Abstract
In photosynthesis, plants use energy from sunlight to turn carbon from CO2 in the air into a solid form of carbon that can build the plant’s body. Photosynthesis consists of two portions: the reactions that absorb sunlight energy and another set of reactions called the Calvin-Benson-Bassham (CBB) cycle. When the plant “wakes up” in the morning, after a night of darkness, these two processes do not wake up at the same pace, which can damage the plant cells. However, plant cells prevent this problem by regulating these two processes carefully. To understand how photosynthetic organisms switch from night to day, a type of photosynthetic bacteria called cyanobacteria were used to explore how another pathway, called the oxidative pentose phosphate (OPP) pathway, helps with this dark-to-light transition. Our research found that the OPP pathway can help photosynthesis quickly reactivate when light is available and can prevent cell damage from too much light.
What Is Photosynthesis and How do we Study it?
Plants do not eat, so how do they get their food? Plants can create their own food using a process called photosynthesis. Photosynthesis uses the energy from sunlight to change carbon dioxide gas from the air into solid carbon that a plant can use as food. The solid carbon is used to build the plant’s body, forming structures like lettuce leaves or potato roots that we can eat. To be a source of food, plants must be efficient at growing, which they do by making solid carbon. Understanding the processes that allow plants to grow could help us to improve agricultural production of foods to feed Earth’s growing population. But plants are difficult to study. Sometimes they grow too big to be kept in the lab, they grow too slowly for many experiments, and their genes are difficult to change. So how do we study photosynthesis? We can use a type of bacteria called cyanobacteria, which can perform photosynthesis. Cyanobacteria behave like simplistic plants and they can serve as a replacement for plants in laboratory experiments. Cyanobacteria share enough similarities with plants that experimental results using cyanobacteria can also be applied to plants.
Cyanobacteria may be microscopic photosynthesizers, but their small size does not make them insignificant! Cyanobacteria were the first lifeforms to perform the type of photosynthesis that makes oxygen (the kind we see in plants), and they are probably responsible for putting oxygen into Earth’s airless atmosphere over 2.4 billion years ago [1]. For our purposes, these tiny, simple microbes make an ideal model organism to use to study photosynthesis in the lab.
How Photosynthesis Wakes up in the Morning
In both cyanobacteria and plants, photosynthesis includes two processes: the light reactions, which use sunlight to make energy, and the Calvin-Benson-Bassham (CBB) cycle. The light reactions use cellular machines to absorb light energy from the sun and convert it into chemical energy in the form of high-energy electrons, which are like little packets of energy. These packets of energy are transferred to the CBB cycle to make the carbon molecules that plants or cyanobacteria use to build their bodies. The CBB cycle is dependent on the activity of proteins called enzymes, to convert the carbon in the air to solid carbon. These enzymes also need other cellular materials called intermediates to make their final products.
Let us imagine a plant, or cyanobacterium, at sunset. Since the light reactions need light to give them energy, they cannot make high-energy electrons without the sun, so those reactions are basically “asleep” when it is dark. The CBB cycle also goes to sleep for the night, as it is dependent on light to wake up some of its enzymes, too (Figure 1A). During the day, these two processes provide energy and carbon to fuel cell growth. While these cycles are inactive at night, photosynthetic cells survive by consuming some of the intermediates formed during the day. This creates an issue, since cellular materials are being used up without being replenished.
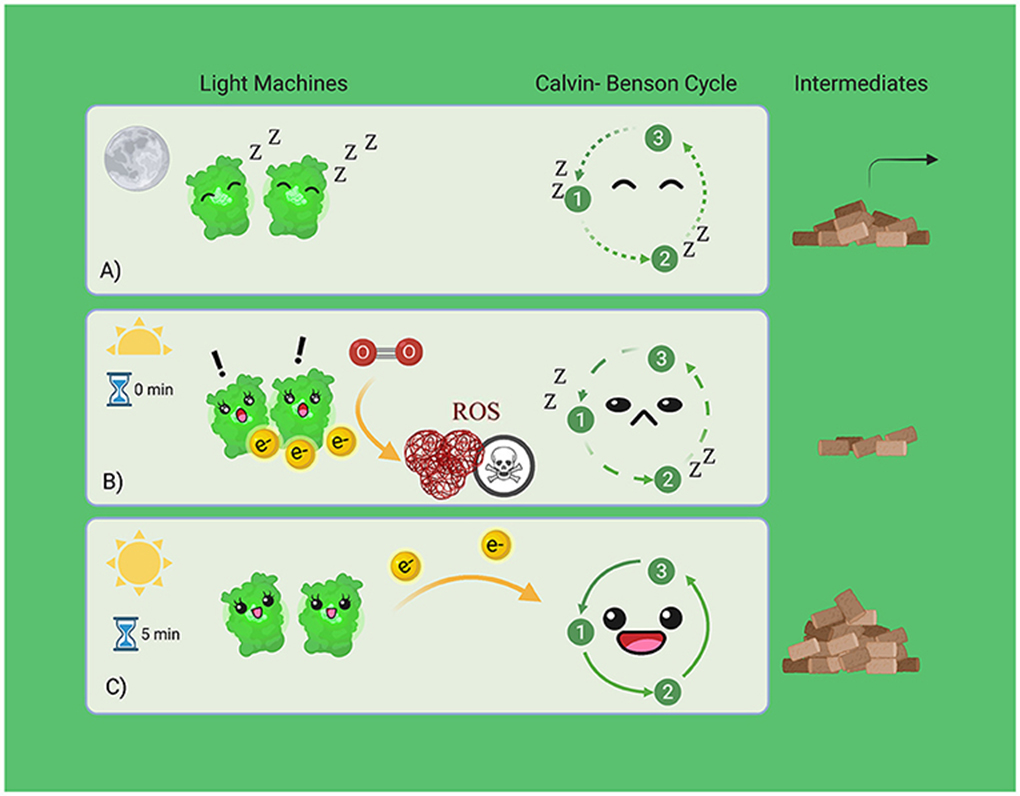
- Figure 1 - (A) Photosynthetic processes are not active at night, because no light is available, so cells use up intermediates to stay alive.
- (B) When cells are exposed to light, the light reactions start but the CBB cycle is slow to wake up and cannot run if no intermediates are left in the cell. Thus, the electrons (e-) from the light reactions cannot go to the CBB cycle, so they end up turning oxygen into harmful reactive oxygen species (ROS). (C) After some time, photosynthesis happens normally again, but it is unknown how this transition happens.
Then the sun rises! The machines that perform the light reactions wake up and start producing high-energy electrons. But the CBB cycle is not ready—although its enzymes wake up with the light, they do so much more slowly, and there are very few intermediates left for the enzymes to work with (Figure 1B). With an inactive CBB cycle, the high-energy electrons from the light reactions have nowhere to go, so they build up and are transferred to oxygen molecules. But oxygen does not handle the high-energy electrons well, and it can become a damaging form called reactive oxygen species (ROS), which damages the machinery of the light reactions and, over time, could kill the cell. But photosynthetic organisms have survived this night-to-day transition for millions of generations. Somehow, the CBB cycle wakes up fast enough and gets photosynthesis operating before major damage occurs (Figure 1C). How does this transition happen?
What Helps Photosynthesis Wake up?
Cyanobacteria have many other chemical pathways besides photosynthesis that help them stay alive during the night. For example, cyanobacteria can store some of the carbon they make during the day, in the form of massive sugar molecules that can be broken into pieces for the cells to use (Figure 2). These pieces are funneled into a series of chemical reactions called the oxidative pentose phosphate (OPP) pathway, which breaks down the sugar to make energy for the cells when photosynthesis is inactive. Scientists used to think that photosynthesis happened during the day and the OPP pathway functioned at night, with only one or the other active at a time [2]. Then it was discovered that the OPP pathway is also active during the day, but not to produce energy like it does at night. Instead, in the daytime, the OPP pathway functions to help the organism transition smoothly from night to day, during the time when the CBB cycle is slowly waking up and cannot pick up the electrons from the light reactions.
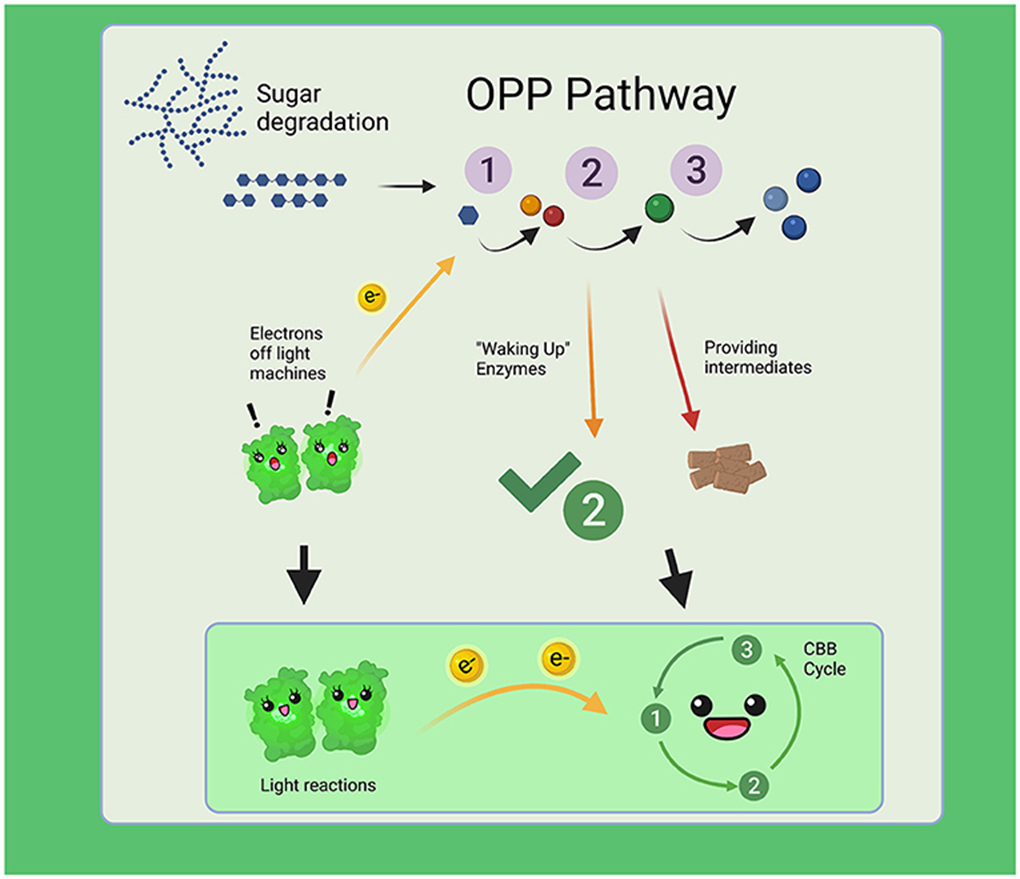
- Figure 2 - The OPP pathway supports photosynthesis during dark-to-light transitions.
- Pieces of the sugar molecules used as fuel for the cells at night are fed into the OPP pathway. When OPP breaks down the sugar, it uses the high-energy electrons from the light reaction machines so that ROS are not formed (yellow arrow). The OPP also “wakes up” some of the CBB cycle enzymes (orange), and it provides important intermediates for the CBB cycle (red).
How does the OPP pathway help? When the pieces of the large sugar molecules are used in the OPP pathway, those processes use up electrons. Therefore, when electrons cannot go to the CBB cycle because it is not awake yet, an active OPP pathway can take up the electrons from the light reactions before they can make ROS. Thus, the OPP pathway helps protect the light reaction machinery by taking away the high-energy electrons before they damage the cell (Figure 2, yellow arrow).
The second challenge of the dark-to-light transition is waking up the CBB enzymes. These enzymes do not wake up as quickly as the light reaction enzymes, but they can be woken up with the chemical version of an alarm clock. The OPP pathway produces these alarm-like molecules that help wake up the enzymes of the CBB cycle (Figure 2, orange arrow).
The final obstacle to restarting the CBB cycle is the missing intermediates, many of which were used up throughout the night as the organism tried to survive without the light reactions. The OPP pathway helps replenish some of the missing intermediates by breaking down large sugar molecules (Figure 2, red arrow). However, many of the other intermediates are not replenished by this pathway. How does an organism get more of these necessary intermediates? This question has not been fully answered, although one theory is that an alternative process called the Entner-Doudoroff (ED) pathway may replenish these intermediates in cyanobacteria [3]. Disruption of the ED pathway decreases growth of cyanobacteria, suggesting the pathway can replenish the missing intermediates. However, the way plants replenish these intermediates is yet to be discovered, since plants do not have the ED pathway.
In summary, the OPP pathway not only helps photosynthetic organisms survive the night, but it also helps these organisms transition from night to day, by promoting a quick “restart.” This role makes the pathway photoprotective, which means it can protect the photosynthetic machinery from the potentially damaging effects of light and keep the cellular processes functioning efficiently.
Lasting Significance
Previously, the idea that the OPP pathway stabilizes photosynthesis was only theoretical [4]. This new work provided experimental evidence of how the OPP pathway could help cyanobacteria cope with the stress of the dark-to-light transition [2]. The major impact of this work is that the results can be applied to plants, since cyanobacteria and plants share the same CBB reactions. Future research using cyanobacteria to learn how to optimize the interactions between the OPP pathway and the CBB cycle could lead to discoveries that could improve photosynthesis. By translating these discoveries to plants, we might be able to make crops grow better, which would help to feed the world’s increasing population.
Funding
This study was supported by the National Science Foundation grant number 2042182. Figures in this work were created with BioRender.com.
Glossary
Cyanobacteria: ↑ Microscopic organisms which create oxygen when they perform photosynthesis, similar to plants, and are used by scientists to study photosynthesis.
Calvin-Benson-Bassham (CBB) Cycle: ↑ The cycle that allows the formation of solid carbon from carbon dioxide using energy from the light reactions of photosynthesis.
Enzymes: ↑ The protein “workers” of the cell that help chemical reactions take place.
Intermediates: ↑ The compounds used by enzymes to complete reactions.
Reactive Oxygen Species: ↑ Forms of oxygen which are toxic due to absorbing too much energy in the form of an electron.
Oxidative Pentose Phosphate (OPP) Pathway: ↑ A pathway that converts sugar molecules to other molecules that can be used in multiple processes, in this case helping photosynthesis.
Entner-Doudoroff Pathway: ↑ A pathway found mostly in bacteria, allowing them to break down sugars using unique reactions.
Conflict of Interest
The authors declare that the research was conducted in the absence of any commercial or financial relationships that could be construed as a potential conflict of interest.
Original Source Article
↑Shinde, S., Zhang, X., Singapuri, S., Kalra, I., Liu, X., Morgan-Kiss, R. M., et al. 2020. Glycogen metabolism supports photosynthesis start through the oxidative pentose phosphate pathway in cyanobacteria. Plant Physiol. 182:507–17. doi: 10.1104/pp.19.01184
References
[1] ↑ Blankenship, R. E. 2010. Early evolution of photosynthesis. Plant Physiol. 154:434–38. doi: 10.1104/pp.110.161687
[2] ↑ Makowka, A., Nichelmann, L., Schulze, D., Spengler, K., Wittmann, C., Forchhammer, K., et al. 2020. Glycolytic shunts replenish the calvin-benseon-bassham cycle as anaplerotic reactions in cyanobacteria. Mol Plant. 13:471–82. doi: 10.1016/j.molp.2020.02.002
[3] ↑ Sharkey, T., and Weise, S. 2016. The glucose 6-phosphate shunt around the Calvin-Benson cycle. J. Exp. Bot. 67:4067–77. doi: 10.1093/jxb/erv484
[4] ↑ Shinde, S., Zhang, X., Singapuri, S., Kalra, I., Liu, X., Morgan-Kiss, R. M., et al. 2020. Glycogen metabolism supports photosynthesis start through the oxidative pentose phosphate pathway in cyanobacteria. Plant Physiol. 182:507–17. doi: 10.1104/pp.19.01184