What we perceive is not always what our eyes see. Vision, and perception more generally, should not be thought of as a webcam that just takes pictures of the world. This is not a fault in how our brains work, but rather is exemplary of how the brain constructs perception and takes advantage of its massive inter-connectedness in ways that are highly similar to social networks. The construction of perception is not only based on the information the eyes capture, but also based on the information stored in the brain and “guesses” based on this stored information. Illusory figure similar to that shown in Figure 1 is a laboratory example of this construction process and demonstrates well how the visual system works. In the real world, the visual system must handle situations of occlusion, noise, and equivocality (that is, when it is unclear what bits of what we see belongs to one object versus another).
If you were to describe what you see in the left part of Figure 1, you would most probably say that you see a white triangle on top of the three black disks. However, strictly speaking, only three segments of black circles (pac-men) are shown. The perception of the so-called Kanizsa triangle [1] is a very interesting phenomenon of the workings of our visual system (as well as that of other species from birds to monkeys). In the case of the Kanizsa triangle, the brain constructs the perception of the triangle and effectively fills in the lines between the mouths of the pac-men, resulting in the perception of a white triangle that seems brighter than the white paper (at least in the case of what is shown in Figure 1). In many cases, we see objects in the background (e.g., a cat) that are occluded by other objects in the foreground (e.g., a fence) (Figure 2). Nevertheless, we typically perceive a complete cat rather than parts of a cat that are separated by parts of a fence. Sorting out which parts belong to the same object is a challenging task for the human brain, since nerve cells in the eyes actually receive information only about specific and small parts of the cat; somewhat like individual pixels in a digital camera. The brain then has to construct what we see by putting together all the pixels in the right way; a process that is often helped by our experiences of what we expect to see.
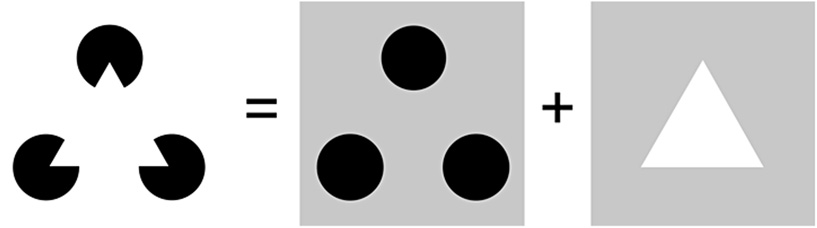
- Figure 1
- Kanizsa triangle (left of equal sign) and how it is perceived by our visual system (right of equal sign).
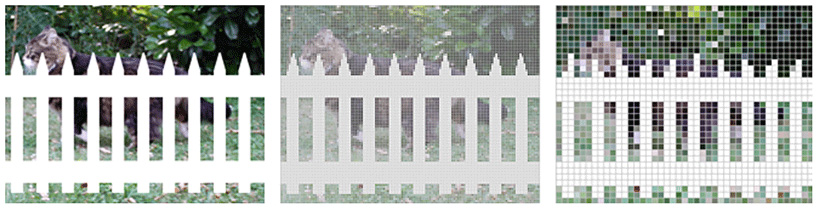
- Figure 2 - Example of an occluded object.
- Typically, we are able to perceive a cat behind a fence (left). However, the nerve cells in our brains only “see” single pixel of this image. Individual nerve cells with small receptive fields see fine details but only small parts of a whole object (middle). Nerve cells with larger receptive fields see larger parts of an object but with less detail (right). Then, the brain has to decide which pixels belong to the cat and which to the fence. One mechanism that helps to do so is that of connecting contours such as the back of the cat even when they are interrupted by the fence. Although this mechanism helps to see complete objects when they are occluded, it also leads to illusions such as the Kanizsa triangle.
Illusory figures have been used in neuroscience research in order to investigate this process of constructing visual perception [2]. The construction of visual perception by the human brain takes advantage of at least three features that all work together in concert.
First, there are the anatomical connections, i.e., the wiring. These connections not only describe the way information comes into the brain from the eyes, but also describe how information circulates around in the brain between “lower” and “higher” visual areas (Figure 3) so that things like your memories and past experiences of triangles and even the name “triangle” can help you perceive. These connections can be divided into those that go from the outside world into the brain in a manner much like a conveyor belt, which are known as feed-forward pathways because information goes from one station and is fed along the conveyor belt to the next station (gray arrows in Figure 3, bottom), and those that can go from one point inside the brain to another point inside the brain, which are known as feedback (and lateral or sideways) pathways (orange arrows in Figure 3, bottom).
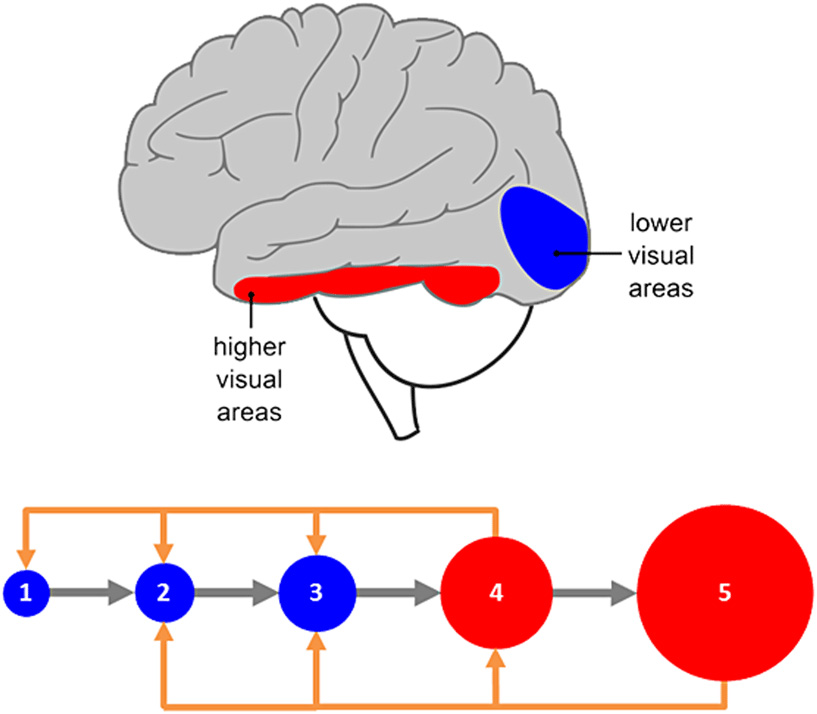
- Figure 3 - Organization of the visual system.
- Top: schematic view of the left hemisphere of a human brain. The front of the brain is displayed on the left, the back on the right. Lower visual areas receive visual information first and are located in the back of the brain (blue). Higher visual areas get the information later and can be found further toward the front of the brain (red). Bottom: illustration of feed-forward connections (gray) and feedback connections (orange) from higher visual areas (red) to lower ones (blue).
Second, there are so-called “receptive fields” of neurons, which can be more readily understood as akin to the size of pixels in a digital camera (Figure 2). Structures like the retina and primary visual cortex have very small receptive fields; their pixels are small and any individual neuron only “sees” a very tiny portion of a scene. As one progresses along the feed-forward anatomical chain from lower visual areas like V1 to higher visual areas, these receptive fields likewise get bigger (circles in the bottom of Figure 3). For example, some higher visual areas have receptive fields that are as big as your hand when you hold out your arm. Other higher visual areas have receptive fields that are as big as a whole house. As one might expect, the bigger the neuron's receptive field, the blurrier its “view” of the world. However, this is only partially true, because different neurons share information and “talk” to each other so that the image they collectively “see” can get sharper.
This brings us to the third feature – the timing of how information is communicated and shared along the wiring and receptive field architectures described above. On the one hand, information is processed in a serial and feed-forward fashion much like a conveyor belt where a car is assembled piece-by-piece at each station one after the other. Accordingly, information follows from the outside world to the eyes to lower visual areas (also called primary visual cortex or V1) located at the back of the brain and in turn to successive higher areas of the brain, with names such as V2, V3, and V4 (denoting the second, third, and fourth visual areas, respectively) that perform diverse and sometimes highly specialized visual functions (Figure 3). For example, neurons in V1 code for simple features such as straight lines. Neurons in V2 code for features like depth perception, which relies on combining information from both eyes. Neurons in V4 code for features, including basic shapes as well as color. In higher stage areas, some of which are located along the bottom of the temporal lobe of the brain (red area in Figure 3, top), code for complex shapes, such as faces or buildings.
On the other hand, information is also processed in a parallel and feedback fashion much like a social network or a subway system. The lines of a subway network can be understood as similar to connections between different parts of the brain; there is a complex mixture of direct and indirect routes between any two locations both in the subway network and in the brain. Intersections can be understood as hubs where information can be combined and integrated. Importantly and much like the subway system, information can go in the forward and backward directions, and different “lines” can run at different speeds all at the same time, resulting in combinations of express and local information routes. In this way, information can quickly “spread” to different locations of a network.
The processing of illusory contours demonstrates nicely how our visual system constructs what we see. Lower stages of processing become active first in detecting simple features of an image. But, these neurons do not “know” to which objects the detected edges, such as the mouths of the pac-men in Figure 1, belong. Only the higher stages of the visual system are able to achieve this task and are first in detecting complex objects. In this regard, these higher stages act as the foremen directing the construction of perception and using feedback to lower stages to perhaps refine the perceptions we ultimately experience. For more illusions and what they tell us about the brain, visit the webpage by Bach [3]. The magic of illusions is not so much in what we perceive (though that is in itself pretty cool), but rather in how they show off the capacity of our brains to shape perceptions.
Acknowledgements
This work was supported by grants to Micah M. Murray from the Swiss National Science Foundation (grants 310030B_133136 and 320000_120579 as well as the National Center of Competence in Research project “SYNAPSY – The Synaptic Bases of Mental Disease”; project no. 51AU40_125759) and to Christoph S. Herrmann from the German Research Foundation (grants SFB/TRR 31 and Hearing4all). We thank Toralf Neuling for designing Figures 1 and 3 (top).
References
[1] ↑ Kanizsa, G. 1976. Subjective contours. Sci. Am. 234:48–52. doi: 10.1038/scientificamerican0476-48
[2] ↑ Murray, M. M., and Herrmann, C. S. 2013. Illusory contours: a window onto the neurophysiology of constructing perception. Trends Cogn. Sci. 17:471–81. doi: 10.1016/j.tics.2013.07.004
[3] ↑ Bach, M. 2013. 105 Visual Phenomena & Optical Illusions. Available at: www.michaelbach.de/ot/