Abstract
Recently, the field of bioengineering, which uses biomedical knowledge to solve problems and create products, has made great progress creating tiny, functioning models of human organs, called organoids. The brain is the most complex organ of the human body. Although brain organoids have been created, they still cannot perform calculations, learn, memorize, or make decisions—“thinking” functions only seen in humans and animals. But a new field of science is emerging that could create brain organoids with some cognitive functions. The necessary technologies are now available, and scientists are starting to combine them. Thinking brain-cell cultures create a lot of ethical questions that must be addressed as research proceeds. This article describes the technologies that form the basis of the science called organoid intelligence (OI). In the future, OI could help us study brain functions, understand brain diseases, find new cures, and could possibly even lead to new supercomputers that are more brain-like than today’s computers.
Brains vs. Computers?
Do you think the human brain is more powerful or less powerful than a computer? If you have heard about computer programs beating human opponents at complex strategy games like chess or Go, you might naturally think that computers are “smarter.” Computers can certainly process simple information faster than human brains can, and artificial intelligence (AI) is increasingly being incorporated into many common technologies, from facial recognition on phones to streaming video recommendations. AI is the ability of a computer to think and learn, with the aim of performing tasks usually done by people. It is easy to believe that AI will eventually surpass human abilities, even at complex tasks like driving. But there is more to the story! The processing power and storage capacity of the human brain are immense: in 2013, it took the world’s fourth-largest computer 40 min to model 1 s of 1% of a human’s brain activity! The memory of the human brain is about as big as the large computers found at universities or research institutes; the number of calculations per second that can be performed by the human brain was only reached by the fastest supercomputer in the world this year (2022). Some tasks may never be matched by AI—for instance, a child can distinguish dogs and cats after seeing about 10 pictures, while AI needs more than 1,000.
As an example, let us look at the AlphaGo system—the AI that beat the world champion in Go in 2016. AlphaGo was trained on data from 160,000 games [1]. If a human played for 5 h a day every single day, it would take that person over 175 years to experience the same number of training games! This tells us that the brain is much more efficient than AI at learning how to perform complex activities. What is more, training AI requires a lot of energy—much more energy than the human brain uses to learn. If it takes the same amount of time for a human and an AI system to learn a new task, the AI system requires 10 million times more energy! AlphaGo’s 4-week training required more energy than it takes to sustain an active adult human for 10 years.
These comparisons tell us that it will be difficult for AI to ever surpass humans at complex tasks like driving, which require real-time learning in a changing environment. This is especially true if technology companies keep their promises to limit global warming by decreasing their carbon emissions. In 2017, it took the equivalent of 34 coal-powered plants to meet the power needs of U.S. data-storage centers [2]! But what if, instead of trying to make computers more like human brains, we instead tried to make human brains more computer-like? And what if these computer-like human brains could be grown in a lab and interfaced with actual computers? Does this sound like something out of a science fiction movie? Although it is still in the very early stages with lots of challenges ahead, this futuristic-sounding technology, called organoid intelligence (OI), is closer to becoming a reality. As a method of biological computing, OI may be able to overcome the limitations of traditional AI and accomplish tasks that were previously unimaginable for machines.
What Are Brain Organoids?
The eventual success of OI depends on our ability to grow tiny, 3D brain cell cultures, called brain organoids (Figure 1A). These tiny organoids, which are generated from stem cells, are 300–500 micrometers (μm; 1/1,000 of a millimeter) in diameter and made up of 30,000–50,000 cells, making them about one 3-millionth the size of the human brain (Figure 1B) [3].
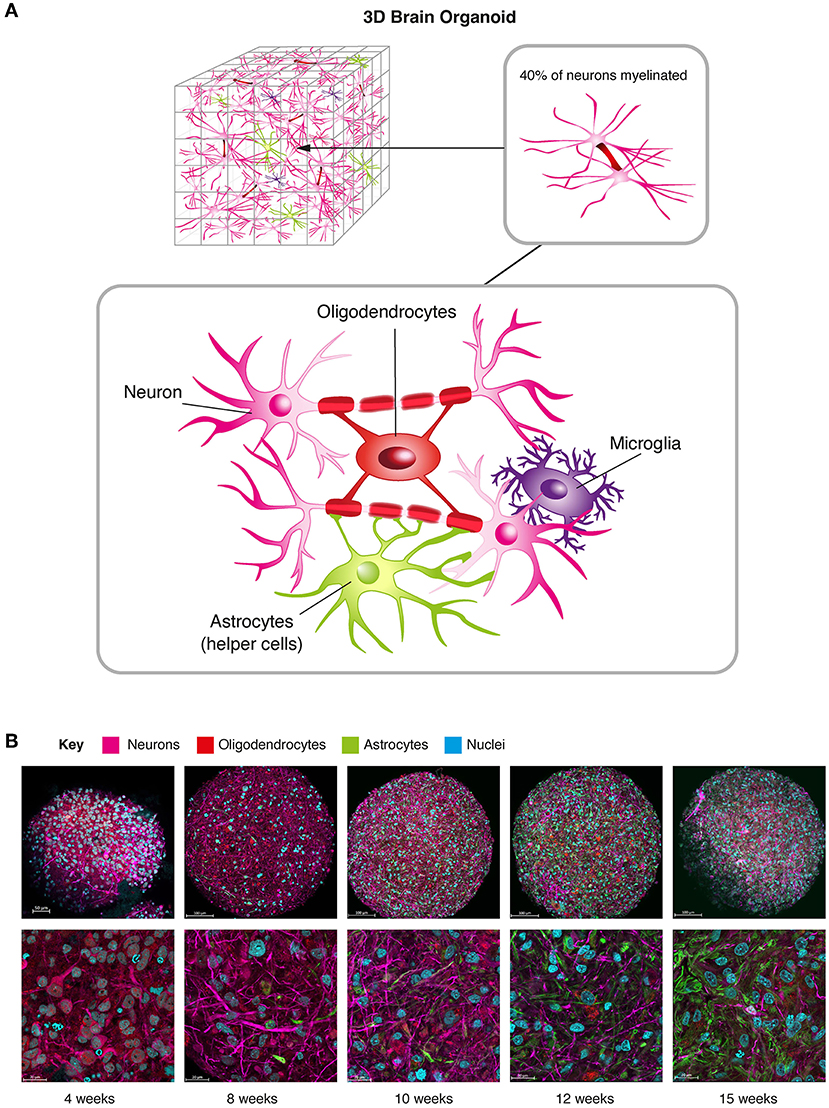
- Figure 1 - (A) 3D brain cell cultures called brain organoids can contain the same brain cell types found in the human brain, including microglia and astrocytes, which support neuron health, and oligodendrocytes, which produce myelin.
- About 40% of neurons in brain organoids are covered with myelin, which can help signals travel between neurons. Fifty-percent of neurons in the human brain are myelinated, so brain organoids are not far off! (B) 3D brain organoids are only about one 3-millionth the size of the human brain (top row: 20x; bottom row: 63x). Neurons appear as early as 4 weeks of culture, while glial cells emerge at 8 weeks, and astrocyte numbers increase over time.
The more closely brain organoids resemble human brains, the more likely they are to be able to perform human-like brain activities, like learning and remembering. Despite their small size, brain organoids have some structural and functional similarities to human brains. For example, the human brain contains several types of cells: neurons (nerve cells), which are responsible for sending signals within the brain and between the brain and the body; oligodendrocytes (oh⋅luh⋅go⋅den⋅druh⋅sites), which cover parts of neurons with a material called myelin; and microglia and astrocytes, which support the health of neurons. Brain organoid cultures have been shown to support the growth of neurons and these other cell types, all of which are necessary for learning (Figure 1A). Additionally, about 40% of the neurons in brain organoids are covered with myelin while, in the healthy human brain, about 50% of neurons are myelinated—so brain organoids are not far off! Myelin acts like the plastic on the outside of a copper wire—it insulates the wire and, different from the insulation on a wire, also makes the signal travel 100 times faster. In terms of function, brain organoids show electrical activity and respond to electrical stimulation in ways that are similar to cells in the brains of premature human babies.
Since the brain organoids being grown now are so tiny that they are barely visible (about the size of a housefly’s eye), learning how to make them bigger and more brain-like is an important first step toward developing organoid intelligence. When brain cell cultures are small or two-dimensional (flat), the cells can obtain oxygen and nutrients and get rid of waste products directly through the fluid they are growing in via diffusion, which is the natural movement of molecules from areas of high concentration to areas of low concentration. But diffusion is not efficient enough to support larger, 3D cultures [4]. The human brain has blood vessels that perform this transport function—half of the brain’s weight in blood flows through the brain every minute. Something similar will be needed to grow larger brain organoids, to prevent the death of their centers—a process called necrosis. Scientists are developing microfluidic systems that can act like tiny blood vessels (Figure 2). Microfluidic systems will not only remove wastes and provide oxygen and nutrients to the brain organoids, but they will also allow scientists to administer and test the effects of other chemicals that are involved in the normal functioning of the human brain, or possible drugs to treat brain diseases.
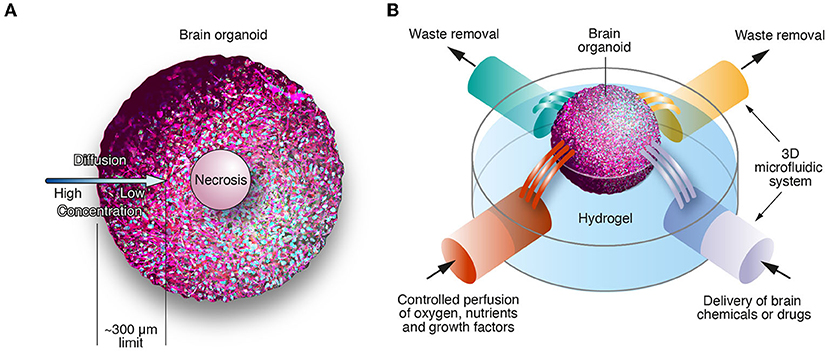
- Figure 2 - (A) Once a 3D brain organoid gets too large, diffusion no longer works to bring oxygen and nutrients into the organoid or remove wastes, because diffusion can only reach about 300 μm into the organoid.
- Thus, the center of the organoid (light pink circle) will die by a process called necrosis. Blood vessels perform this critical function in the human brain. (B) To mimic blood vessels, microfluidic systems have been developed to bring oxygen and nutrients into the brain organoid and remove wastes. These systems permit the growth of larger brain organoids and allow scientists to test the effects of various chemicals and drugs on the organoid’s function.
Measuring Brain Organoid Function and Learning Ability
To develop OI, brain organoids must function similarly to human brains—and scientists must have a way to measure this activity. Doctors measure the electrical activity of actual human brains with a technique called electroencephalography (uh⋅lek⋅trow⋅uhn⋅seh⋅fuh⋅laa⋅gruh⋅fee; EEG), in which electrodes are placed on a patient’s scalp. This technique inspired us to create a tiny version, called a 3D microelectrode array (MEA), that can both stimulate and measure the electrical activity of brain organoids. The MEAs are like flexible shells that fold around the brain organoids, allowing measurement of electrical activity across the entire surface of the 3D organoid (Figure 3). Researchers are also working to develop special probes that the brain organoids can grow completely around, which might provide clearer signals and allow researchers to access the inside of an organoid.
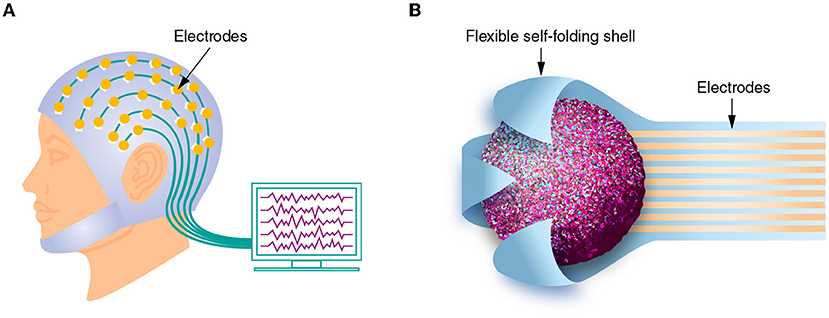
- Figure 3 - (A) Doctors measure the electrical activity of human brains with a technique called electroencephalography (EEG), in which electrodes (yellow circles connected by green wires) are placed on a patient’s scalp.
- (B) EEG inspired scientists to create a tiny version, called a 3D microelectrode array (MEA), that can both stimulate and measure the electrical activity of brain organoids. The MEAs contain many electrodes in flexible shells that the brain organoids are grown inside, allowing measurement of electrical activity across the entire surface of the 3D organoid.
After scientists can monitor the function of brain organoids, they can begin to study whether these organoids can learn. In brain organoids, “learning” will involve responding in a certain way to patterns of electrical or chemical stimulation, for example by changes in the connections between cells or changes in electrical activity. These are the same two factors that affect memory formation and learning in the human brain. One of the first learning tasks could be a simple computer game like Pong, in which a paddle must be moved to keep the bouncing ball in the field. In fact, researchers from Cortical Labs in Australia have recently shown that brain cultures can learn to do this [5].
Beyond Biological Computing
While we have established that brain organoids could lead to a revolution in biological computing, they could also be used as a powerful research tool. Because the brain is so complex and is well-protected by the skull, it is a difficult organ to study in living organisms. So, brain cell cultures that look and act like human brains could help scientists answer important questions that are tough to study in humans—questions about brain development, learning, memory, and the effects of drugs or infections on brain function. Brain organoids could also be used to study devastating brain diseases like dementia. Dementia is a disease of older adults, in which memory and other brain functions progressively decline. Dementia is a growing problem: globally, over 55 million people are living with dementia, and this number is projected to exceed 150 million by 2050 [6]. Alzheimer’s disease, a type of dementia, is among the top 10 causes of death in the US. There is currently no cure for dementia and the treatments that exist are not very effective. A brain organoid system might help researchers to understand what causes dementia and how it affects the brain. Potential treatments could be developed from this knowledge, and they could be tested in brain organoids to make sure they work, before they are used in humans.
Ethical Challenges
While the use of OI for biological computing and critical brain research may sound promising and exciting, OI also generates a number of important questions that scientists and society have never before had to consider. For example, will brain organoids be “conscious,” and if so, is it ethical for scientists to create them? Could organoids experience pain and possibly suffer during the experiments scientists perform on them? Other difficult ethics questions are sure to arise as the field of organoid intelligence develops. Professional ethicists must work closely with researchers to identify and navigate such issues. To be socially responsible, it is also important for OI research to be guided by input from the public. Discussions with members of the public who hold various beliefs and moral perspectives can help to earn the public’s trust, which helps to prevent adverse public reactions to new technologies and maximizes their future impact.
Conclusion
While it may still sound a little like science fiction, we hope that this article has helped you to understand the potential for brain organoids to revolutionize biological computing. There is still much research to be done and many technological and ethical obstacles to face. But, if we succeed, OI is likely to overcome many of the limitations of traditional computing and AI. Specifically, we believe that OI-based biocomputing systems will allow faster decision-making, improved learning, and greater energy efficiency. These advances could impact the entire world, through the development of technologies that could enhance AI performance or create “smart” prosthetics that could help amputees regain body functions. Further, OI presents an amazing opportunity for brain research and could help scientists to discover the basic principles behind thinking, learning, and memory, and to better understand and potentially treat devastating brain diseases like dementia. So, the next time you hear someone talking about AI, take a minute to tell them about OI… and make sure to mention that it is science, not science fiction!
Glossary
Organoid Intelligence: ↑ The ability of an organoid to compute and store information that is provided (input) to execute a task (output).
Biological Computing: ↑ A technique that uses living organisms to perform computer functions, like storing and processing information, to possibly complement current computers.
Brain Organoids: ↑ Bioengineered models of the brain that can perform some brain functions.
Neuron: ↑ A nerve cell; a fundamental unit of the nervous system that uses electrical and chemical signals to transmit information to other neurons an muscle cells.
Myelin: ↑ A fatty, insulating material that covers nerve fibers and makes electrical communication between neurons more efficient.
Microfluidic System: ↑ A technology that circulates fluids through small channels, to supply cells with oxygen and nutrients, for example.
3D Microelectrode Array: ↑ A device with many sensors that can read the electrical activity in brain cell cultures, similar to the electroencephalogram (EEG) that is used in humans.
Ethics: ↑ A branch of philosophy that studies the differences between good and bad or right and wrong.
Conflict of Interest
TH is named inventor on a patent by Johns Hopkins University on the production of brain organoids, which is licensed to AxoSim, New Orleans, LA, USA, and receives royalty shares. TH and LS consult AxoSim.
The remaining author declares that the research was conducted in the absence of any commercial or financial relationships that could be construed as a potential conflict of interest.
Acknowledgments
Co-written by Susan Debad Ph.D., graduate of the University of Massachusetts Graduate School of Biomedical Sciences (USA) and scientific writer/editor at SJD Consulting, LLC. The financial support by Johns Hopkins’ Discovery Grant and SURPASS program is gratefully appreciated. IM received stipends from the NIEHS training grant (T32 ES007141) and the Johns Hopkins’ ASPIRE program, i.e., the Academic Success via Postdoctoral Independence in Research and Education (ASPIRE), an NIH-sponsored Institutional Research and Academic Career Development Award (IRACDA) postdoctoral fellowship.
Original Source Article
↑Smirnova, L., Caffo, B. S., Gracias, D. H., Huang, Q., Morales Pantoja, I. E., Tang, B., et al. 2023. Organoid intelligence (OI): the new frontier in biocomputing and intelligence-in-a-dish. Front. Sci. 1:1017235. doi: 10.3389/fsci.2023.1017235
References
[1] ↑ Kim, J., Ricci, M., and Serre, T. 2018. Not-So-CLEVR: Learning same–different relations strains feedforward neural networks. Interface Focus 8:20180011. doi: 10.1098/rsfs.2018.0011
[2] ↑ Masanet, E., Shehabi, A., Lei, N., Smith, S., and Koomey, J. 2020. Recalibrating global data center energy-use estimates. Science 367:984–6. doi: 10.1126/science.aba3758
[3] ↑ Pamies, D., Barreras, P., Block, K., Makri, G., Kumar, A., Wiersma, D., et al. 2017. A human brain microphysiological system derived from iPSC to study central nervous system toxicity and disease. ALTEX 34:362–76. doi: 10.14573/altex.1609122
[4] ↑ Zhang, S., Wan, Z., and Kamm, R. D. 2021. Vascularized organoids on a chip: Strategies for engineering organoids with functional vasculature. Lab Chip. 21:473–88. doi: 10.1039/D0LC01186J
[5] ↑ Kagan, B. J., Kitchen, A. C., Tran, N. T., Parker, B. J., Bhat, A., Rollo, B., et al. 2021. In vitro neurons learn and exhibit sentience when embodied in a simulated game-world. bioRxiv. doi: 10.1101/2021.12.02.471005
[6] ↑ GBD 2019 Dementia Forecasting Collaborators. 2022. Estimation of the global prevalence of dementia in 2019 and forecasted prevalence in 2050: an analysis for the Global Burden of Disease Study 2019. Lancet Public Health. 7:e105–25. doi: 10.1016/S2468-2667(21)00249-8