Abstract
A solar eclipse is a unique and exciting event that involves the Earth, moon, and Sun. During a solar eclipse, the moon covers the Sun entirely or partially. As a result, the moon temporarily blocks the sunlight coming toward Earth. Solar eclipses also change Earth’s atmosphere by affecting how close tiny particles called electrons are to each other. These electron density changes can be measured over various regions of the Earth by a group of satellites called the Global Navigation Satellite Systems (GNSS). In this article, we will tell you about changes in electron density seen during two recent solar eclipses. This is important because these changes can affect satellite-based navigation (like the GPS on your phone) and communication.
What Happens During a Solar Eclipse?
From kids to adults, many people become excited when they learn that a solar eclipse will be visible from their city or town (Figure 1). During a solar eclipse, everyone becomes an astronomer for a few minutes. Solar eclipses are also exciting for scientists who study Earth’s atmosphere because changes happen to particles in the upper atmosphere during an eclipse. Before diving into that topic, we must first explain exactly what happens during a solar eclipse.
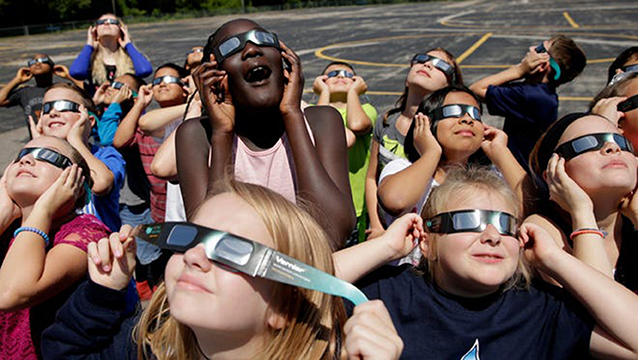
- Figure 1 - Children at Clardy Elementary School, in Kansas City, Missouri, watch a solar eclipse on August 21, 2017.
- The students were practicing the proper use of eclipse glasses, which protect their eyes from the Sun’s rays (Figure credit: https://www.usatoday.com/story/news/nation/2017/08/20/solar-eclipse-2017-anticipation-builds-millions-pack-prime-viewing-spots/584083001/).
Every object in the solar system creates a shadow when it blocks the path of incoming sunlight. A solar eclipse on Earth occurs when our natural satellite, the moon, passes between the Sun and the Earth, eventually hiding the Sun from our view. While the diameter of the sun is about 400 times larger than that of the moon, the Sun is also about 400 times farther from the Earth than the moon. So, looking at the Sun and moon from Earth, they appear nearly the same size. When the moon blocks the Sun perfectly, only a dim outer ring of the sun appears to be visible. This spectacular sight is called a total solar eclipse. There are other kinds of eclipses, too. A partial solar eclipse occurs when the moon blocks only a portion of the Sun. An annular solar eclipse is like a total solar eclipse, but it happens when the moon is near its furthest point from Earth, and therefore appears smaller. In an annular eclipse, a fiery, glowing ring appears around the edges of the moon. Figure 2A shows the difference between the various kinds of solar eclipses.
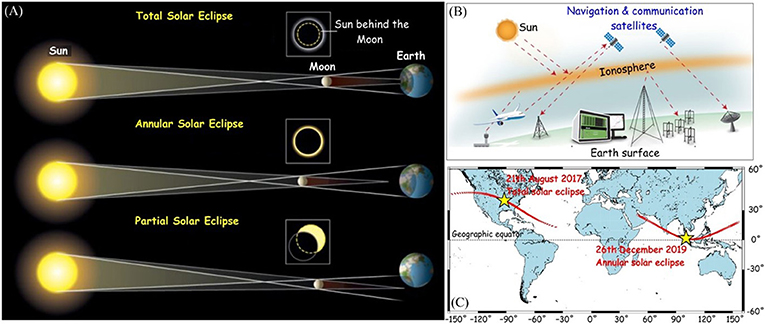
- Figure 2 - (A) There are three main types of solar eclipses.
- When the moon comes between the Sun and Earth, it may completely cover the Sun (total solar eclipse) or partially cover the Sun (annular and partial solar eclipse) (https://www.clearias.com/eclipse/). (B) Signals for navigation and long-distance communication must pass through a layer of the atmosphere called the ionosphere. (C) Location and path of the solar eclipses (red curves) on August 21, 2017 (total solar eclipse) and December 26, 2019 (annular solar eclipse). The points of the greatest eclipse are marked by the yellow stars.
Solar Eclipses Change Earth’s Atmosphere
In addition to being spectacular, solar eclipses can teach us some exciting things about the ionosphere. The ionosphere is a layer of the upper atmosphere, located about 80–600 km above Earth’s surface [1]. The atoms and molecules in the ionosphere normally break into charged particles called ions when they absorb energy from the Sun. Ions in the ionosphere can be positive, negative, or neutral in charge. The ionosphere also contains many free electrons, which affect radio waves and satellite signals. In other words, the ionosphere is an important part of the atmosphere because it reflects and changes the radio waves and satellite signals that are used for navigation and long-distance communication (Figure 2B). Without the ionosphere, forms of navigation like GPS and long-distance satellite communication would not be possible.
Since the energy of sunlight is what creates the ions and electrons in the ionosphere, you might predict that the sudden absence of sunlight during a solar eclipse might cause changes in the ionosphere—and you would be correct! During a solar eclipse, when the moon temporarily blocks the incoming sunlight from reaching the Earth, the density of electrons in the shadow zone within the ionosphere drops.
Studying the Ionosphere and the Effects of Solar Eclipses
How can scientists detect and measure changes in the ionosphere? One way involves using satellites, particularly the Global Navigation Satellite Systems (GNSS) network. This is a group of satellites that provides location, navigation, and timing services across the world (Figure 2B). GNSS satellites are continuously moving around the Earth, and they enable scientists to capture the changes in the ionosphere that happen during a solar eclipse [2].
Figure 2C shows the paths of two recent solar eclipses. One was a total solar eclipse that traveled through the central United States on August 21, 2017. The other one was an annular solar eclipse that occurred in Southeast Asia on December 26, 2019. When both eclipses were their fullest, a dense GNSS network captured a significant drop in electron density in the shadow zone of the ionosphere [3, 4]. The drop in electron density happens because the incoming sunlight is temporarily blocked from reaching the Earth, which stops the breakdown of atoms and molecules in the ionosphere into ions, resulting in a drop in electron density at the time of the solar eclipse. This is important because it influences radio signals as they travel to distant places on Earth, and it also affects GNSS signals that travel through this layer of the atmosphere.
Things Get Even More Interesting in the Ionosphere
Scientists found it quite interesting when they discovered the decrease of electrons in the ionosphere during a solar eclipse. But the GNSS satellites moving across the sky also found something even more interesting. Slight increase in electron density were also seen in the ionosphere during the two solar eclipses described above. The locations where electrons increased can be seen in Figure 3. Why did these areas experience increases in electron density during the eclipses? Scientists have suggested that this “excitement” in the ionosphere is mainly caused by the interaction between the ionosphere and Earth’s magnetic field [5].
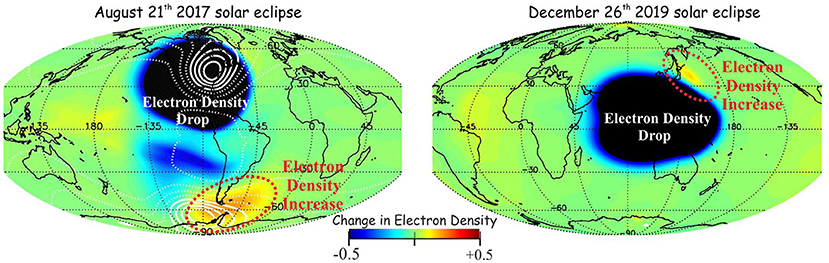
- Figure 3 - Variation in the electron density in the ionosphere during two recent solar eclipses.
- The dark blue represents a drop in electron density at the location of the greatest eclipse. The yellowish-colored patches circled in red at the tip of South America and Antarctica (for the August 21, 2017 eclipse) and around northern Japan (for the December 26, 2019 eclipse) are the areas where the electron density increased.
The Excitement Continues
In this article, you have learned about solar eclipses and their effects on the ionosphere. During a solar eclipse, there is a significant drop in the electron density in the ionosphere, but in some areas, the electron density mysteriously increases slightly. These changes in the ionosphere affect radio waves and the GNSS signals that travel through this layer of the atmosphere. These findings are exciting and could be important for scientists trying to understand the features of the ionosphere and how they affect communication, navigation, and information transfer during a solar eclipse. There is much more work to be done and many important questions still to answer.
Glossary
Total Solar Eclipse: ↑ When the moon passes between the Sun and Earth, completely blocking the face of the Sun.
Partial Solar Eclipse: ↑ When the moon passes between the Sun and Earth, partial blocking the face of the Sun.
Annular Solar Eclipse: ↑ When the moon is its furthest away from Earth, so it does not completely cover the Sun during the eclipse. As a result, only a ring of light is visible.
Ionosphere: ↑ The layer of the atmosphere that extends from 80 to 600 km above Earth’s surface. It contains a high concentration of ions and free electrons, and it is important for communication and navigation.
Ions: ↑ Electrically charged (either positive or negative) atoms or molecules.
Electrons: ↑ Small particles of an atoms with a negative charge. Electrons are the primary carrier of electricity in solids.
Shadow Zone: ↑ Places on Earth where the sun’s light can’t reach temporarily during the solar eclipse.
Global Navigation Satellite Systems (GNSS): ↑ A group satellites that provides positioning, navigation, and timing services on a global or regional scale. For example, the GPS your phone uses is a group of 24 satellites.
Electron Density: ↑ The number of electrons present in a square meter area of the ionosphere.
Conflict of Interest
The authors declare that the research was conducted in the absence of any commercial or financial relationships that could be construed as a potential conflict of interest.
References
[1] ↑ Strahler, A. N. 1963. The earth sciences. Science 142:1287–8. doi: 10.1126/science.142.3597.1287-a
[2] ↑ Heki, K. 2021. Ionospheric disturbances related to earthquakes. Ionosphere Dyn. Appl. 511–26. doi: 10.1002/9781119815617.ch21
[3] ↑ Kundu, B., Panda, D., Gahalaut, V. K., and Catherine, J. K. 2018. The August 21, 2017 American total solar eclipse through the eyes of GPS. Geophys. J. Int. 214:651–5. doi: 10.1093/gji/ggy149
[4] ↑ Senapati, B., Huba, J. D., Kundu, B., Gahalaut, V. K., Panda, D., Mondal, S. K., et al. 2020. Change in total electron content during the 26 December 2019 solar eclipse: constraints from GNSS observations and comparison with SAMI3 model results. J. Geophys. Res. Space Phys. 125:e2020JA028230. doi: 10.1029/2020JA028230
[5] ↑ Huba, J. D., and Drob, D. 2017. SAMI3 prediction of the impact of the 21 August 2017 total solar eclipse on the ionosphere/plasmasphere system. Geophys. Res. Lett. 44, 5928–35. doi: 10.1002/2017GL073549