can look very similar but have very different personalities. Here, we explain how the different personalities of volcanoes can be defined, and how this can help scientists and people around the world to predict the future impacts of volcanic eruptions.
The Shapes of Volcanoes
Scientists who study volcanoes are called volcanologists. Volcanologists like us travel the world to study as many volcanoes as possible. The more volcanoes we study, the better we understand how they work. Sometimes we arrive in a city like Naples in Italy and see right away from the train that a beautiful volcano dominates the city (Vesuvius; Figure 1). When we explore the area around Vesuvius, we find rocks that give us lots of information about the history of the volcano. To obtain this information, we determine the mineral content of our rock samples and analyze their chemical composition. This allows us to read rocks like books! This is what we do as volcanologists.
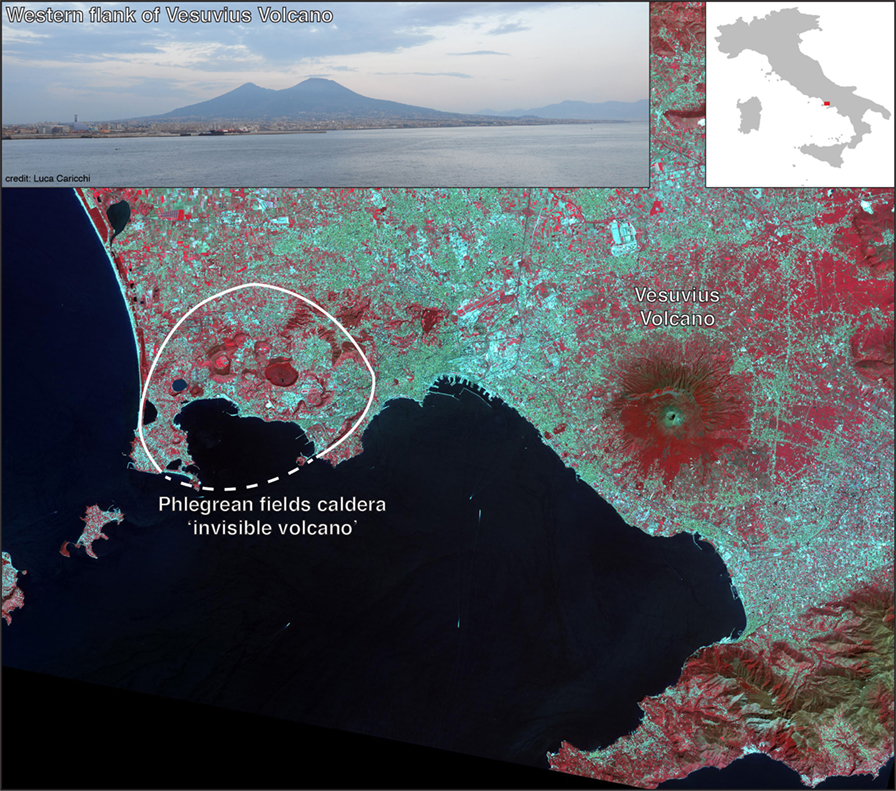
- Figure 1 - Satellite image of the area of Naples (Italy).
- The Vesuvius stratovolcano and the Phlegrean Fields caldera are labeled in the image. The drawing of Italy in the upper right corner shows the area pictured in the satellite image (in red). Credit: NASA (ASTER image).
What is interesting in Naples is that when you travel around the city, you discover other volcanic rocks. These rocks have different chemical compositions and look different from the rocks found next to Vesuvius. These rocks belong to another volcano nearby, called the Phlegrean Fields (Figure 1). This volcano is very hard to see from the ground, but it is so large that can be seen from space (Figure 1).
Pointy volcanoes like Vesuvius are called stratovolcanoes and flatter volcanoes like the Phlegrean Fields are called calderas. Stratovolcanoes are like the volcanoes you draw at school and they are some of the easiest volcanoes to spot. They look like mountains and have a central hole, called a crater, which is connected to an underground chamber. The shape of calderas is more similar to that of a large soup dish (Figure 2). Some of the first volcanologists called them the “inverted volcanoes” [1].
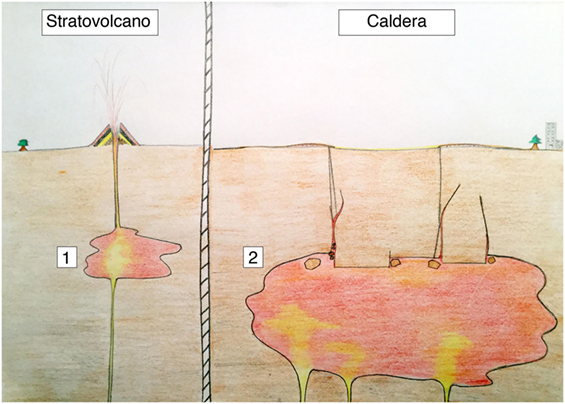
- Figure 2 - Drawing of a stratovolcano (left-hand side) and a caldera volcanic system (right-hand side).
- The magma chamber of a stratovolcano (1) is much smaller than that of a caldera (2)
Why such different shapes? When a volcano is not erupting, the rocks that will eventually be erupted are stored in a magma chamber, located 5–15 km below the volcano (Figure 2). The rocks in the magma chamber are so hot (about 800–1,000°C; your pizza cooks at 300°C) that they are molten. Molten rock is called magma. When a stratovolcano erupts, only a small part of the magma in the chamber erupts and through eruption after eruption, the cooled magma builds up to form a cone. Calderas are different. When they erupt, so much magma comes out of the magma chamber that the surface sinks to form the “bowl,” which we call the caldera. So, an important question for scientists is, “why do volcanoes look different?”
The Size of the Magma Chamber Can Determine a Volcano’s Personality
The magma contained in the chamber below a volcano is molten, which makes it less dense than the rock around the chamber. This means that the magma in the chamber is buoyant (it wants to rise to the surface). Buoyancy is the force you feel when you try to immerse a football into water. You can feel buoyancy trying to make the ball come up to the surface. Archimedes of Syracuse described this force for the first time 2,200 years ago, and it was a very important discovery, because it explains why boats float on water. Unlike a football under water, which will always want to rise to the surface, the magma is kept below a volcano because the rock surrounding the magma chamber is strong enough to stop it from rising. So how does magma erupt?
The rock surrounding the magma chamber is like you trying to hold the football under water. If the football is small you succeed, but the larger the football, the more difficult it is for you to hold it below water. The magma chambers below caldera volcanoes grow so large that eventually an eruption occurs. Other volcanologists also showed that, in some cases, the heat the magma contains consumes the rocks on top of the magma chamber, which like you holding the football below water with less force, eventually let the magma rise and an eruption occurs [2]. In other cases an earthquake can shake the magma chamber, which like shaking a bottle of soda, can lead to an eruption [3]. This is what we know for large magma chambers, but why do stratovolcanoes, with much smaller magma chambers, erupt?
In smaller chambers, the eruption trigger is different. For these volcanoes, the injection of new hotter magma from deep inside the Earth (60–100 km depth) can increase the pressure in the magma chamber enough to break the surrounding rock [4].
On Earth, large eruptions are much less frequent than small eruptions [5]. This means that most magma chambers cannot grow large enough to produce a caldera volcano, and so they lose magma in smaller eruptions. By studying rocks erupted by volcanoes, we can see that the sizes of eruptions from calderas are bigger than those from stratovolcanoes [6]. For example, while rocks related to Vesuvius can be found around Venice, Italy (540 km away), rocks related to Phlegrean Fields can be found as far away as Russia (4,000 km away!). By understanding why calderas and stratovolcanoes produce different sizes of eruptions (the volcano personality), and how the size of the eruption is related to the size of the magma chamber, scientists can help forecast what the impact of an eruption could be.
Which Volcano Personalities are More Risky?
Volcanologists study the personality of volcanoes because this information can determine how far from the volcanoes the eruptions will have an impact—remember rocks from the Phlegrean fields were found 4,000 km away! Now, we will tell you about the different hazards associated with volcanic eruptions.
When a volcano erupts explosively, the magma cools and is turned into volcanic ash and gases, like carbon dioxide (CO2) and sulfur dioxide (SO2). The ash travels so fast that it is injected far into the atmosphere, as high as planes can travel. Volcanic ash can stop planes from flying, because ash is bad for their engines. Volcanic ash and gas from large eruptions can travel in the atmosphere all around the Earth, changing the climate. Volcanic gases form aerosols (mixture of fine particles) in the atmosphere that reflect the solar radiation back to space and lead to global cooling. In fact, one eruption in Indonesia in 1815 (Tambora; Figure 3) caused a year without a summer in Europe and North America in 1816.
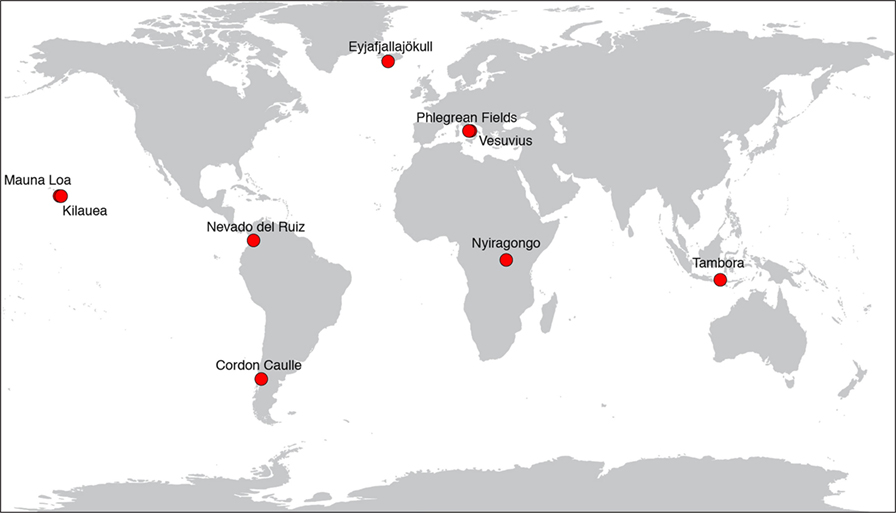
- Figure 3
- Map showing the location of the volcanoes discussed in the article.
Closer to the volcanoes, the ash can destroy life, by covering plants and polluting water sources. This happened in the 2010 Eyjafjallajökull eruption, in Iceland and the 2011 Cordon Caulle eruption, in Chile (Figure 3). Ash can travel down the slopes of a volcano in huge clouds, called pyroclastic flows, for tens of kilometers. Pyroclastic flows are very hot and fast mixtures of volcanic gas, ash, and blocks (volcanic rock >64 mm diameter) that travel down the volcano, destroying everything in their paths. They can travel up to 400 km/h! A pyroclastic flow occurred during the 79 AD eruption of Vesuvius, which completely destroyed the towns of Pompeii and Herculaneum. When ash mixes with water and flows down the flanks of the volcano it forms a lahar (an Indonesian word). Lahars are cooler than pyroclastic flows but can travel very fast and are also dangerous for communities around a volcano. One of the most infamous lahars in history killed more than 26,000 people in Colombia, as a result of the 1985 eruption of Nevado del Ruiz (Figure 3). If an eruption is not explosive it is effusive, meaning that magma pours out of the volcano, forming lava flows. Lava flows are hot, much slower than pyroclastic flows, and can burn down anything in their path. Lava flows occurred during the eruptions of Mauna Loa and Kilauea in Hawaii and the Nyiragongo volcano in the Democratic Republic of Congo (Figure 3).
Scientists can forecast where these volcanic hazards will have an impact using computer models to produce what are called hazard maps. On the maps you have in your classroom, colors indicate the arrangement of the land (green is for flat areas and brown and white for mountains, for example). On hazard maps, colors tell where different type of hazards will impact when an eruption occurs. Larger eruptions, like those produced from caldera volcanoes such as the Phlegrean Fields, will require a larger hazard map and so you might think that these volcanoes have more risky personalities. However, because it is much harder to produce a very large magma chamber, these eruptions are very rare. Smaller eruptions, like those from Vesuvius, will have a smaller hazard map but can occur more frequently. Because there are more eruptions at Vesuvius, this volcano could be more risky than the Phlegrean Fields!
We Should Respect the Personality of a Volcano
Today, more than 500 million people live next to volcanoes. In fact, volcanoes may seem scary, but the rocks they erupt are rich in elements that make the soil around them very fertile. Volcanoes are also important sources of renewable energy. The heat the magma contains can be used to produce electricity and the elements volcanoes give us can be used to build solar panels. This means that volcanoes are important for life on Earth, but they can also be very dangerous. To live near volcanoes, we need to understand their language and the messages they are sending us. Volcanologists read the rocks volcanoes produce to understand what happens before an eruption, which helps to predict future eruptions and their impact!
Volcanoes provide an exceptional display of natural beauty and power, which humans have the privilege to witness in their lifetime. However, they also remind us that, to fully enjoy nature, we also need to learn how to respect her. We can build a brighter future if we understand and respect the “rules” of nature! This means that we cannot build our houses and our important buildings (like schools and hospitals) too close to volcanoes in locations where they would likely be destroyed by an eruption. Many volcanoes do not erupt for many thousands of years, and so we have to understand why eruptions occur and how different volcanoes may behave differently. As scientists, we can understand the personality of a volcano from what it looks like and the rocks it produces. We need to understand and respect nature, as she can easily live without humans, but we cannot live without her!
Glossary
Magma: ↑ Molten rock rising from depth to feed volcanic eruptions.
Pyroclastic Flow: ↑ Hot mixture of gas, ash, and rocks of volcanic origin.
Lahar: ↑ Mud flow (water + volcanic material).
Lava: ↑ Magma that reaches the Earth’s surface.
Conflict of Interest Statement
The authors declare that the research was conducted in the absence of any commercial or financial relationships that could be construed as a potential conflict of interest.
References
[1] ↑ Walker, G. P. L. 1984. Downsag calderas, ring faults, caldera sizes, and incremental caldera growth. J. Geophys. Res. 89, 8407–16. doi:10.1029/JB089iB10p08407
[2] ↑ Gregg, P. M., de Silva, S. L., Grosfils, E. B., and Parmigiani, J. P. 2012. Catastrophic caldera-forming eruptions: thermomechanics and implications for eruption triggering and maximum caldera dimensions on Earth. J. Volcanol. Geother. Res. 241–242, 1–12. doi:10.1016/j.jvolgeores.2012.06.009
[3] ↑ Sumita, I., and Manga, M. 2008. Suspension rheology under oscillatory shear and its geophysical implications. Earth Planet. Sci. Lett. 269, 468–77. doi:10.1016/j.epsl.2008.02.043
[4] ↑ Caricchi, L., Annen, C., Blundy, J., Simpson, G., and Pinel, V. 2014. Frequency and magnitude of volcanic eruptions controlled by magma injection and buoyancy. Nat. Geosci. 7, 126–30. doi:10.1038/ngeo2041
[5] ↑ Newhall, C. G., and Self, S. 1982. The volcanic explosivity index (VEI) an estimate of explosive magnitude for historical volcanism. J Geophys Res Oceans Atmos 87, 1231–8. doi:10.1029/JC087iC02p01231
[6] ↑ Sheldrake, T., and Caricchi, L. 2016. Regional variability in the frequency and magnitude of large explosive volcanic eruptions. Geology 45, 111–4. doi:10.1130/G38372.1