Abstract
Often, the first thing that comes to mind when we think about bacteria is that they are harmful and cause diseases. However, this is not entirely true. Almost all bacteria are beneficial to humans, animals, or plants. In this article, we focus specifically on the bacteria that benefit plants. You may be surprised to learn that these bacteria are not just helpful to plants, but essential for plant survival. These beneficial bacteria provide plants with necessary nutrients from the soil, reduce the effects of environmental stresses, and protect plants from harmful bacteria and other enemies. That is why these bacteria are known as plant growth-promoting bacteria. As you read, you will learn about the functions and mechanisms of these essential bacteria.
The Underground Story of Food
There are a huge number of bacteria in the soil. According to some estimates, one spoonful of soil contains ~1 billion bacteria. Bacteria are much older than the plants on Earth today. Long ago, bacteria were mining the rocks for nutrients, to stay alive in early Earth’s harsh conditions. Plants were living in the ocean, in the form of algae. Then, about 450 million years ago, plants made the move to land [1]. It was a very challenging transition, because plants cannot move from one place to another like animals can, but they still need water and various nutrients like nitrogen, phosphorus, zinc, and iron to grow and be healthy.
To reach food and water while remaining anchored in place, plants grew their roots deep down and spread out around them. The nutrients that plants needed were always there, but in locked-up forms they could not use. Fortunately, there are certain soil bacteria called plant growth-promoting bacteria (PGPB) that have specialized skills in mining, transferring, and releasing locked-up nutrients. PGPB crave sugars but never have a fresh supply. The advantage plants had over all other life forms on land was that they could prepare their own fresh sugars, powered by solar energy, through the process of photosynthesis. So, this is how the friendship started between bacteria and plants. PGPB perform critical transformations that release locked-up soil nutrients for plants to use. In return, plants provide PGPB with sugars. It is as if plants are paying PGPB for their services, with their own homemade sweets [2].
Bacteria as Nitrogen Fixers
Nitrogen is very special nutrient for plants because it is one of the main ingredients of their genetic material and the proteins that perform all their functions. These proteins include chlorophyll, which gives plants their green color and acts as a “solar panel” during photosynthesis. Plants cannot use nitrogen gas from the air, where it is ~80% of Earth’s atmosphere. However, PGPB have a special tool, called nitrogenase, that lets them use nitrogen gas. Nitrogenase breaks nitrogen gas (N2) and adds three hydrogen atoms (3H+) to form ammonia (NH3), which then takes another hydrogen atom from atmosphere to form ammonium (). Ammonium is a form of nitrogen that plants can use as food. This whole process is called bacterial nitrogen fixation.
PGPB are so important for plants that some plants have special places for them on their roots, called root nodules. PGPB live in root nodules, where they are treated like VIP guests. In exchange, the PGPB must fix nitrogen (Figure 1). For example, Rhizobium bacteria live inside the root nodules of beans and lentils. However, some bacteria do not want to give up their freedom. For example, Azospirillum bacteria prefer to roam freely around the roots and fix nitrogen nearby [3]. In modern-day agriculture, nitrogen is often supplied to plants through synthetic fertilizers applied to crops. However, most scientists believe that bacterial nitrogen fixation can replace the need for man-made fertilizers. Production of man-made fertilizers uses a lot of energy, and its excessive use can cause water pollution. Further, the production and transportation of fertilizers increase greenhouse gases, which are causing environmental problems. Use of PGPB as biological fertilizers can reduce the energy consumption and environmental problems caused by man-made fertilizers.
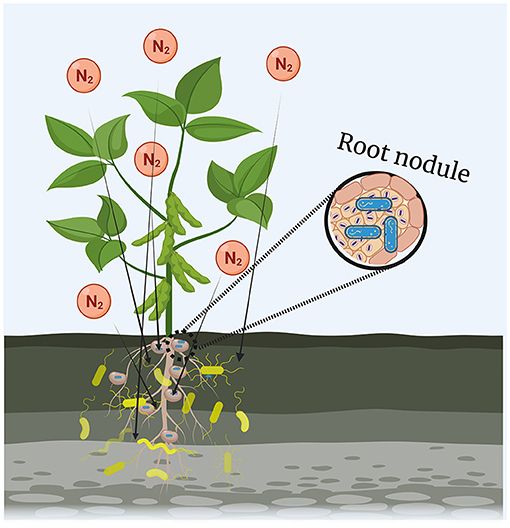
- Figure 1 - Certain PGPB (Rhizobium, blue bacteria) live in the root nodules and fixing atmospheric Nitrogen (N2) for bean and lentil plants (legumes).
Acids to Dissolve and Magnets to Capture
Other nutrients, including phosphorus, zinc, and iron, are also essential to plants. Plant genetic material requires phosphorus, and many plant proteins need zinc and iron to function. Iron is also part of the chlorophyll molecules that perform photosynthesis. These nutrients are unavailable to plants unless PGPB make them available. But how do they do that? Have you ever tasted something sour, like a lemon or vinegar? That sour taste comes from acids! Acids are great at dissolving other substances, like metal or rocks, by breaking them down into tiny pieces. For example, the stomach contains the acids that dissolve the foods we eat, which helps us to absorb nutrients. Similarly, PGPB like Bacillus and Acinetobacter produce various acids to dissolve locked-up nutrients like phosphorus, zinc, and iron in soil (Figure 2), which can then be absorbed by plant roots [4].
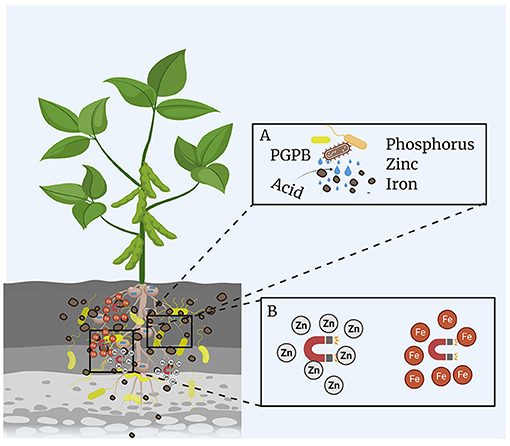
- Figure 2 - PGPB help plants take up nutrients.
- (A) PGPB release various acids to dissolve locked-up nutrients like phosphorus, zinc, and iron in soil. (B) PGPB produce siderophores, which are like small magnets that attract nearby metal nutrients and take them into roots.
In soils, metal nutrients like iron and zinc are relatively rare and, even after they are unlocked, they do not get into plant roots efficiently. In this case, PGPB release small molecules called siderophores, which are like small magnets that attract nearby metal nutrients and take them into roots (Figure 2). Bacillus and Acinetobacter are well-known for producing these small magnets to help their plant friends. These bacteria also create tiny soil clumps that improve the ability of soil to hold water and nutrients and help plant roots to spread. Some cereal crops like wheat, rice, and corn are naturally deficient in iron and zinc, and scientists think that PGPB could be a promising way to boost the amounts of zinc and iron in these foods, helping people to get enough of these nutrients [4].
PGPB—Healers and Peacekeepers
Unlike humans and other animals, plants do not have brains, but they can sense environmental cues such as light, heat, and cold, as well as stresses like lack of nutrients or water. Plants react to cues and stresses through growth or movement. For example, sunflower plants move their flowers to face the sun, and lack of water or nutrients can cause leaves to curl and fall off. How do these messages get to flowers or leaves? The messengers responsible for this communication are molecules called hormones.
Plants have various hormones that carry both positive and negative messages. For example, some hormones play a role in seed germination, root and shoot growth, and fruit ripening, while other hormones cause growth reduction or result in leaves and flowers falling off. PGPB can produce hormones, sending messages to promote plant growth, or counteracting damaging messages to protect plants from damage. For example, Bacillus produce a hormone called auxin, which improves plant growth and overall health [5]. Additionally, these bacteria have a special enzyme called ACC deaminase, which prevents the production a negative messenger that can stop plant growth and cause leaves and flowers to fall off. Thus, PGPB not only provide nutrients but also contribute to the well-being of plants in difficult environments.
Unfortunately, there are also soil organisms (bacteria, fungi, and other small enemies) that can harm plants. But first, these harmful organisms must face PGPB. Similar to the way humans take antibiotics to kill bacteria and use vaccines to boost protection against dangerous invaders, many PGPB produce antibiotics to kill harmful bacteria and other plant enemies (Figure 3). These act as natural vaccines, triggering the plants to protect themselves. PGPB can also starve harmful microbes for iron, by capturing all the iron in the area using their siderophore magnets. When the harmful organisms are dead, PGPB might use them as nutrients for themselves and the plants. Bacillus and Pseudomonas are the best-known examples of bacteria that protect plants from foreign invaders [6]. Therefore, scientists believe that PGPB may be able to replace pesticides, which are synthetic chemicals used to prevent plant diseases. Pesticides also harm beneficial microbes, insects, and the environment.
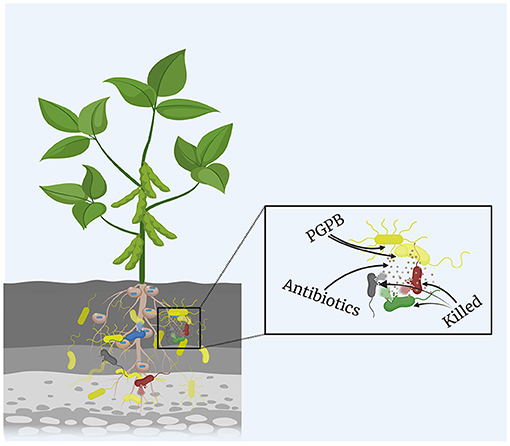
- Figure 3 - Many PBPB can kill harmful microbes by producing antibiotics.
Take-Home Message
Scientists understand the importance of PGPB in helping plants grow and stay healthy. However, since there are millions of types of bacteria in nature, researchers still have lots of questions. One main question involves how plants select the types of bacteria that help them. If researchers grow PGPB in a laboratory and provide them to plants, would the plants accept them as friends and would the community of organisms around the roots welcome them? Another important question is how climate change will affect future crops and their PGPB. Obtaining answers to these questions will help us better understand the relationship between plants and PGPB, and potentially allow us to find ways to use these helpful bacteria for our home gardens and farms.
Glossary
Photosynthesis: ↑ Process by which plants synthesize sugars in their leaves by combining water and carbon dioxide, powered by solar energy.
Nitrogenase: ↑ An enzyme produced by the nitrogen-fixing bacteria to fix nitrogen.
Bacterial Nitrogen Fixation: ↑ A process in which bacteria convert N2 to ammonia (NH4+) using nitrogenase enzyme is called bacterial nitrogen fixation.
Root Nodules: ↑ Small, rounded swellings on the roots of certain plants, containing nitrogen-fixing bacteria that can convert nitrogen gas into a form that plants can use.
Siderophores: ↑ Molecules that can bind tightly to certain metals. Soil bacteria release siderophores to scavenge metals for themselves and their plants and to “starve” competitors or harmful organisms.
Hormones: ↑ Chemical substances produced by cells that function as signaling molecules. They play important roles in the growth, development, and behavior of organisms.
Auxin: ↑ A hormone that improves the plant growth by improving the root growth.
ACC Deaminase: ↑ A protein that blocks a negative growth hormone in plants and thus helps protect plants from damage.
Conflict of Interest
The authors declare that the research was conducted in the absence of any commercial or financial relationships that could be construed as a potential conflict of interest.
References
[1] ↑ Delaux, P. M., and Schornack, S. 2021. Plant evolution driven by interactions with symbiotic and pathogenic microbes. Science 371:eaba6605. doi: 10.1126/science.aba6605
[2] ↑ Singh, B. K., Millard, P., Whiteley, A. S., and Murrell, J. C. 2004. Unravelling rhizosphere–microbial interactions: opportunities and limitations. Trends Microbiol. 12:386–93. doi: 10.1016/j.tim.2004.06.008
[3] ↑ Stokstad, E. 2016. The nitrogen fix. Science 353:1225–7. doi: 10.1126/science.353.6305.1225
[4] ↑ Hakim, S., Naqqash, T., Nawaz, M. S., Laraib, I., Siddique, M. J., Zia, R., et al. 2021. Rhizosphere engineering with plant growth-promoting microorganisms for agriculture and ecological sustainability. Front. Sustain. Food Syst. 5:617157. doi: 10.3389/fsufs.2021.617157
[5] ↑ Lambrecht, M., Okon, Y., Broek, A. V., and Vanderleyden, J. 2000. Indole-3-acetic acid: a reciprocal signalling molecule in bacteria–plant interactions. Trends Microbiol. 8:298–300. doi: 10.1016/S0966-842X(00)01732-7
[6] ↑ Pennisi, E. 2021. This microscopic hitchhiker is a friend to both plants and humans. Sci. Mag. 2021:abi6461. doi: 10.1126/science.abi6461