Abstract
If you could time travel to the central U.S. 300 million years ago, you would find yourself at the equator of the supercontinent Pangea. At first you might enjoy a warm climate, surrounded by seas filled with life. But, after some millions of years, the seas would vanish as the climate turned increasingly hot, dry, and hostile. Billowing dust would engulf you, and nearly all life on Earth would vanish in an event called the Great Dying. How do we know? Geoscientists reconstruct past landscapes and climates by drilling into ancient sediments—tiny grains of sand and silt. These tiny particles tell us how fast the mountains rose and which way the wind blew. Microscopic fossils reveal water and air temperatures. And miniature bubbles trapped in salt preserve actual fossil water, from nearly 300 million years ago. Travel back in time with us to explore the Great Dying.
Earth’s Climate: Past, Present, and Future
Around 250 million years ago, during the Permian geologic time period, there was a mass extinction event called the Great Dying [1], during which life on our planet almost came to an end. Ninety percentage of life in the oceans and 70% of life on land vanished. Changes in Earth’s climate system leading up to this event were very extreme. There was dramatic global warming and a huge change in Earth’s atmosphere and the oceans. This time period is the only example we have of the complete melting of Earth’s ice caps during a time when there was a diverse and healthy ecosystem on land. How do we know all of this?
Sedimentary rocks record the history of climate change, even climate change that happened hundreds of millions of years ago. Learning about the past helps us understand the processes that cause the climate to swing in different directions. This helps us prepare for the future and how our planet might respond to climate change. For example, Earth currently has ice caps at both the north and south poles, and researchers have been looking to the Permian to learn more about how our planet and the life it hosts might change in response to the melting of those ice caps. However, it is important to remember that the future is not a direct copy of the past.
Clues From Cores: The Rocks Tell the Story
The rocks tell the story. For example, if the climate was dry and dusty, the dust fell to the ground as a record of that dusty time. Or, if seawater covered the land, shells and bones of ocean animals settled down onto the seafloor and recorded hints of what the sea was like. These particles settled into layers, became buried by more layers, and, over millions of years, hardened into sedimentary rock. Today, geoscientists can drill into these rock layers and remove a core sample. A core sample is a long cylinder of rock only a few cm (or inches) wide that can be more than 1.5 km (a mile) long! Core samples are collected using a big, tower-like drill that is operated by a large crew of people (Figure 1). Geoscientists can read the story of how the climate and landscape changed over this very long time in the layers of rock in the core. The oldest material, and therefore the beginning of our story, is at the bottom of the core. The youngest, or most recent part of the story, is at the top, closest to the Earth’s surface. Our story begins in ancient Oklahoma.
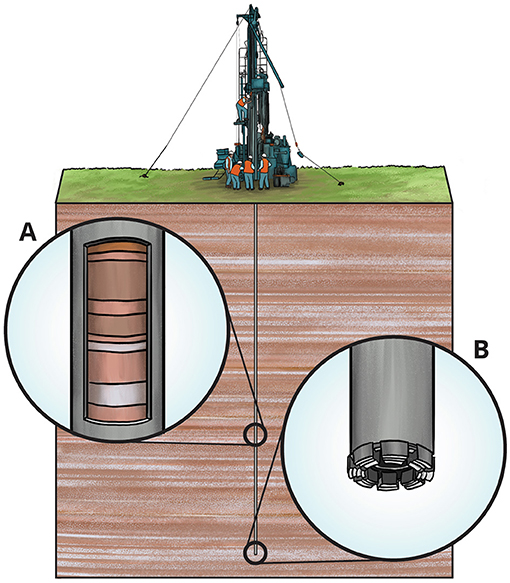
- Figure 1 - A drill rig at Earth’s surface digs into the rock below.
- (A) Cut-away view of the rock core inside the drill pipe. This is the sample that will be pulled to the surface for geologists to study. (B) The drill bit at the end of the long pipe has tiny diamonds in it that are hard enough to cut through rock. The hole in the middle of the drill bit cuts a circle in the rock, leaving a long cylinder of rock inside the pipe—this is the core sample.
Project Deep Dust: Rock Core From Equatorial Pangea
The shape of Earth’s land has changed over time because continents are constantly moving. If you could time travel to the central U.S. 300 million years ago during the Early Permian, you would find yourself at the equator on the supercontinent named Pangea. This massive continent included what we now know as North and South America, Africa, Europe, Antarctica, Australia, India, and Siberia—combined (Figure 2). The enormous forces that pushed the continents together caused mountains to rise.
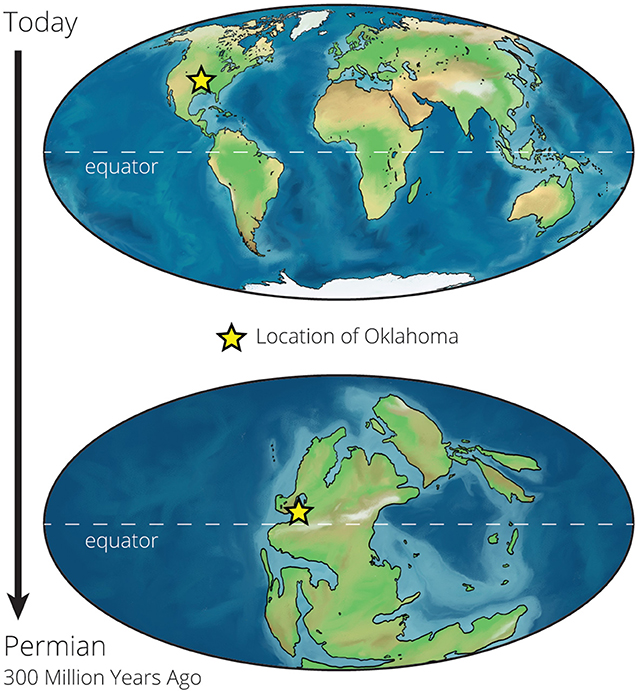
- Figure 2 - Continents are always moving.
- These maps show how the shape of the continents 300 million years ago, during the Permian period (bottom), was very different from their shape today (top).
These mountains arose in the center of this supercontinent, along a line that stretched from Europe and northwest Africa, along eastern North America, and into central North America—what is today Oklahoma. Layers of sedimentary rock formed from tiny grains of sand and dust that washed down from these ancient mountains millions of years ago.
Our team is planning an expedition to collect new rock cores from Oklahoma, to learn about how and why the climate changed during the Permian. We are naming our project Deep Dust because we plan to drill deep—over 1,500 m—into rocks made of ancient dust [2], which provide a continuous record of how life and climate at the equator of Earth’s largest continent evolved through a time of dramatic planetary change. What do we hope to find in these cores? Let us start with some history of the past.
Equatorial Pangea in the Past
During the Early Permian period, the Earth cycled between cold glacial periods and warm interglacial periods. Ice caps grew during glacial periods, which caused sea levels to drop, sometimes over 100 m (100’s of feet), as Earth’s water was locked away in ice. During this time, equatorial Pangea was home to a rich coastal ecosystem busy with insects, amphibians, and reptiles. As ice melted during warm interglacial phases, sea level rose, and the area transitioned to a shallow sea overflowing with life. Sea creatures covered the seafloor, and ancient animals swam through the warm waters. As the climate cycled through these warm interglacial and cold glacial periods, sea level rose and fell accordingly. The diverse ecosystems shifted back and forth as the animals and plants migrated along with the shoreline.
Over tens of millions of years, the heat of Earth’s interior began to build up beneath the giant supercontinent. This heat caused the land to rise, and the shallow seas disappeared from equatorial Pangea’s shores. Air temperatures began to rise, ice melted, and the supercontinent dried out, creating large deserts across the equator. Extreme winds ripped across the land as the climate turned increasingly hot, dry, and hostile. Salty, acidic lakes and cracked earth covered the landscape [3]. Billowing dust storms engulfed the region, and nearly all life on Earth vanished in the Great Dying. What does this look like in a core sample?
The Bottom of the Core: Early Permian
Rocks at the bottom of the core from Oklahoma tell us about the beginning of the Permian period. We find layers of rock that formed in oceans, on land, and in lakes (Figure 3). These layers formed as the sea level rose and fell. We also find fossils. In rocks that formed in lakes, fossils of insects are found [4]. Although a core sample might not have the bones of an entire fish, there are many fossils of tiny sea creatures in the cores (Figure 3). These little creatures were the shape and size of a grain of rice and lived in shallow, clear water. Fossils of ancient marine animals found in the cores paint a picture of healthy and diverse ecosystems in equatorial Pangea 300 million years ago, when shallow seas were common.
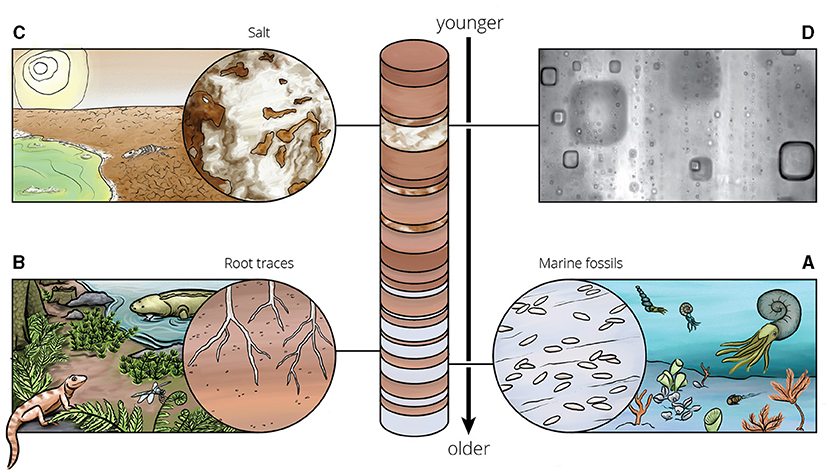
- Figure 3 - In a core, the oldest rock is at the bottom and the youngest is at the top.
- (A) Marine fossils from the early Permian are evidence of a healthy ocean ecosystem. (B) Ancient soils from the early Permian supported a diversity of insect, reptile, and amphibian life. (C) Salt found in later Permian rocks indicates a dry, hostile environment. (D) This microscope image shows the square pockets of fossil water trapped in salt that record temperatures of over 150°F!
Not all fossils are the remains of ancient animals. During the cooler glacial periods, the seas disappeared, and a different kind of fossil formed—ancient soil. Soil is material at Earth’s surface that supports plants and other life. Traces of roots from Permian plants can be seen in ancient soils. These soils can also be identified by the minerals they contain. For example, ancient soils with a lot of clay suggest the area was warm and wet and could support healthy plant life (Figure 3).
The Middle and Top of the Core: A Gradual Change
Higher in the cores, we see different kinds of rock. This represents a time closer to today, during the middle Permian. The marine fossils and deep soils are gone. Instead, we see minerals that form when water dries up, like salt (Figure 3). If you leave a glass of salt water out in your kitchen and wait for the water to dry out, you find crystals of salt left behind. And if you look closely enough, you might notice the salt crystals form perfect cubes.
The salt in the cores of the middle Permian formed as the region dried to become a desert, and salty lakes formed. As the salt crystals grew, they trapped tiny pockets of lake water. Because the salt grows as a cube, the pockets of water in the crystals also form cubes, which look like squares under the microscope (Figure 2). The tiny square bubbles preserve ancient water that dates from nearly 300 million years ago! Today, we can study this ancient water and learn about air temperatures when the crystals formed. From this, we know that the surface temperature reached over 73°C (163°F) during the day at the equator in this interior region of Pangea [5]!
Eventually the climate became too hot and dry for even salt to form. By the end of the Permian, only wind-blown dust is present in the cores [6]. The rocks provide a glimpse of this dusty and dangerously hot landscape in the center of Pangea.
Becoming a Geoscientist
Project Deep Dust will collect new core samples from Oklahoma to help us learn details about how and why the climate changed during the Permian—but you do not need a giant drill to learn about Earth’s past. All the rocks around you can tell stories if you learn how to read them. Learning about rocks is more than just identifying and sorting them into groups. If you become a geoscientist, you can use rocks to read the story of Earth’s past—from thousands to hundreds of millions or even billions of years ago—including fossils that show how life on Earth has changed through its history. Study of the “deep” past gives us a look at the alien landscapes of ancient Earth.
Glossary
Sedimentary Rocks: ↑ Form when small particles of minerals, ancient animals or plants, or other rocks are bonded together.
Geoscientist: ↑ A Geoscientist is a person who studies some part of the Earth and planetary systems—the air, water, rocks, soils, and ice—and how humans interact with these systems.
Core: ↑ A core sample is a cylinder of rock or mud collected by inserting a long hollow tube or pipe into the Earth’s surface and removing the materials inside.
Supercontinent: ↑ A supercontinent is a giant landmass that includes many or all of the existing continents on Earth today.
Glacial Period: ↑ A glacial period is a time in Earth’s history when glaciers and ice sheets are common on Earth’s surface.
Interglacial Period: ↑ An interglacial period is a time in Earth’s history when glaciers and ice sheets are rare on Earth’s surface.
Soil: ↑ Is the uppermost layer of the Earth’s surface that supports plant life.
Minerals: ↑ Are non-living solids that have unique organized internal structures and occur naturally on Earth.
Conflict of Interest
AM is employed by Amiable Consulting.
The remaining authors declare that the research was conducted in the absence of any commercial or financial relationships that could be construed as a potential conflict of interest.
Original Source Article
↑Soreghan, G. S., Beccaletto, L., Benison, K. C., Bourquin, S., Feulner, G., Hamamura, N., et al. 2020. Report on ICDP Deep Dust workshops: probing continental climate of the late Paleozoic icehouse–greenhouse transition and beyond. Sci. Dril. 28:93–112. doi: 10.5194/sd-28-93-2020
References
[1] ↑ Erwin, D. H. 2006. Extinction: How Life on Earth Nearly Ended 250 Million Years Ago (Princeton, NJ: Princeton University Press). p. 320.
[2] ↑ Soreghan, G. S., Beccaletto, L., Benison, K. C., Bourquin, S., Feulner, G., Hamamura, N., et al. 2020. Report on ICDP Deep Dust workshops: probing continental climate of the late Paleozoic icehouse–greenhouse transition and beyond. Sci. Dril. 28:93–112. doi: 10.5194/sd-28-93-2020
[3] ↑ Benison, K.C., and Goldstein, R. H. 2001. Evaporites and siliciclastics of the Permian Nippewalla Group, Kansas and Oklahoma: a case for nonmarine deposition. Sedimentology 48:165–88. doi: 10.1046/j.1365-3091.2001.00362
[4] ↑ Giles, J. M., Soreghan, M. J., Benison, K. C., Soreghan, G. S., and Hasiotis, S. T. 2013. Lakes, loess, and paleosols in the Permian Wellington Formation of Oklahoma, U.S.A.: implications for paleoclimate and paleogeography of the midcontinent. J. Sediment. Res. 83, 825–846. doi: 10.2110/jsr.2013.59
[5] ↑ Zambito, I., James J., and Benison, K. C. 2013. Extremely high temperatures and paleoclimate trends recorded in Permian ephemeral lake halite. Geology 41:587–590. doi: 10.1130/G34078.1
[6] ↑ Soreghan, M. J., Swift, M. M., and Soreghan, G. S. 2018. Provenance of Permian eolian and related strata in the North American midcontinent: tectonic and climatic controls on sediment dispersal in western tropical Pangea. Geol. Soc. Am. Spec. Pap. 28:1–28. doi: 10.1130/2018.2540(28)