Abstract
Nature is the source of many fascinating colors, which have been the subject of extensive research by biologists, chemists, physicists, and other scientists. Under the microscope, scientists discovered something interesting: some colors arise from chemicals, while others result from the interaction of light with tiny structures. The second type have incredible properties, especially their ability to stay the same, so that their colors do not fade over time. For scientists, understanding how this process works is crucial if they want to replicate such colors in their labs. By unlocking this knowledge, they can use it to create new materials that have any color of the rainbow, just by playing with their structures.
Have you ever tried playing Twister in front of a campfire or in a dim room? If so, you may have noticed that it is difficult to figure out which circle is blue and which one green… while you stand upside down on your left foot and your right hand, trying to decide where to place your left hand next! What causes our eyes not to see colors anymore when there is little light? To answer this question—and to know how to win during a fire-lit game night—we must first understand what makes things a certain color.
What is the “Recipe” for Color?
We all know that we can mix yellow and blue paint to make green, yellow and red to make orange, or red and blue to make purple. Mixing all the colors together creates an ugly brown color. How does this color mixing work? The paints in your paintbox are a concentrated mix of pigments, special molecules with amazing colors. When we blend these paints, we combine their molecules, resulting in a color that is the sum of the individual hues. But there is a catch: to see the color, we need light. Light is therefore a crucial ingredient of color.
Sunlight contains all the colors (Figure 1A). We witness this when looking at a rainbow after a rainfall—water droplets split the rays of sunlight hitting them into all of the light’s different colors. What is the difference between red light and blue light? When light moves, it does not do so in a straight path. It moves up and down, forming a wave, and the distance between the peak of each wave remains constant. This length is called the wavelength, and each color of light has a distinct, specific wavelength (Figure 1A).
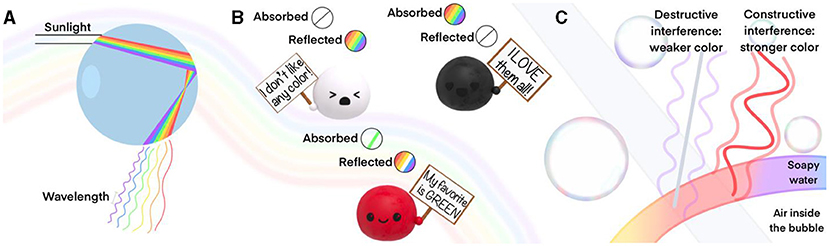
- Figure 1 - (A) A water droplet breaks down sunlight into the different colors it contains, each with their specific wavelengths.
- (B) Pigments interact with light to create their colors. White reflects every color and absorbs none; black reflects no colors and absorbs all; red absorbs green and reflects all the others, which sum up to make red. (C) Interference in a soap bubble. When two waves “match” and sum up, we see a more intense color (constructive interference). When two waves cancel each other, we cannot see the corresponding color (destructive interference).
Pigments interact with light, and their interaction is perceived as color by our eyes. Depending on what the pigment is made of, it absorbs certain wavelengths of light and reflects others. The result? As the colors it absorbs are now missing from the reflected light, the material displays the complementary color to the one that is most strongly absorbed (Figure 1B). For example, the materials we see as red actually absorb the green color and reflect all the others, which together give rise to the red appearance. There are two extremes. White pigments do not absorb any color and instead reflect all wavelengths. Black pigments absorb all colors, leaving none for us to enjoy.
The Off-Menu Special
Not all colors in nature are due to the presence of pigments. The colors observed in the shiny wings of blue butterflies, in the beautiful tails of peacocks, and in hummingbirds come from a different phenomenon. On their wings and feathers, these creatures have tiny structures that interact with sunlight to create their colors.
This might sound very complex, but it is not. It is the same phenomenon that you observe in a soap bubble. When you make a soap bubble, you start with a clear soap solution and make a very colorful, iridescent bubble (Figure 1C). Intriguingly, if you observe a soap bubble closely, you will see that the predominant colors change from yellow/reddish to blueish over time. Right before it pops, it becomes almost transparent. When we blow a bubble, we create a very thin layer of soapy water filled with air. When a material is as thin as the shell of a soap bubble, it can reflect color, depending on its thickness. And guess what—the thickness changes over time and, along with it, the color of the bubble changes too. When the layer becomes too thin, no color is observed. How can a simple layer of soap do that? It takes advantage of the wave behavior of light.
Light waves behave much like the waves that appear when you throw a pebble in a calm lake. The waves can either cancel out or add up, depending on how they meet and what their wavelengths are. If they add up, they become stronger and increase the intensity of a specific color. If they cancel out, the color disappears. This phenomenon is called wave interference (Figure 1C). When the waves sum up, it is called constructive interference; and when waves cancel out, it is called destructive interference.
What does this mean for our soap bubble? The delicate, soapy water film that makes up the bubble has two surfaces—the one on the outside of the bubble and the one on the inside. Light waves are reflected from both surfaces (Figure 1C). Any wave with a wavelength that just “fits into” the film comes out in step with a wave that is reflected from the outside of the bubble. These waves are said to be in phase. Such waves overlap and produce constructive interference, which strongly enhances their colors. As time goes on, the thickness of the soap film creating the bubble gradually gets thinner. This means that other wavelengths of light will “fit” perfectly into the thin film, and therefore, other colors will be enhanced. If you watch closely, you will see that the soap bubble eventually becomes transparent—it is now so thin that even the smallest wavelength of a visible color cannot fit into the thin film, so no color is enhanced anymore.
Nature uses the same principle to make the special iridescent colors found in butterflies, peacocks, and other animals. If we look at a blue Morpho butterfly wing under a microscope, we see a lot of tiny scales (Figure 2). Each scale seems to have ridges on top of it. If we zoom in even further, we can see that every ridge is shaped like a small Christmas tree, whose branch distance is similar to the wavelength of blue light. When light interacts with one of these branches, it is reflected in a manner similar to what happens within a soap bubble. Since the distance between the branches is constant, only the wavelength corresponding to the blue color will have constructive interference. This is how the butterfly makes its blue color without using any pigment!
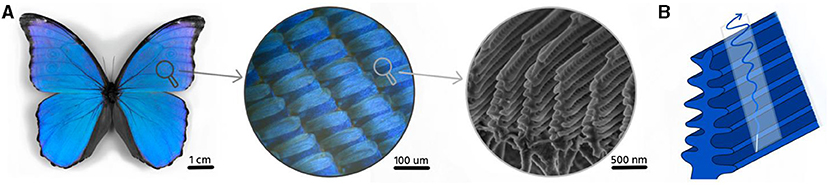
- Figure 2 - (A) Morpho butterfly wing seen at two different magnifications.
- You can see the multiple scales that compose the wing and the structure on top of each of the scales. (B) Light interacts with the structure of the butterfly wing to give rise to its blue color. Since the distance between the branches is specific and constant, wavelength corresponding to blue light become stronger (constructive interference) with the others cancel out (destructive interference).
The strategy used by the butterfly offers a significant advantage over pigments. Have you ever noticed how old family pictures fade? Their colors are made out of pigments, and pigments break down when they are in the sun for too long. In pictures, this is called pigment bleaching, and it causes the picture to fade. However, the butterfly keeps its vibrant color as long as its structure is not damaged. What is even more remarkable is there are million-year-old fossilized animals that still have a shiny, colorful appearance [1].
Easy as Making a PB&J Sandwich
The fascinating trick used by the butterfly has caught scientists’ attention—they are always looking for new, clever strategies to make new materials in their labs. Using strategies found in nature to develop new materials and technologies is called bioinspired design. Can scientists produce the same structure as on the butterfly wing but from a different material in the lab? Scientists can create materials by putting one layer on top of another and, as long as they can control the layer thickness and composition, they can create colored structures without using pigments. This is a bit like making a peanut butter and jelly sandwich (Figure 3A). To make a PB&J, you start with a slice of bread and carefully spread a layer of peanut butter and then a layer of jelly, making sure it is as even as possible, to get the perfect ratio in each bite. In the lab, scientists can create the simplest structural colored materials with only a couple of layers of colorless materials, as long as the materials have a thickness similar to the wavelength of colored light. By doing this, they create a colored material that does not bleach (Figure 3B).
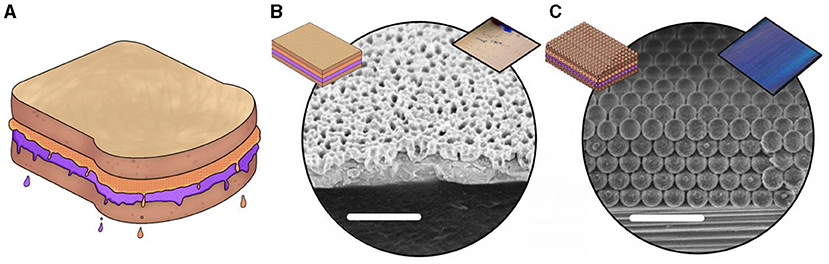
- Figure 3 - (A) A PB&J sandwich drawing.
- (B) A microscope image of a layered material. (C) A microscope image of a particle-based material. Both materials consist of layers, like a sandwich, with a thickness similar to the wavelength of colored light—so they appear colorful. The insets at the top left of each image show a schematic of the structure, and photographs of the materials, showing their colors, can be seen on the top right.
Once they master the basic recipe, scientists can experiment and create new ones. For example, they can make layers out of small, colorless glass particles—spherical objects that pack together and that have a similar size to the wavelength of visible light (Figure 3C).
Scientists can then build on these principles to create materials for interesting applications. Researchers have learned from chameleons to make materials that change color when stretched [2]. To do so, they made the layers of their PB&J sandwich elastic. When they pull on the material, the layers get thinner. As you have already learned from the soap bubble, the color now changes. This technique can be used to create a shirt that flashes in different colors when you stretch your muscles, but it can also tell medical doctors if a bandage is properly applied to a patient [3]. These are just a couple of examples of what can be achieved by imitating colors in nature, but further applications are being developed. For example, this technology can be used to reveal toxic gases in the air or chemicals in liquids, by making a material that changes color when a danger is present.
Even though similar technologies can be achieved with pigments, they will fade with use and not withstand the test of time. Therefore, taking inspiration from nature will help scientists to improve many important materials. In fact, nature is the best source when it comes to finding new ideas to create new materials. Nature specializes in fancy, unique colors, and, if scientists manage to get a copy of its “secret recipes”, they can put them in their “cookbooks” and use them to develop original strategies to include in their very own “menu” of materials.
Glossary
Pigments: ↑ Molecules displaying a specific color.
Wavelength: ↑ The length from the top (or bottom) of one wave to the top (or bottom) of the next. Each wavelength of light corresponds to a color of the rainbow.
Iridescent: ↑ A material that changes color depending on the direction we look at it from.
Constructive Interference: ↑ When the top of two waves overlap and the wave becomes stronger.
Destructive Interference: ↑ When the top of a wave overlaps with the bottom of another and the wave becomes weaker.
Bioinspired Design: ↑ The art of picking a property of the natural world, carefully analyzing the way it works, and recreating it in the lab to make a material mimicking the desired property.
Conflict of Interest
The authors declare that the research was conducted in the absence of any commercial or financial relationships that could be construed as a potential conflict of interest.
Acknowledgments
This work was supported by the Deutsche Forschungsgemeinschaft (DFG, German Research Foundation)—Project-ID 416229255–SFB 1411, by the European Research Council under the European Union’s Horizon 2020 research and innovation program (EYESPOT—grant agreement no. 101000504), and by the Portuguese Foundation for Science and Technology (FCT) research contracts to MC (CEECINST/00014/2018/CP1512/CT0002) and PA (2020.01405.CEECIND/CP1601/CT0011).
References
[1] ↑ McNamara, M. E., Briggs, D. E. G., Orr, P. J., Noh, H., Cao, H. 2012. The original colours of fossil beetles. Proc. R. Soc. B 279:1114–1121. doi: 10.1098/rspb.2011.1677
[2] ↑ Schäfer, C. G., Winter, T., Heidt, S., Dietz, C., Ding, T., Baumberg, J. J., et al. 2015. Smart polymer inverse-opal photonic crystal films by melt-shear organization for hybrid core–shell architectures. J. Mater. Chem. C 3:2204–2214. doi: 10.1039/C4TC02788D
[3] ↑ Sandt, J. D., Moudio, M., Clark, J. K., Hardin, J., Argenti, C., Carty, M., et al. 2018. Stretchable optomechanical fiber sensors for pressure determination in compressive medical textiles. Adv. Healthcare Mater. 7:1800293. doi: 10.1002/adhm.201800293