Abstract
We are surrounded by high-tech materials in our daily lives; for example, in our smart phones or computers. Modern materials can also turn sunlight into electricity, or turn waste CO2 from the atmosphere into eco-friendly chemicals to reduce the need for fossil fuels. These applications often need very specific or even custom-made materials. Materials scientists are always looking for new and/or better materials. They want to learn how materials work, so they can design and improve the properties of the materials to increase their performance. We are a group of materials scientists who do research on materials with customizable properties, called perovskites. In this article, we will tell you how the structure of a material is connected to its properties, and how we can use this connection to create designer materials using perovskites as an example.
Why Are We Talking About Materials?
Materials are the basis of our day-to-day lives. Think about it: all the things we use are made of some kind of material. Examples include building materials like wood, concrete, and steel. We need these materials to build houses, furniture, and infrastructure like streets or bridges. Fabrics are another example. We use fabrics to make clothes to keep us warm during winter, to keep us dry in the rain, and to protect us from the summer sun. Two examples of more complicated materials are silicon chips and polymers. Silicon chips are used in electronic devices. Polymers are the main ingredients of plastics, which have many different applications. Diverse uses of plastics include car tires, Blu-ray disks, packing materials, and space suits.
Materials scientists, as the name suggests, are scientists who study materials. Materials scientists predict how a newly discovered material might behave, or how new materials with certain traits could be produced. In the past, finding the right material for a specific use could only happen by testing lots of different substances. Nowadays, new materials can be specifically designed for any application we want. For example, perovskites are solid materials that are very versatile. Because of their flexibility, they are promising for many uses.
What Are Perovskites?
Perovskites are a large family of solid materials. They are what scientists call crystalline solids (or just crystals for short). The name of this family of materials comes from a mineral that is also called perovskite. A mineral is a solid with a defined composition that occurs in nature in its pure form. The mineral perovskite is fairly common, and it is made up of calcium, titanium, and oxygen. This specific combination of atoms is called calcium titanate by chemists, or CaTiO3 if they want to write in shorthand.
To understand what all members of the perovskite family have in common, we must first talk about what makes a material a crystal. The atoms of a crystal form what is called a crystal lattice, which is a repeating structure in which atoms are arranged in a highly ordered way. That means you only need to look at a small part of a large crystal or rock to describe and study it. This small part is called a unit cell. You can think of it as a box that contains atoms at certain positions. Different materials have boxes with different side lengths and different angles. To “build” a crystal, you only need to repeat this box in all directions (Figure 1A).
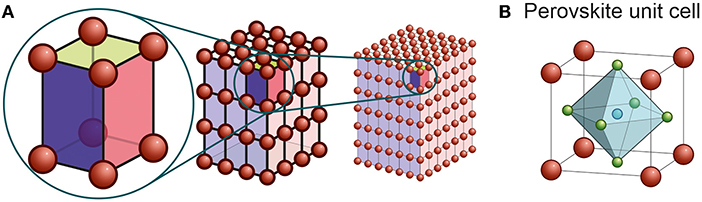
- Figure 1 - (A) A unit cell can make up a larger crystal by repetition.
- The largest crystal in this figure consists of 216 unit cells. However, the unit cell contains all the information about the crystal that we need to understand it. (B) The typical unit cell of perovskites. Large cations (red) make up the corners of the cube. The smaller cation (blue) sits in the middle and is surrounded by six non-metal anions (green). The anions make up an octahedron (a regular double pyramid with 8 faces). The octahedra of neighboring unit cells are connected at their corners.
Thinking of crystalline materials as made up of many unit cells is very useful. Instead of dealing with a huge number of atoms, scientists can focus on just the atoms of the unit cell.
The shape of the unit cell combined with the positions of the atoms in the unit cell makes up the crystal structure. You can imagine a crystal structure as a blueprint. This is the common trait of perovskite materials: they all share the same basic perovskite structure.
Most perovskites contain three different kinds of ions (charged atoms). Two positively charged metal cations—a large one and a small one—and a negatively charged non-metal anion make up the perovskite structure. Perovskites mostly have cubic unit cells—this means all sides of their “box” have the same length and all angles are 90 degrees. The large cations sit at the corners of the unit cell. The small cations are in the middle, surrounded by six non-metal anions. You can see this arrangement in Figure 1B. In fact, these ions do not have to be calcium, titanium, and oxygen. For example, the most abundant mineral on Earth, called bridgmanite, has its atoms arranged in a perovskite structure. Bridgmanite makes up large part of the Earth’s mantle [1].
A Material’s Properties Come From Its Structure
Why should we care about the structure of perovskites? The short and definitive answer is that all of a material’s properties depend on its structure. The structure includes which chemical elements the material contains, how these elements are arranged, and how they are connected. Take for example expensive diamond and cheap graphite—which is found in your pencil, the only difference between those two is how their atoms are arranged. That is why materials scientists almost always start by studying the structure of a new material.
Mechanical properties, for instance, are closely connected to the binding forces in the material. For example, how hard or soft a material is depends on how the atoms are connected. Both diamond and graphite are made up only of carbon. Diamond is one of the hardest materials we know. For this reason, drills used for hard materials are often coated with pieces of diamond. Graphite is one of the softest materials. The reason for the differences between these two materials lies in the difference between the connections between the carbon atoms. In diamond, all the carbon atoms are bound strongly to each other. Graphite is a layered material and the connections between layers are weak. They are so weak that the graphite in a pencil can be used to write on paper.
Any errors in a crystal’s structure can affect the properties of the crystal. These errors are called defects. Defects usually make crystals extremely interesting. Two examples of defects from our own research [2, 3] include vacancies and substitute atoms. Vacancies are spaces where an atom of the crystal is missing. Vacancies can cause atoms to wander through the crystal, which is known as diffusion. You can imagine this situation like a traffic jam. The crystal without vacancies is a highway full of cars with no way to move. If you remove cars from the highway (or atoms from the crystal), movement becomes possible. Substitute atoms are chemical elements that replace some “regular” atoms in a structure. For instance, substitute atoms can change the electrical properties of a substance or can create the colors in many colorful gemstones. If metallic cations like chromium or cobalt replace some “regular” atoms of crystal structures, this exchange makes rubies red, emeralds green, and sapphires blue.
The link between properties and structures is incredibly important in materials science. Why? Because this relationship works in the other direction as well: first we pick a certain property, then we imagine what the material structure should be to create that property. Thus, we can build our own designer material—a unique material that is especially well-suited for our specific application (Figure 2).
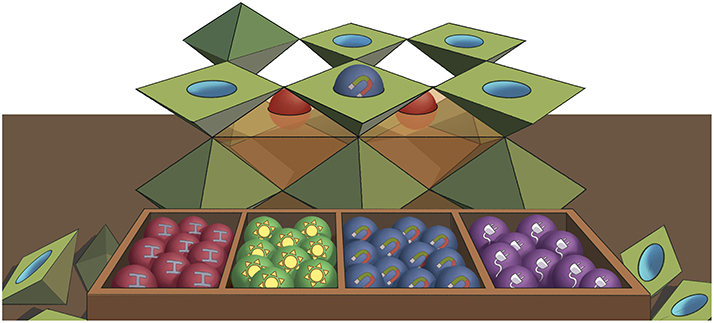
- Figure 2 - We can create materials with different properties by choosing different cations (the balls in the boxes) to sit in the middle of double pyramids (called octahedra) made of anions.
- Elements can be chosen to increase the stability of the material, to give the material the ability to turn sunlight into power, to make the material magnetic, or to allow the material to conduct electricity.
Perovskites as a Construction Kit
As we mentioned before, perovskites are common solid materials. They are used in many applications, for instance in solar cells [4]. Perovskites are excellent building blocks for designing materials for two reasons. First, we can use various chemical elements to form the perovskite, as long as we are careful to arrange them correctly. Using various elements leads to many possible combinations. Common elements for the corners are calcium, strontium, and lanthanum. Iron, titanium, manganese, and silicon can frequently be found in the center. We can include various non-metals as well. For example, oxygen and iodine are quite common. Second, it is easy to dope any perovskite. Doping means putting substitute atoms in the perovskite structure, which gives us the ability to fine-tune properties we want, or even to add totally new properties. For instance, we can use doping to make a perovskite magnetic.
To put this in a different way, think of a construction kit. You can use the same building blocks to construct various buildings. Whether you build a pyramid or a castle depends on how you connect the blocks. Perovskites are like that, but instead of building blocks we use atoms to build materials.
Materials scientists use the perovskite structure as a construction plan. With this plan, they can create a large variety of designer materials by arranging different atoms in the perovskite structure. The properties of these materials depend on the type of building blocks—atoms—used.
Take-Home Message
Modern high-tech applications, like solar cells and smart phones, need materials with specific properties. Sometimes we even need them to have different desirable but opposite properties simultaneously: The wings of a plane are a good example. Wings need to be durable and strong, to carry the whole plane, but at the same time, they need to be lightweight, to minimize the amount of fuel needed. We materials scientists want to understand how properties and structure are connected. That is why we must study materials in detail. We use this understanding to design new materials with the correct properties for a given application. Perovskites are a fascinating family of solids. They are well-suited for designing functional materials (Figure 3). Perovskites are versatile for two reasons: various chemical elements can be used to make different kinds of perovskites; and we can modify and tune the perovskites to adapt them to our needs. We are sure that perovskites are materials that will play important roles in future applications.
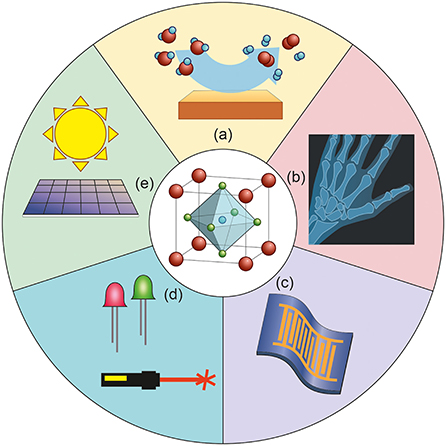
- Figure 3 - Perovskites are important in various applications.
- (a) They play important roles as catalysts, which are substances that make chemical reactions possible, like splitting water into hydrogen and oxygen. (b) Perovskites can be used in imaging; for example, they can be used in X-ray detectors to diagnose injured bones. (c) Perovskites are the basis for flexible and bendable screens in phones or tablets. Flexible electronics are a very interesting and cutting-edge application of perovskites. Perovskites are also used in (d) lasers and LEDs, and (e) solar cells.
Glossary
Crystalline Solid: ↑ A solid material made up of highly ordered and regularly arranged atoms; also called a crystal.
Unit Cell: ↑ A small part of a crystal that is repeated in all directions to build the crystal.
Crystal Structure: ↑ The molecular shape of a crystalline solid, determined by the unit cell together with the positions of the atoms.
Cation/Anion: ↑ Positively/negatively charged atom.
Vacancy: ↑ A type of crystal defect where an atom is missing from its position in a crystal structure.
Diffusion: ↑ The movement of atoms/small molecules through a crystal.
Substitute Atom: ↑ A type of crystal defect in which an atom in the crystal structure is replaced by a different kind of atom.
Dope: ↑ To put substitute atoms into the structure of a material.
Conflict of Interest
The authors declare that the research was conducted in the absence of any commercial or financial relationships that could be construed as a potential conflict of interest.
Acknowledgments
We want to thank the European Research Council (ERC) for financial support through the European Union’s Horizon 2020 research and innovation programme (grant agreement n° 755744/ERC-Starting Grant TUCAS).
References
[1] ↑ Tschauner, O., Ma, C., Becket, J. R., Prescher, C., Prakapenka, V. B., Rossman, G. R. 2014. Discovery of bridgmanite, the most abundant mineral in Earth, in a shocked meteorite. Science. 346:1100–2. doi: 10.1126/science.1259369
[2] ↑ Lindenthal, L., Popovic, J., Rameshan, R., Huber, J., Schrenk, F., Ruh, T., et al. 2021. Novel perovskite catalysts for CO2 utilization-Exsolution enhanced reverse water-gas shift activity. Appl. Catalysis B Environ. 292:120183. doi: 10.1016/j.apcatb.2021.120183
[3] ↑ Lindenthal, L., Ruh, T., Rameshan, R., Summerer, H., Nenning, A., Herzig, C., et al. 2020. Ca-doped rare earth perovskite materials for tailored exsolution of metal nanoparticles. Acta Crystallogr. B (2020) 76:1055–70. doi: 10.1107/s2052520620013475
[4] ↑ Yin, W. J., Shi, T. T., and Yan, Y. F. 2014. Unique properties of halide perovskites as possible origins of the superior solar cell performance. Adv. Materials 26:4653. doi: 10.1002/adma.201306281