Abstract
Nature is absolutely amazing at building tiny machines. These machines are responsible for many of the important processes in biology. For example, our eyes have millions of tiny switches that enable us to see each other, and our cells contain tiny motors that pump materials in and out and produce energy. In my lab in the Netherlands, my students and I are excited about building tiny molecular machines inspired by nature. We build molecular switches and motors, and recently we even built the tiniest car in the world! These molecular machines can be used for improving human health, advancing technology, and creating new products that never existed before. In this article, I will tell you how we build our molecular machines and give you a peek into how we could use them to improve lives.
Professor Ben L. Feringa won the Nobel Prize in Chemistry in 2016, jointly with Profs. Jean-Pierre Sauvage and J. Fraser Stoddart, for the design and synthesis of molecular machines.
Tiny Machines—in Nature and in the Lab
Did you know that, in every moment, countless molecular machines are operating in your body? These machines are responsible for your ability to move, to see, and to produce the energy your cells need to function. In your eyes, for example, there are millions of tiny molecular switches that respond to light (Figure 1). When light hits these tiny switches, they turn on and send an electrical signal to the brain, enabling us to see each other and the world around us.
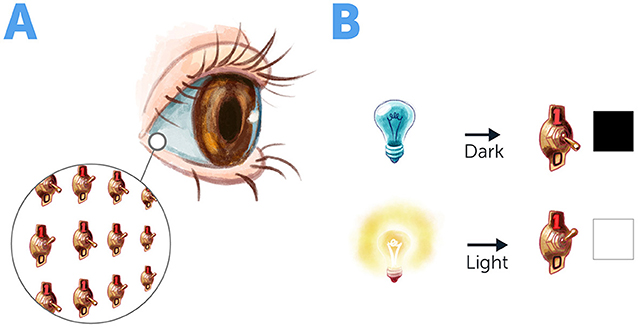
- Figure 1 - Molecular switches in the eye.
- (A) At the back of the eye, there is region filled with tiny molecular switches that respond to light. (B) When there is no light, these switches are “off” and they do not transfer any signal to the brain, so we do not see anything. When light hits these switches, they turn “on” and transfer signals to the brain, enabling us to see each other and the world around us.
Since I was a little boy, I have always been inspired by the beauty of Mother Nature. I enjoyed observing nature, learning from it, and using this knowledge to make new, helpful things. While I was in university, I was lucky enough to create my first molecule in the laboratory! Knowing that I created a molecule that never existed before was such an exciting feeling. I became interested in using my knowledge of chemistry to create synthetic molecules that mimic the biological machines created by nature. This took me on a long journey of exploration, eventually leading to the assembly of the tiniest car ever built.
Building Molecules in the Lab
In my lab in the Netherlands, we build tiny molecules and study their properties. The size of these molecules is difficult to imagine. If you bring two fingers as close together as possible in front of your eyes, until you see only a tiny sliver of light between them, that is the size of 1 millimeter. The molecules we build are only nanometers (nm) in size, and 1 nm is 1 million times smaller than the gap between your fingers! Building molecules is a bit like making a beautiful castle with Lego® blocks. We take the smallest molecules, perform chemical reactions to remove or add certain parts to them, and then use other reactions to put the molecules together to build bigger molecules. In essence, we are breaking and creating bonds between the atoms that make up molecules. By “playing” with these molecular building blocks, we can make new molecules that never existed before.
We often want to make molecules for specific uses, such as beautiful colors for painting cars or effective medicines for treating diseases. We commonly use computers to help us figure out which atoms and reactions we should use to design new molecules. Even with the help of computers, it is still very difficult to predict the properties of the new molecules we make, and these molecules often do not have the exact properties we want. Sometimes this “error” is actually a good thing because it helps us discover properties we had not thought of. Other times, we must keep changing the molecular ingredients until we eventually create the desired properties.
After making new molecules, we measure them and learn all about how they behave, both as single molecules and when there are many of them together (think of this like studying a spoonful of sugar molecules as well as one individual sugar molecule). We have advanced laboratory equipment to help us study the structure and behavior of molecules, including a very powerful microscope called a scanning tunneling microscope (STM). The STM contains a type of a needle with a tiny tip the size of only one atom. Can you imagine that? Using the STM and other advanced equipment, we can determine if our molecules have the three-dimensional shapes we were hoping for, as well as the desired properties (e.g., the right color, stiffness, or stickiness). Sometimes it takes us many tries, but we can eventually create the right molecules with the right properties.
Molecules that Move—From Switches to Motors to Cars
In my lab, we are particularly interested in molecules that can move, like the molecular machines in the body. We love movement so much that we are even trying to make moving versions of materials that usually do not move—like plastic and glass. How cool would it be if the glass in your bedroom’s window or your family’s car could clean itself? A simple kind of molecular movement involves switching between two states, like the molecular switches in the eyes. To create that kind of movement, we designed molecules with an upper part that can be “flipped” using light [1]. Think of this like switching between a “right-handed” and a “left-handed” state (Figure 2). One color of light switches the “right-handed” state over to the “left-handed” state, and another color of light switches the “left-handed” state back to the “right-handed” state. This type of molecule can be used, for example, for storing digital information in computers (as you may know, information is stored in computers in basic units represented by the numbers “1” and “0”. Usually, the 1s and 0s are implemented as different voltages in a component made of silicon that is called a transistor. The same idea of having two distinct “1” and “0” states could be implemented using molecular switches that have two states).
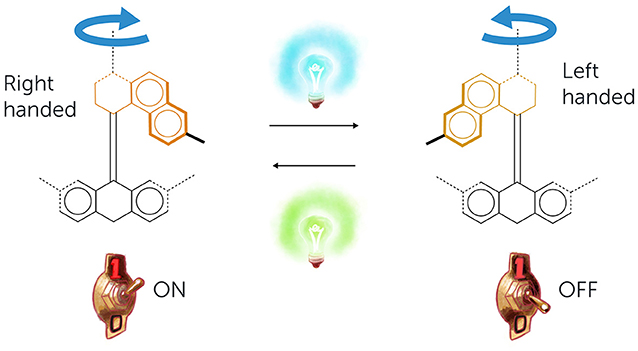
- Figure 2 - Synthetic molecular switches.
- In our lab, we designed molecules that act like switches. Using light, we can switch these molecules from a right-handed to a left-handed state and back again. Such molecules could be useful for various technologies, including storing digital information in computers.
Once we could build molecular switches that move between two states, we wanted to advance to a more complicated type of movement—that of a rotary motor. A rotary motor is a machine that takes in some kind of fuel and rotates in one direction to produce a desired product. In our cells, we have tiny rotary motors that create ATP—the energy molecule that fuels cells. Inspired by this natural rotary motor, we tried to create a synthetic rotary motor that takes in light (either from a lamp or from the sun), and continuously rotates in one direction, like the tire of a moving car. This task was challenging because it is very hard to control the direction that molecules move—they naturally tend to move like crazy in all directions. It was therefore a challenge to make the movement continuous and smooth.
To achieve this type of motion, we had to build the three-dimensional structure of the molecules very precisely. Like the motor in a car, our molecular motor had to include an axle and a rotating part that rotates around the axle. After much trial and error, we managed to build a molecule that rotates by 360° in four steps of 90° each [2] (see this video). The first and third steps require light, which breaks a certain bond in the molecule that enables the rotating part to rotate around the axle. The second and fourth steps happen spontaneously without light, when the molecule “relaxes” from a state of high energy to a state of a lower energy.
Our original rotary motor molecule was slightly less than 1 nm in size and it rotated once every hour. By changing its shape and bond properties in various ways, we eventually achieved speeds up to 10 million rotations every second! While playing around with these molecular motors, my students raised a challenging question—could we transform their rotational movement into forward movement, like in a car?
I got excited by this idea and told my students that we should build a four-wheel drive [3]. Four molecular motors would serve as our four wheels, and we had to figure out how to connect them to the car’s framework (called the chassis). To do so, we first built half the chassis and connected two wheels to it, then we built the second half of the chassis and connected two wheels to that half. Finally, we connected the two halves to get the complete car. Importantly, we had to make sure that our “wheels” rotated in the correct direction. Eventually, through all this hard work, we created the smallest nano-car in the world (see this video)!
After cracking this challenge, we created an “assembly line” for nano-cars, which we could use build nano-cars with various sizes and that drive at various speeds.
Self-Cleaning Cars and Smart Drugs
Now that we have molecular switches, motors, and cars, what can we do with them? In general, molecular machines enable us to design materials that can change their structure and function in response to certain triggers [4]. For example, self-cleaning or self-repairing materials could, through their internal movements, remove dust from themselves or repair cracks or other damage. Imagine these materials being used to build a car that would never have to be washed and would autonomously repair scratches and scrapes on its doors! The same capabilities could be very useful in other situations, such as self-cleaning solar panels or self-repairing smartphone screens, for example.
We can also use responsive materials to improve medicines and create what are called smart drugs [5]. Opposed to normal drugs, which are distributed throughout the body and act everywhere, smart drugs can be activated only when and where we want them to act. Molecular switches could be attached to the drugs, so that we could switch them on only when we shine light on them. Using this method, we could prevent undesired effects of drugs in the body and in the environment. For example, antibiotics with this property could help to prevent antibiotic resistance, which is a serious issue. We can also envision tiny molecular cars that roam around inside the body and help keep it healthy, for example by delivering specific medicines to the specific areas where they are needed (Figure 3).
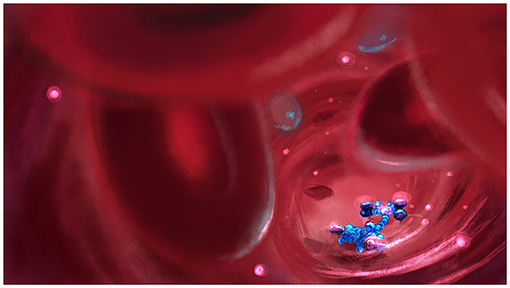
- Figure 3 - Can we use tiny molecular cars to improve our health?
- In the future, we might have tiny molecular cars traveling throughout our bodies, helping us maintain good health. For example, these cars might release drugs in the specific locations in the body where those medicines are needed.
When the Wright brothers performed their first historical flight in 1903, I do not think anyone predicted that, just a 100 years later, millions of people would be flying all over the globe. Even though the planes we use today are much more sophisticated than the first airplane invented by the Wright brothers, planes are still built on the same principles. I envision the same for the field of molecular machines—in the future, our early ideas will be developed and used for many technologies that we currently cannot even dream about. Part of our job as chemists is to be creative, build our own molecular world, and invent things that have never existed before. The process of discovery is often challenging because we are walking through unknown territory. With time, I have learned to stay confident even when my scientific progress is tough, knowing that the struggle could lead to something spectacular. I know that every one of you also has fantastic talents, and I hope that you will enjoy them. Follow your dreams and have faith in your ability to fulfill them.
Glossary
Molecular Switches: ↑ Tiny molecules that switch between states in response to a stimulus. The simplest switches have two states, “on” and “off”, much like light switches in your home.
Synthetic: ↑ Made by humans (not by nature), often in a laboratory.
Scanning Tunneling Microscope (STM): ↑ A microscope containing a tiny tip that scans molecules and helps us study their structure and behavior.
Rotary Motor: ↑ A rotating machine that creates mechanical movement. Rotary motors are commonly used in vehicles, including cars and airplanes.
Conflict of Interest
The authors declare that the research was conducted in the absence of any commercial or financial relationships that could be construed as a potential conflict of interest.
Acknowledgments
I wish to thank Or Raphael for conducting the interview which served as the basis for this paper and for co-authoring the paper, and Alex Bernstein for providing the figures. The interview was conducted with the assistance of the Israel Academy of Sciences and Humanities, who brought BF to Israel, and of Dr. Yael Ben Haim, Manager of the Sciences Division in the Academy.
Additional Materials
- Ben Feringa: Nano-motors that open giga-opportunities (YouTube).
- Ben Feringa Reserach Group (University of Groningen).
References
[1] ↑ Feringa, B. L., Van Delden, R. A., Koumura, N., and Geertsema, E. M. 2000. Chiroptical molecular switches. Chem. Rev. 100:1789–816. doi: 10.1021/cr9900228
[2] ↑ Koumura, N., Zijlstra, R. W., van Delden, R. A., Harada, N., and Feringa, B. L. 1999. Light-driven monodirectional molecular rotor. Nature 401:152–5. doi: 10.1038/43646
[3] ↑ Kudernac, T., Ruangsupapichat, N., Parschau, M., Maciá, B., Katsonis, N., Harutyunyan, S. R., et al. 2011. Electrically driven directional motion of a four-wheeled molecule on a metal surface. Nature 479:208–11. doi: 10.1038/nature10587
[4] ↑ Feringa, B. L. 2020. Vision statement: materials in motion. Adv. Mater. 32:1906416. doi: 10.1002/adma.201906416
[5] ↑ Wegener, M., Hansen, M. J., Driessen, A. J., Szymanski, W., and Feringa, B. L. 2017. Photocontrol of antibacterial activity: shifting from UV to red light activation. J. Am. Chem. Soc. 139:17979–86. doi: 10.1021/jacs.7b09281