Abstract
Many of us enjoy listening to and even making music. Music moves us, inspires us, and just makes us happy. It can become an important part of who we are. Making music is also good training for the brain, especially if you start young and stick with it. Musical training can improve the way the brain processes all sounds, including speech, which can help with hearing and communication, especially in noisy places. It can also improve how other senses, such as touch and vision, work together with hearing to paint a complete picture of the world. It is important, however, to avoid listening to music so loud that it damages your ears. If you ever experience ringing in your ears (called tinnitus), even for a short time, you are risking some permanent damage to your ability to hear. So if you love listening to your music player, having band practice, or going to dances or concerts, it is important to try to keep the volume of the music down at a safe level. Damage due to loud music (or loud noise in general) can be quite minor at first and difficult for even skilled doctors to detect, but it can build over time and noticeably decrease your ability to hear as you get older.
When you learn to play an instrument – whether a piano, guitar, violin, trumpet, or drum – you simultaneously engage a large number of brain areas. These include areas responsible for sensation (hearing, touch, and vision), movement, and “cognitive functions” such as concentration, memory, reasoning, and decision making. Over years of training, these brain areas become better connected and you are able to learn and perform longer, more complex pieces.
Modern neuroimaging allows us to look at both the structure and function of the brain without even scratching the skin. These non-invasive scans reveal differences between musicians and non-musicians in a variety of brain regions. Such differences are related to musicians being better than non-musicians in a number of auditory, multi-sensory, and cognitive areas. I will give some specific examples below. Importantly, the biggest differences were found in the musicians who had been training the longest and who had started playing in preschool or in the first years of elementary school. This suggests that musical training itself is likely to be the source of the brain differences and the sensory and cognitive benefits, rather than a natural (genetic) gift that musicians have and others do not.
Brain Basics
The human brain is arguably the most complex structure known to science. A century ago, the German Korbinian Brodmann made the first serious attempt at cataloging this complexity. He divided the cerebral cortex, the biggest and uniquely mammalian part of the brain, into about 50 regions based on their anatomical appearance. These are now known as “Brodmann areas” (Figure 1). In modern neuroscience, we understand that some of these anatomical regions have similar functions, while others play multiple roles. For example, Brodmann areas 39, 40, and part of 22 together make up “Wernicke’s area,” named after Carl Wernicke, another German. Wernicke’s area is crucial for our seemingly effortless ability to make sense of words (as well as other forms of communication, such as sign language). When scientists first studied brains, they noticed that some parts were a bit darker in appearance than others, and called these “gray matter” and “white matter.” We now know that gray matter is made up of billions of nerve cells or neurons. White matter consists of the neural cables or axons that connect different brain regions.
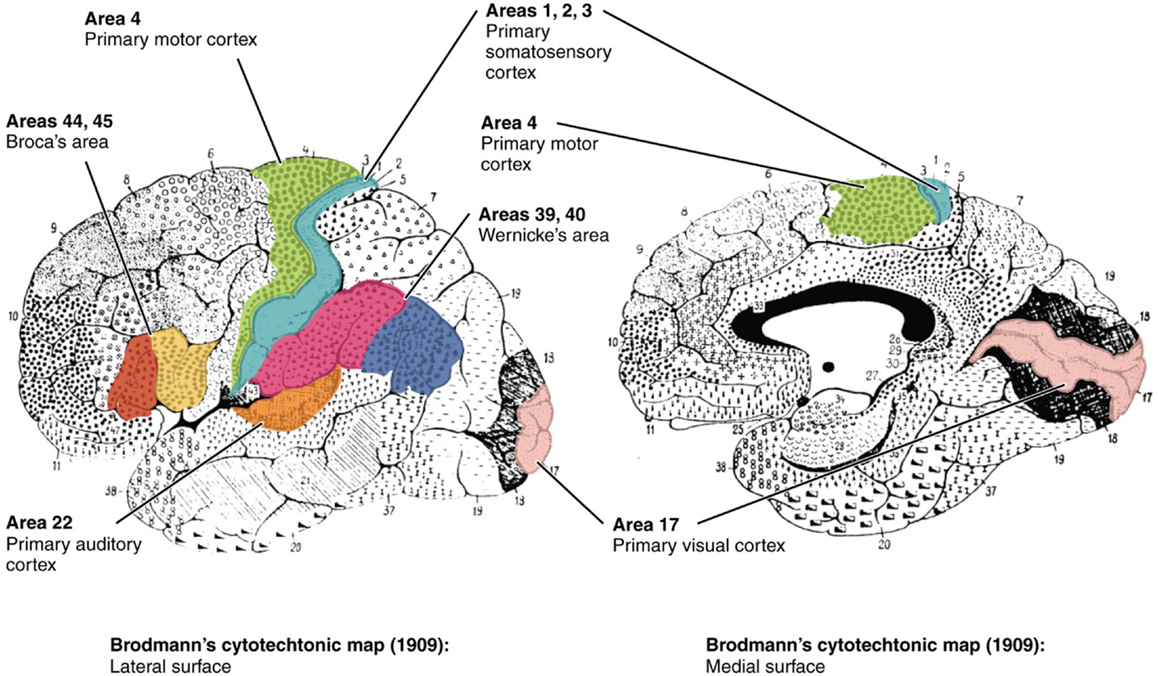
- Figure 1 - Brodmann areas of the cerebral cortex, with some key areas shaded in.
- Downloaded from Wikipedia (http://en.wikipedia.org/wiki/Brodmann_area#mediaviewer/File:1307_Brodmann_Areas.jpg).
What are the Differences between the Brains of Musicians and Non-Musicians?
It appears that musicians’ brains have more gray matter in certain areas, and are better connected with more white matter. The areas with more gray matter include parts of auditory, somatosensory (touch), and motor (movement) cortex, parts of the frontal cortex (involved in cognitive functions), cerebellum (involved in the coordination of complex learned movements), and – in vocalists – Broca’s area (involved in speech production). More white matter is seen in the connections between temporal (sensory) and frontal (cognitive) cortex, and in the corpus callosum, the thick axonal bridge that connects the left and right halves of the brain. These structural changes may help explain the increase in neural activity that functional scans have detected in musicians (compared to non-musicians) as they listen to and distinguish between the various sound elements of music and speech [1, 2].
What are the Advantages of These Differences in Brain Structure?
Having more neurons in – and better connections between – these brain regions allows musicians to more efficiently and accurately process information from their senses and send motor commands to their muscles. Musicians are better at noticing small differences in the timing and tonal quality (frequency spectrum) of both music and speech sounds. They are also better at understanding speech in noisy settings, such as a room full of people talking and laughing. Beyond hearing, musicians have better short-term memory (again, in group comparisons to non-musicians), more nimble hands and fingers, and are better on tasks that combine audio and visual processing, such as lip-reading [1, 2]. Of course, music is just one of many activities that provide great stimulation for the body and mind. Reading, writing, drawing, thinking and problem solving, exploring, playing games, and sports – these are all fun activities with potential benefits.
The advantages of musical training can last throughout a person’s life. They can even make up for some of the negative effects that loud noise (our next topic) and aging can have on hearing. Older musicians with some hearing loss can understand speech significantly better than non-musicians of similar age and with a similar amount of hearing loss.
Why is the Ear so Vulnerable to Loud Music, and to Loud Noise in General?
Music is enjoyable and useful for brain development, but loud music can permanently damage your ears, which can counteract music’s benefits, or worse. To estimate the loudness of many every day sounds, take a look at Figure 2. Sound intensity is measured in units called decibels (dB) and more intense sounds are heard as being louder. You can download the answer key at http://www.turnittotheleft.org/educational/Audio_Quiz_KEY.pdf. According to Figure 2, sounds in the 90–120 dB range – which can be reached by the average music player, rock concert, or marching band – can permanently damage hearing in about 30 min. Let us take a look at why the ear is so vulnerable to loud sound.
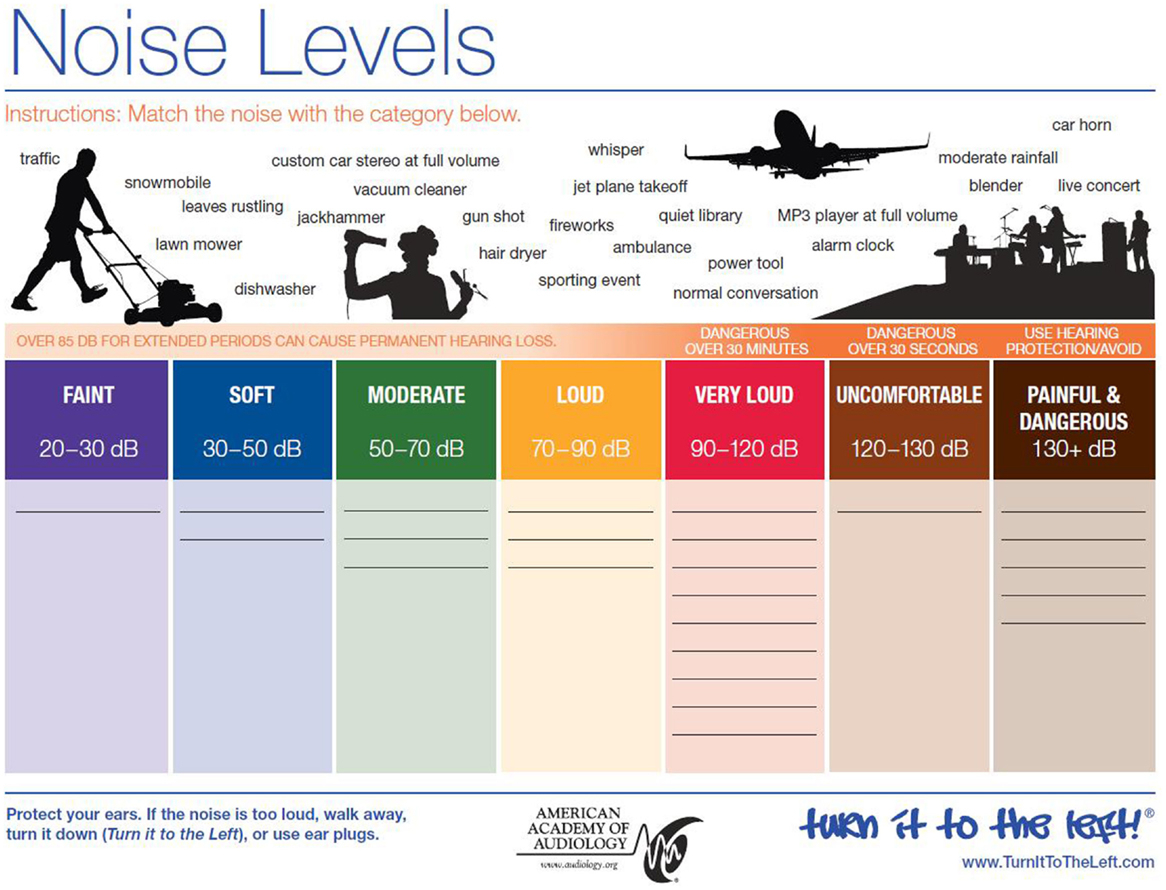
- Figure 2 - Fill in the intensities of some everyday sounds.
- Find the answers at: http://www.turnittotheleft.org/educational/Audio_Quiz_KEY.pdf. Courtesy of the American Academy of Audiology.
The ear can be divided into three distinct parts: the outer, middle, and inner ear. As the next few paragraphs follow the path a sound takes through these parts of the ear, refer to Figure 3.
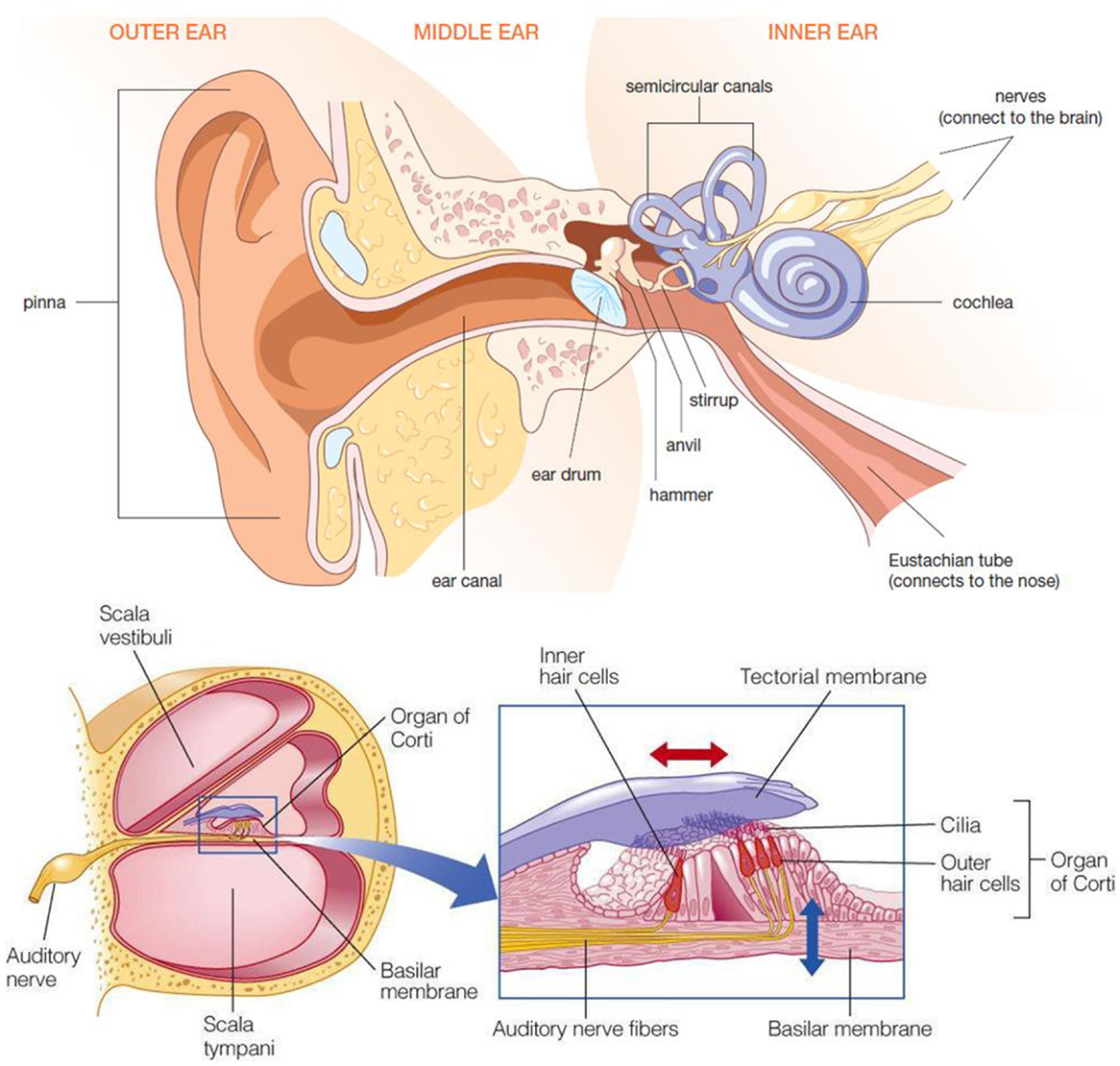
- Figure 3 - Anatomic drawings of the outer, middle, and inner ears (top), cross-section through the cochlea (bottom-left), and close-up of the organ of Corti (bottom-right).
- The main text refers to this anatomy to outline the physiology of hearing. Courtesy of the American Academy of Audiology.
Starting from the outer ear, the pinna funnels sound into the ear canal, which makes the eardrum vibrate in and out. The eardrum is attached to a chain of three tiny bones in the middle ear (nicknamed the hammer, anvil, and stirrup), which transmit eardrum vibrations into the water-filled cochlea (Latin for “snail”) of the inner ear. If the middle ear did not exist and the eardrum was connected directly to the cochlea, most of the sound energy would be reflected back from the eardrum into the ear canal and out through the pinna because water is much more dense (heavier) than air. The same thing happens when a small and light marble recoils when it hits a big and heavy marble, while the big marble moves forward only a little. The middle ear gives airborne sound (i.e., the small marble) a boost so that it can more forcefully vibrate the water column (big marble) in the cochlea. Vibration of this water column (which fills the scala vestibuli and scala tympani of the cochlea) causes the basilar membrane to wiggle up and down (blue double-sided arrow in Figure 3).
Sitting atop this basilar membrane are the inner hair cells, which sense the sound and send information about it to the brain. The inner hair cells are housed in a complex sub-structure of the cochlea named the organ of Corti, after its discoverer, the Italian Alfonso Corti. As sound causes the basilar membrane to wiggle up and down, tiny hairs (or cilia) on the inner hair cells sway back and forth (red arrow). The swaying opens tiny pores (ion channels) in the cilia, allowing signaling molecules (mostly potassium ions) to enter the hair cells. The amount of potassium that enters each of the roughly 3,500 inner hair cells (in a healthy cochlea) at a given point in time tells the ear much about the loudness, type, and origin of the sound. This information is translated into bursts of electrical impulses (action potentials or spikes) in the auditory nerve, which travel to the brain for further processing and recognition.
There is one more important piece of this complex hearing puzzle: the three rows of outer hair cells (Figure 3). These cells respond to their swaying cilia by physically amplifying the sound, making it louder and more distinct for the inner hair cells. Outer hair cells are really electrical motors in disguise – they can vibrate rapidly and in step with the incoming sound to amplify it.
It is an exquisitely precise system, but unfortunately this comes with a price. To be better at detecting and amplifying incoming sound, the cilia of the outer hair cells are attached to the floppy tectorial membrane (Figure 3), which gives them a firm anchoring point for swaying back and forth. However, it also makes them prone to tearing when the sound gets too loud and the vibrations become too big. When the cilia tear, the outer hair cell is often damaged beyond repair and is permanently lost. If enough outer hair cells are lost (each ear starts with about 12,000), quiet sounds will become inaudible and moderate sounds will become quiet and less clear.
Inner hair cell cilia are not attached to the tectorial membrane and so are less vulnerable to tearing damage. However, inner hair cell synapses, which are their points of contact with the auditory nerve fibers, are also very vulnerable to loud noise, and can be destroyed by toxic molecules released in the course of excessive noise stimulation. If a synapse is destroyed, its auditory nerve fiber can degenerate and be lost. There are about 30,000 auditory nerve fibers in a healthy ear so as long as the losses are not too large, your ability to hear may not suffer at first. However, after repeated noise exposures, you may begin to notice that your hearing is not as good as it used to be. This would first be noticeable only in noisy environments where things are harder to hear, but eventually also in quiet environments. Recent experiments in several animal species (mice and guinea pigs) have shown that a single 2-h noise exposure in the 100 dB range (refer to Figure 2) can do significant and permanent damage to the ear [3], and that several such exposures delivered a few weeks apart can cause even more severe damage [4].
How Can You Tell What’s Too Loud?
Some people’s ears can tolerate loud sounds a bit better than others, and guidelines such as “90–120 dB for no more than a half hour” are very rough. A good rule of thumb is to avoid noise exposures that leave your ears ringing afterwards, even if only for a relatively short time. This temporary tinnitus usually occurs with a temporary loss of hearing sensitivity, which makes things sound a bit muffled for a while. The problem is that animal studies [3, 4] show that sounds loud enough to cause these “temporary” problems are actually doing permanent damage that is difficult to detect at first because of the relatively large starting number of auditory hair cells and nerve fibers. So be careful, avoid very loud music and noise, and if you cannot, wear hearing protection such as ordinary foam or special “musician’s” earplugs [5].
References
[1] ↑ Herholz, S.C., Zatorre, R.J. 2012. Musical training as a framework for brain plasticity: behavior, function, and structure. Neuron 76:486–502. doi: 10.1016/j.neuron.2012.10.011
[2] ↑ Moreno, S., Bidelman, G.M. 2014. Examining neural plasticity and cognitive benefit through the unique lens of musical training. Hear. Res. 308:84–97. doi: 10.1016/j.heares.2013.09.012
[3] ↑ Kujawa, S.G., Liberman, M.C. 2009. Adding insult to injury: cochlear nerve degeneration after “temporary” noise-induced hearing loss. J. Neurosci. 29:14077–85. doi: 10.1523/JNEUROSCI.2845-09.2009
[4] ↑ Wang, Y, Ren, C. 2012. Effects of repeated ‘benign’ noise exposures in young CBA mice: shedding light on age-related hearing loss. J. Assoc. Res. Otolaryngol. 13:505–15. doi: 10.1007/s10162-012-0329-0
[5] ↑ Chesky, K. 2011. Schools of music and conservatories and hearing loss prevention. Int. J. Audiol. 50:S32–7. doi: 10.3109/14992027.2010.540583