Abstract
Positron emission tomography (PET) is a method for taking pictures of a disease inside of a sick person’s body, without having to cut them open. In PET imaging, a radioactive chemical called a radiotracer is injected into a patient. All radiotracers have two main parts: a part that targets the disease, and an unstable atom (radioisotope) that emits positive electrons (positrons) as it breaks down (decays). When a positron crashes into an electron, both are destroyed, and two particles of light emerge. Doctors can detect these radioactive signals and use them to create a 3D image of where the tracer (and the disease) is in the body. This article describes PET and PET radioisotopes in greater detail. We focus on fluorine-18, a radioisotope that makes up the “signaling part” of many important PET radiotracers.
What Is Molecular Imaging?
When a person gets sick, it is not always easy to see what is wrong simply by examining them with your eyes. Sometimes doctors can test a patient’s blood for a suspected disease, or they can remove a small piece of the person and test it in a laboratory. These approaches can give doctors some useful information about the type of disease, but they cannot provide a complete picture of where the disease might be in the patient’s body. Medical imaging is the name for a group of techniques that can be used to look inside people without harming them. The goals of medical imaging are to diagnose a disease, determine its location in the body, and find out how serious it is.
Some medical imaging methods use unstable atoms called radioisotopes to create a signal from inside the patient that can be detected using special scanners. As a radioisotope decays toward something more stable, there is a change in its nucleus (its center, where protons and neutrons are found). At the same time, some type of energetic particle is emitted, which we call radioactivity. Doctors and scientists can detect these radioactive emissions and use them to create images. The machines used to do this are very sensitive, so only a small amount of radioactivity is required. In addition, radioisotopes used for medical imaging usually decay quickly, ensuring that the patient is not exposed to radioactivity for a very long time.
In most cases, a radioisotope will not naturally travel to the site of disease; therefore, it must be attached to a chemical compound that targets something unique or unusual about the disease. We call these compounds targeting vectors. You can think of targeting vectors as police dogs that are trained to sniff out bad guys (diseases). The police are looking for a certain gang of bank robbers that love to spend their stolen money on stinky cheeses. So, the police train their dogs to track down people who smell like stinky cheese. When they find a person who smells like this, the dogs bite down and they do not let go! The dogs have GPS trackers in their collars—these represent the radioisotopes. The collars allow the police to follow the dogs wherever they go and hopefully reveal the location of the bank robbers. The combination of a vector (dog) and a radioisotope (collar) is called a radiotracer. Medical imaging techniques that use radiotracers to target and image health problems are called nuclear molecular imaging techniques.
Nuclear molecular imaging requires different scientists to work closely together. Nuclear physicists and engineers design ways to make the radioisotopes and detect the radioactivity. Chemists make the radiotracers by attaching the radioisotopes to the targeting vectors. We call this “radiolabeling” the vector. Pharmacists make sure that the radiotracers are made in a clean and safe way. Biological scientists test the radiotracers in cells and in animals. Doctors called radiologists look at the images that are generated and interpret them. Medical physicists work with radiologists to predict how much radiation each part of a patient’s body is likely to receive. This information is important because nuclear radiation has the potential to damage living tissues, often by causing cancer. The careful work of radiologists and medical physicists ensures that nuclear molecular imaging provides information that helps doctors make good decisions about the best way to treat a patient, while keeping the patient’s risk of cancer very low.
PET Imaging: Using Radioactivty to Find Disease in the Human Body
Positron emission tomography (PET) is a type of nuclear molecular imaging that uses signals from positron-emitting radioisotopes. Positrons (β+) are identical to electrons (β−), except that they carry a positive charge, not a negative one. When a positron is emitted from a radioisotope’s nucleus, one of the protons that make up the nucleus is changed into a neutron (Figure 1).
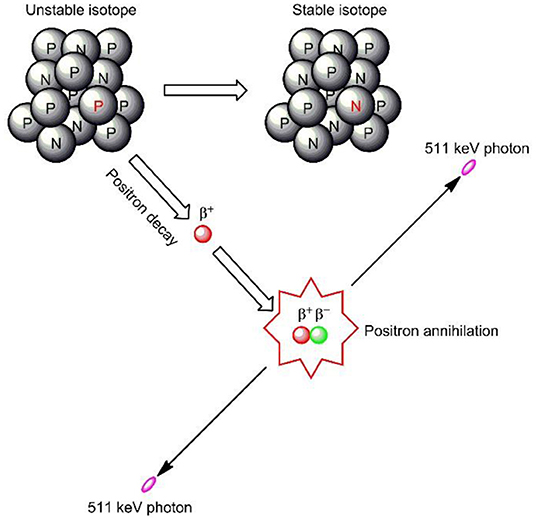
- Figure 1 - Positron emission and annihilation.
- In the unstable atom’s nucleus, a proton (P) changes to a neutron (N) and a positron (β+) is emitted. The positron travels a short distance until it collides with an electron (β−). Both are destroyed, and two photons travel out in opposite directions from the point of annihilation. They have the same energy (511 keV, or “kilo-electron volts”). The photons are detected and used to build an image of where the radiotracer was located. Image adapted from Reddy and Robinson [1].
When positrons travel through the body, they gradually lose energy. Eventually, they collide with an electron from another atom and both particles are destroyed (annihilated) (Figure 1). Their energy is converted into two particles of light called photons. All photons created through positron annihilation have the exact same energy, which makes them easier to pick out from other photons. These special photons always shoot off in opposite directions from each other.
After being injected with a PET radiotracer, a patient is passed through a ring of scintillation detectors called a PET scanner (Figure 2). Scintillation is the process by which radioactive particles passing through certain materials generate flashes of light. Scintillation detectors on opposite sides of the scanner ring are linked. If both detect a photon within a certain amount of time, these photons are considered coincident, and we assume that they came from the same annihilation event. Powerful computers are used to record all these annihilation events and calculate lines of photon emission. They use this information to build a 3D image of where the radiotracer (and the disease) can be found in the patient.
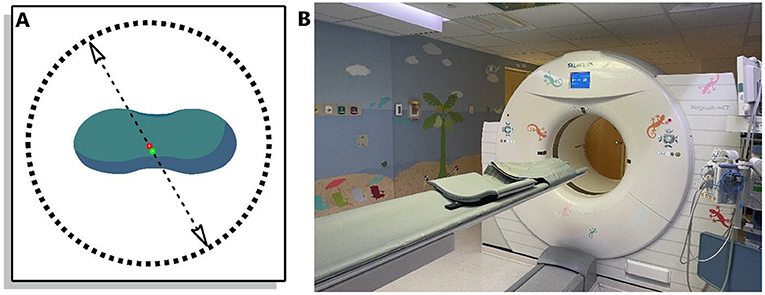
- Figure 2 - (A) The green blob represents a patient lying on their back.
- The plane of the image “slices” through them like a saw. The circle is a PET ring of scintillation detectors. Every positron-electron annihilation creates two photons, which travel in opposite directions from the site of annihilation (along the dashed arrows) and are detected by the PET scanner. (B) The PET scanner at Boston Children’s Hospital. Notice the bed that the children lie down on as they are passed through the scanner (photo credit: Joanne Louis).
What Makes a Good PET Isotope?
There are roughly 3,800 radioisotopes, and 541 of them can undergo positron emission [2]. However, not all are suitable for PET. There are a few important qualities that make a radioisotope useful for PET imaging. First, it should emit enough positrons. Some positron-emitters decay only a tiny bit by positron emission. For example, lead-201 decays only 0.063% by positron emission [2]. Second, radioisotopes that emit low-energy positrons are preferred. The lower the average positron energy, the shorter the distance the positron travels before it is annihilated, and the better the PET scanner can estimate the true position of the radiotracer in the patient’s body. Third, the PET radioisotope should have a relatively short half-life. A half-life is the time that it takes for half of a sample of radioactive atoms to decay. Radioisotopes with short half-lives decay quicker, which results in the patient being exposed to less radiation. PET isotopes have half-lives ranging from a few seconds to a few days, but not weeks or months. Finally, “good” PET isotopes can be prepared in usable amounts, using nuclear reactions that do not generate a lot of additional, unwanted radioisotopes.
How Are PET Radioisotopes Created?
There are two main methods used to produce PET radioisotopes: cyclotrons and generators. Cyclotrons are large, complex machines that can spin protons outwards in a spiral shape, then smash them into pure samples of stable isotopes. This is called cyclotron bombardment. The result is a nuclear reaction that creates the desired radioisotope. Generators are much simpler devices. They take advantage of the fact that some radioisotopes do not decay to stable atoms; instead, they become other radioisotopes, and some of these new radioisotopes are useful for PET. Most generators are about the size of a microwave oven, tipped on its side. Inside, a longer-lived radioisotope is packaged in such a way as to easily flush out a shorter-lived PET isotope that builds up over time. We call this “milking” the generator- the long-lived isotope is a cow and the short-lived isotope is the milk! For example, germanium-68 (68Ge) has a half-life of 270 days and decays to gallium-68 (68Ga). The half-life of 68Ga is only 68.3 min. Whenever a chemist needs to prepare a 68Ga radiotracer, they can “milk” a 68Ge/68Ga generator for 68Ga. Such generators can be milked around 400 times or used for about 1 year.
My Favorite Isotope: Fluorine-18
Is there a “best” PET isotope? Not really…but in our lab, we have a favorite. Fluorine-18 (18F) can be produced in large amounts through cyclotron bombardment of a sample of water that contains oxygen-18 (H218O). Fluorine-18 made this way comes in the form of negatively-charged fluoride (18F−). Fluorine-18 positrons are lower in energy than those created by most other PET isotopes. They have a maximum range in water of 2.4 mm [3]—that is about the thickness of two American dimes. This short distance results in better PET images. Fluorine-18 decays mainly by positron emission (97%). Most cyclotrons can make large amounts of 18F− in less than an hour. Finally, the half-life of 18F is 110 min, which allows for the preparation of complicated radiotracers—as long as the chemist works quickly!
18F-labeled radiotracers have been invented for the imaging of many types of illnesses, including heart disease and certain disorders of the brain, such as Parkinson’s disease. However, the most important 18F radiotracer is [18F]FDG. It is used to diagnose cancer. [18F]FDG looks like a molecule of sugar, except that it has been radiolabeled with 18F. Cancer cells need more energy than normal cells because they grow and multiply faster, so they take up more [18F]FDG. In this case, the “police dog” is the sugar molecule, the signaling “collar” is 18F, the “gang of robbers” are the cancer cells and their need for energy is the stinky “smell” that the robbers give off. In addition to detecting cancer, [18F]FDG can be used to see if a person is responding well to cancer treatment (Figure 3). Ninety percent of all PET scans carried out in the United States are [18F]FDG scans.
![Figure 3 - (A) PET scan of a man with cancer [4].](https://www.frontiersin.org/files/Articles/689970/frym-10-689970-HTML/image_m/figure-3.jpg)
- Figure 3 - (A) PET scan of a man with cancer [4].
- The cancer has spread to many places in his body, which can be seen by the small pockets of high [18F]FDG uptake. (B) The same person, after successful cancer treatment. In addition to cancer cells, [18F]FDG is naturally taken up by some healthy organs, which is why you can see the brain (red arrow), heart (green arrow), and bladder (blue arrow) in the images.
Conclusion
Molecular imaging is a growing area of health science, and PET imaging is at the forefront. Today, scientists are pushing the boundaries of what we can radiolabel and what we can image. PET is more than a fascinating blend of physics, chemistry, and biology—for many people, it is a lifesaver!
Glossary
Radioisotope: ↑ An unstable atom that converts (decays) to a more stable one over one or more steps, in the process emitting radiation. This radiation can be in the form of particles with mass, such as positrons, or photons.
Radiotracer: ↑ A chemical compound that gives off a radioactive signal that can be tracked. Radiotracers are often designed to target sites of disease inside a living being.
Positron: ↑ An antimatter electron, equal in every way except for charge. Positrons are positively charged.
Photon: ↑ A discrete packet of electromagnetic radiation, including light.
Scintillation: ↑ A process in which certain materials will absorb radioactive emissions and give off flashes of visible or ultraviolet light, which can then be measured.
Coincidence Detection: ↑ The detection of two photons at the same time on two different detectors. In PET, coincidence detection is used to identify photons which emerge from the same annihilation event.
Half-Life: ↑ The time it takes for one half of a radioactive sample to decay. Thus, radioisotopes with short half- lives decay faster.
Conflict of Interest
The authors declare that the research was conducted in the absence of any commercial or financial relationships that could be construed as a potential conflict of interest.
References
[1] ↑ Reddy, S., and Robinson, M. K. 2010. Immuno-positron emission tomography in cancer models. Semin. Nucl. Med. 40:182–9. doi: 10.1053/j.semnuclmed.2009.12.004
[2] ↑ Brookhaven National Laboratory 2022. Nuclear Structure and Decay Data. Available online at: https://www.nndc.bnl.gov/nudat3 (accessed April 21, 2022).
[3] ↑ Bailey, D. L., Karp, J. S., and Surti, S. 2005. “Physics and instrumentation,” in PET Positron Emission Tomography: Basic Sciences, ed D. L. Bailey (London; New York, NY: Springer). p. 13–39.
[4] ↑ Little, D. 2020. Lymphoma - deauville 2 (FDG PET-CT). Case study, Radiopaedia.org. Available online at: https://doi.org/10.53347/rID-77502 (accessed April 21, 2022).