Abstract
Bacteria are the microorganisms all around us—on our bodies, in our food, and in the environment. Some bacteria are helpful, but others can cause disease. To learn how bacteria protect or hurt us, researchers usually grow the bacteria in their labs so that they have many of them to study. In this article, you will learn about how we “culture” bacteria in the lab and how different culture methods can affect bacterial behavior. We will tell the story of a new culture system that our research lab developed for studying interactions between different types of bacteria. Finally, you will learn about the clever ways that scientists separate out different types of bacteria, using a method called selective plating. Learning these methods is a fundamental first step for researchers who study how to nurture bacteria that are good for us and fight disease-causing bacteria!
Bacteria Everywhere
Bacteria are the microorganisms all around us—they are on our bodies, in the food we eat, and in the air we breathe. Some bacteria are helpful, but others can cause disease. Scientists want to understand how disease-causing pathogens work so they can help make better treatments for patients. Scientists also want to know how to nurture the good bacteria that keep us healthy. Most researchers study bacteria in a laboratory. Studying bacteria in a speck of soil or from wiping your skin can be difficult [1]. Those samples only have a limited number of bacteria to study and they generally contain a mixture of many types of bacteria. Growing “farms” of separate types of bacteria in the lab is much easier. Researchers can simply grow a lot of a certain bacteria when they want to do an experiment with them. However, “farming” bacteria does not involve green fields or pig pens! Instead, researchers grow bacteria in many kinds of media.
Oh, The Places You Will Grow (Bacteria)
When you hear the word “media,” you might think of TV, movies, or social media. But when microbiologists say “media,” they are talking about how they grow bacteria. Just like farmers feed their chickens different foods than they feed their fish, different kinds of bacteria also need different nutrients to grow. We can make media with various ingredients or recipes to feed specific types of bacteria. Also, media for growing bacteria can be a liquid or solid (Figure 1). Compare chicken farms and fish farms: chickens live on grass (a solid substrate) and fish live in the water (a liquid substrate). Researchers make liquid media into a solid by adding an ingredient called agar. Agar is similar to Jello—it dissolves into hot liquids and turns them into a wiggly solid when cooled down. Scientists use liquid or solid cultures for different purposes. Liquid cultures are like a big bacterial soup—everything is well-mixed. Solid cultures let bacteria grow in structured communities, like cities: what a single bacterium eats and how it talks to its neighbors changes based on where it lives [2].
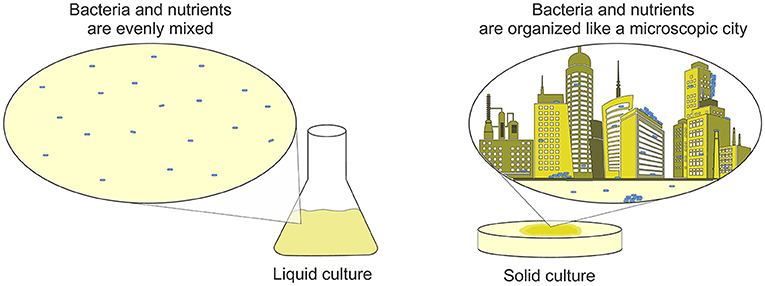
- Figure 1 - In the lab, bacteria can be grown on several different substrates.
- In liquid cultures, the media is continually shaken to mix the bacteria. In solid cultures, bacteria grow in groups. If you think of solid cultures as a city, you can imagine that the bacteria living in the basement of a building have very different lifestyles than the bacteria living in a penthouse! The differences in how much food and oxygen the bacteria receive affects how they grow and behave in experiments.
How Do Scientists Choose Their Media?
So, how do scientists choose which media to use for their experiments? It depends on what questions they want to answer. Scientists who study pathogens that cause skin diseases might want to grow bacteria on solid substrates to imitate how bacteria grow on the skin. Researchers interested in bacterial genes might not worry about how the bacteria grow—a bacterium’s DNA should stay the same no matter how it is cultured. Scientists also choose how nutritious to make the media. Bacteria in the soil are surrounded by nutrients, but skin pathogens often live in nutrient-limited environments. Deciding which media to use is an important first step that scientists take when designing experiments.
Real-World Experiment: Good vs. Evil Escherichia coli
Let us explore a real experimental design process from research done on the bacterial species Escherichia coli (E. coli). You may have heard of this species because E. coli can cause food-borne illness when it grows on beef or lettuce. However, some types of E. coli are actually good for us! These probiotic E. coli can help fight off pathogenic E. coli in the gut. For our research, we wanted to study how a probiotic E. coli strain called Nissle stops another strain of E. coli from surviving drug treatment [3]. First, we grew a model pathogenic E. coli in low-nutrient, liquid media. The minimal amount of nutrients represents an infection environment. We chose liquid culture so that the drug we added would mix in evenly and reach all bacteria. After a few hours, we removed the drug and measured how many bacteria were still alive. Even with a high drug dose, some of the bacteria always survived. We tried adding probiotic Nissle to kill the drug-treated bacteria—and it worked! Fewer pathogens survived when Nissle was added to the culture.
If we could learn how Nissle works, we could try to make it even better at killing pathogens. So, the important question is: how does Nissle kill the other bacteria? Nissle might directly touch the other bacteria to kill them [4]. Or, Nissle could send a chemical attack through the liquid to the other bacteria. It would take a lot of time and money to directly watch our bacteria under the microscope to see if they are touching or sending tiny molecules through the media. Instead, we chose to design a new type of culture system to answer this question.
Designing a New Culture System
We call our new system the H-Cell because the two chambers (or “cells”) look like the letter H (Figures 2A, B). A bridge with a filter in the middle connects the two chambers. The filter has microscopic openings that are too small for bacteria to go through, but big enough to allow liquid and chemical signals to flow between the chambers. Therefore, the filter keeps the probiotic and the pathogen from directly touching. When we repeated our experiment in the H-Cell, we grew probiotic Nissle in one side and the drug-treated cells in the other (Figure 2C). Unlike experiments in a test tube, the Nissle did not kill the drug-treated bacteria in the H-Cell. This result means that Nissle must have physical contact to kill the pathogenic bacteria!
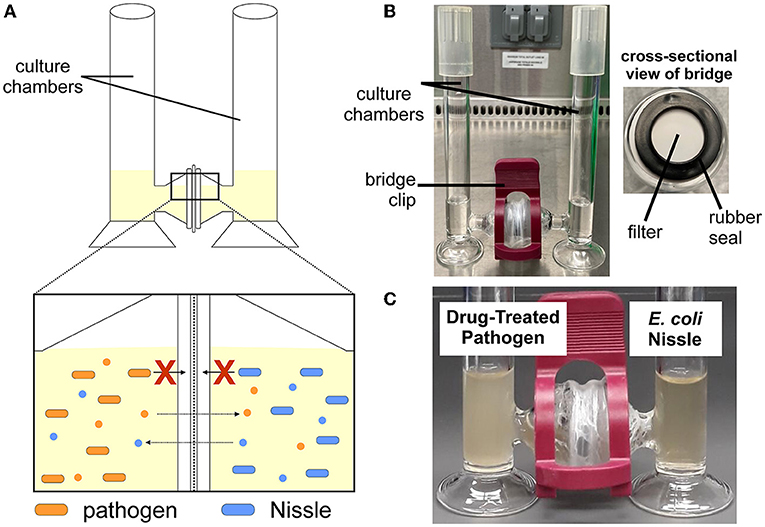
- Figure 2 - (A) In our H-Cell system, there is a filter that divides two chambers.
- The filter keeps bacteria from crossing over but allows chemical signals (small circles) from the bacteria to pass through. (B) This picture of an H-Cell shows the glass chambers and their connecting bridge. The cross-sectional view shows the filter in the middle of the bridge. (C) In our experiment, drug-treated pathogens were cultured in one side of the H-Cell, with probiotic Nissle on the other side.
The H-Cell allows us to ask other interesting questions about bacterial interactions. Unlike the Nissle experiments, not all interactions between bacteria are harmful. When certain bacterial species are together, drugs can be less effective against them. Many researchers are interested in how these bacteria survive as partners [5]. To study bacterial relationships, researchers need a way to count each species separately to measure how well each type of bacteria survives (Figure 3). One method to do this is called selective plating. We first use one liquid culture to grow all of the species together. Then, we take samples of that multi-species culture and transfer them to various types of solid media, each containing a unique combination of nutrients that “selects” for specific species. The number of bacteria that grow on each type of solid media tells us how many of one species survived vs. the others. This method does not work if the various bacterial species have the same diets. In other words, this method does not work if there is no “selective” nutrient to use to grow one species vs. another. This problem could be solved by using the H-Cell. Researchers can study how bacteria interact because the two species can communicate through the liquid media; but, when it is time to count the survivors, the species are physically separate and can be easily measured.
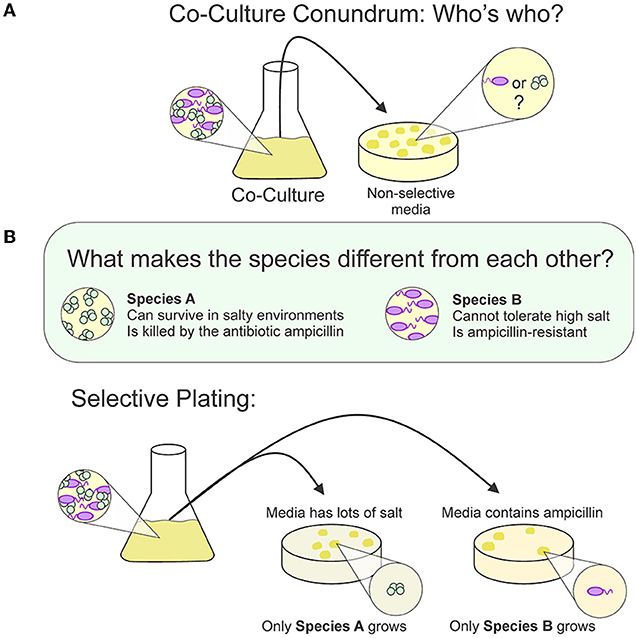
- Figure 3 - (A) If two bacterial species are cultured together and then transferred onto non-selective agar, it is impossible to know whether the bacteria grew from species A or B, or a mix of both.
- (B) If the two species need different nutrients or are killed by different drugs, we can tell them apart using selective plating. When the bacteria are transferred to agar plates, they will only survive under the conditions that support their growth.
Which System Is Best?
As you can see, there are many ways to grow and study bacteria, but each system has its ups and downs. Test tubes with liquid media are easy to work with, but they are not very similar to human skin, soil, or other places where bacteria normally live. Scientists have built microchips that simulate the environment or human organs, but they can be expensive and tricky to use. No system is perfect, but that is why scientific questions are studied in different ways by multiple researchers! Researchers conduct experiments then share the details of their methods and results with other researchers, so that everyone can compare their systems. Altogether, each experiment helps paint a more complete picture of how bacteria work and how we can stop disease-causing pathogens.
Glossary
Pathogen: ↑ Bacteria or other microorganisms that cause disease.
Media: ↑ A mix of specific nutrients used for growing bacteria.
Microbiologist: ↑ A scientist that studies microscopic organisms like bacteria, fungi, and viruses.
Substrate: ↑ What an organism grows in/on; examples include agar gels, liquid media, or solid surfaces like plastic.
Culture: ↑ A batch of bacteria grown in the laboratory (noun); or, to grow bacteria in the laboratory (verb).
Probiotic: ↑ A bacterial species that helps promote a healthy balance of bacteria in our bodies.
H-Cell: ↑ A custom glassware co-culture system. The filter between each bacterial population prevents them from physically interacting, but they can communicate to each other by transferring small molecules across the filter.
Selective Plating: ↑ A method for separating out certain bacteria from a mixture of bacterial species.
Conflict of Interest
The authors declare that the research was conducted in the absence of any commercial or financial relationships that could be construed as a potential conflict of interest.
Original Source Article
↑Hare, P. J., Englander, H. E., and Mok, W. W. K. 2022. Probiotic Escherichia coli Nissle 1917 inhibits bacterial persisters that survive fluoroquinolone treatment. J. Appl. Microbiol. 132:4020–32. doi: 10.1111/jam.15541
References
[1] ↑ Fournier, P. E., Drancourt, M., Colson, P., Rolain, J. M., La Scola, B., and Raoult, D. 2013. Modern clinical microbiology: new challenges and solutions. Nat. Rev. Microbiol. 11:574–85. doi: 10.1038/nrmicro3068
[2] ↑ Manner, C., Dias Teixeira, R., Saha, D., Kaczmarczyk, A., Zemp, R., Wyss, F., et al. 2023. A genetic switch controls Pseudomonas aeruginosa surface colonization. Nat. Microbiol. doi: 10.1038/s41564-023-01403-0. [Epub ahead of print].
[3] ↑ Hare, P. J., Englander, H. E., and Mok, W. W. K. 2022. Probiotic Escherichia coli Nissle 1917 inhibits bacterial persisters that survive fluoroquinolone treatment. J. Appl. Microbiol. 132:4020–32. doi: 10.1111/jam.15541
[4] ↑ Sonnenborn, U. 2016. Escherichia coli strain Nissle 1917-from bench to bedside and back: history of a special Escherichia coli strain with probiotic properties. FEMS Microbiol. Lett. 363:fnw212. doi: 10.1093/femsle/fnw212
[5] ↑ Orazi, G., Ruoff, K. L., and O'Toole, G. A. 2019. Pseudomonas aeruginosa increases the sensitivity of biofilm-grown staphylococcus aureus to membrane-targeting antiseptics and antibiotics. mBio. 10:e01501–19. doi: 10.1128/mBio.01501-19