Abstract
Just like you and me, plants need a balanced diet to be healthy. However, while we can get most of our nutrients from the foods we eat, plants get their food from photosynthesis—and photosynthesis only provides sugar. A sugar-only diet would not be healthy for you, and it is not healthy for a plant, either. Plants need to get other essential nutrients from the soil around them, and it is not always easy. How do plants get around this? By partnering up with bacteria! In this article, we will note a few key details of these helpful microbes and explain where we found them. We will also describe a few experiments we performed to discover how they help plants to get one particular nutrient, phosphorus.
What Is Phosphorus?
Phosphorus, the 15th element of the periodic table, is a nutrient that is essential for all forms of life. It is part of every cell membrane, the backbone of every molecule of DNA, and part of the main energy currency of all cells (ATP), and it has lots of other jobs in plants, bacteria, and humans. Humans and other animals can get phosphorus from the foods they eat. Plants, however, get their food through photosynthesis, which does not provide phosphorus. As a result, plants must use their roots to absorb phosphorus from the soil.
Unfortunately for the plants, soil phosphorus is often stored in chemical forms that plants cannot use. It is typically in the form of a charged ion called a phosphate ion, bound to other elements to form a molecule. These molecules, which include calcium phosphate, iron phosphate, and aluminum phosphate, are insoluble, meaning they do not dissolve in water. Why is this a problem? Well, imagine stirring a few spoonfuls of salt into a cup of water. Because salt is soluble in water, it will dissolve. However, something different happens if we add the same amount of sand. No matter how much you stir it, the sand does not dissolve, it just sinks to the bottom. Since plants “drink” with their roots, they need to be able to “drink” the phosphorus—so if it is not soluble in water, they cannot use it.
Farmers often get around this problem by adding chemical fertilizers to the soil. These fertilizers contain easy-to-use forms of phosphorus and other nutrients. Unfortunately, there are a few problems with this approach. First, phosphorus fertilizer is not a renewable resource. The phosphorus in fertilizer is mined from rock and soil, and some estimates predict we will run out during the twenty-second century [1]. Also, the amount we do use is not taken up very efficiently. Depending on where you live, only 8–25% of the phosphorus applied as fertilizer actually makes it into the plant [2, 3]. The rest just stays there in the soil, but often in forms that the plants cannot use. These leftovers are called legacy phosphorus. In addition to being inefficient, fertilizer can be environmentally harmful. Water from rain and irrigation can carry the minerals into rivers or lakes, and even the ocean, which can cause overgrowth of algae, pollute drinking water, and harm aquatic life.
Because of all this, you can see that we need a better way to get phosphorus to the plants we farm. Fortunately, some plants have found a solution. They team up with bacteria to get the phosphorus they need!
What Are Endophytes?
For many people, the word “bacteria” can sound a little scary. Folks tend to hear “bacteria” and think of the germs that make us sick. The truth is, most bacteria do not cause disease, and some play important roles in plant and human health. Many bacteria live on or inside of plants (and humans!) and can help plants get nutrients, fight off sickness, or adapt to harsh conditions. In return, the bacteria get a place to live, and they may get food, too. This type of relationship is an example of mutualism—the plants and bacteria work together, and they both benefit. Bacteria that live inside plants in this manner are called endophytes.
Some of these helpful endophytes can live inside plants as well as on a plant’s roots. There are several ways that endophytes can make phosphate soluble and available to plants, but not all are well-understood. One method is for the bacteria to secrete acids into the surrounding soil. These acids help to dissolve the nearby phosphate, so the plant can suck up the phosphorus through its roots. We wanted to learn more about this process, so we studied what happens to the phosphorus once the bacteria get to work.
How Do Endophytes Solubilize Phosphorus?
First, we needed to find bacteria that could dissolve locked-up phosphates. To do this, we looked to the plants that need them the most. We started the search with poplar trees, which can live in nutrient-poor soils on riverbanks in the Northwest area of North America. The riverbank soil has much more sand and gravel than typical soil does (Figure 1A). To survive here, poplars must be able to extract nutrients very efficiently, so these trees are more likely than others to harbor the helpful bacteria we are looking for.
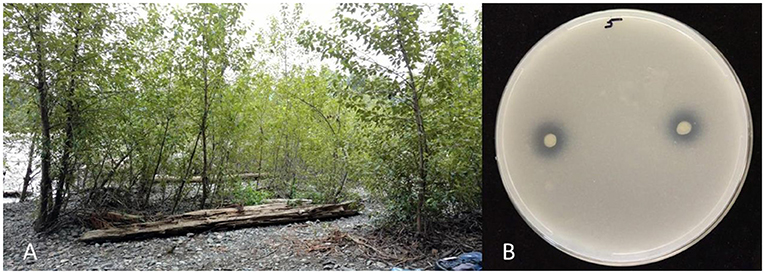
- Figure 1 - (A) Poplar trees growing on the rocky, sandy banks of the Snoqualmie River in the state of Washington (United States).
- The endophytes used in this experiment were isolated from trees in this area. (B) A dish containing agar with calcium phosphate and two colonies of endophytes (white dots). The milky-white calcium phosphate in the agar is solubilized (dissolved) by the bacteria, leaving a clear “halo” around the colonies.
Not all bacteria living on or in a plant can solubilize phosphate. We selected phosphate-solubilizing endophytes by growing them on dishes of bacteria food, called agar, containing calcium phosphate. The insoluble phosphate is initially cloudy, but phosphate-solubilizing strains of bacteria will create a clear “halo” in the dish as they make the phosphate soluble (Figure 1B). We selected two of the most successful strains of bacteria for our next steps.
Next, we asked how well our phosphate-solubilizing endophytes could handle different types of phosphate. To answer this question, we grew two endophyte strains, strain WP5 and strain WP42, in liquid bacterial growth media. We added one of three types of insoluble phosphorus: calcium phosphate, iron phosphate, or aluminum phosphate. By measuring the amount of soluble phosphate before and after growing the bacteria overnight in it, we saw how much phosphate the bacteria could solubilize. We also performed these same steps without the bacteria. This was our negative control, which is a way to verify that our results were actually caused by the bacteria, instead of by some other factor.
We found that our two strains of endophyte bacteria were easily able to solubilize calcium phosphate, but they had more trouble with aluminum and iron phosphates (Figure 2). It is more difficult to solubilize these because they can’t be easily dissolved by organic acids, which are the substance the bacteria use to solubilize calcium phosphate. If we think of the bacteria as “unlocking” the phosphorus, we can imagine that different types of phosphorus require different “keys,” and each type of bacteria carries a unique set of keys to unlock the different forms of locked phosphorus.
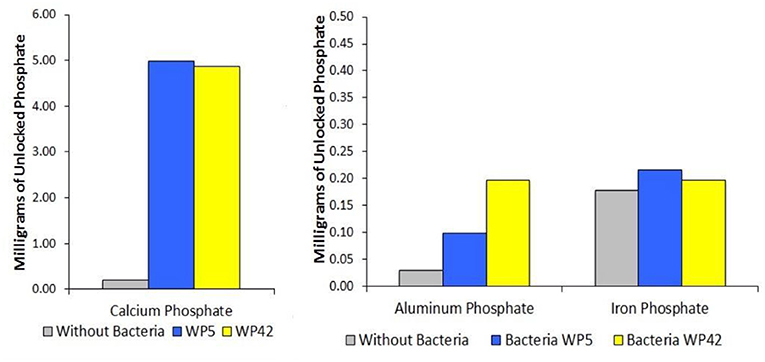
- Figure 2 - Some endophytes can “unlock” the phosphorus from the insoluble, “locked” forms commonly found in soils.
- We used two strains of endophytes, bacteria strain WP5 and bacteria strain WP42. Both strains were grown in liquid containing insoluble calcium phosphate, aluminum phosphate, or iron phosphate. The gray bars represent the negative control, and they show the amount of available (soluble) phosphate present in liquid without bacteria. You can see that our two bacterial strains could easily solubilize calcium phosphate, but they had more trouble with the other two forms of phosphate. Notice that the scale bars are different on the two graphs.
How Do Endophytes Help Plants?
Once we knew which bacteria could solubilize phosphate, we wanted to learn more about how their activity might benefit plants. To monitor phosphate uptake into plant roots, we grew small poplar trees (a few inches tall) in a food solution containing insoluble phosphate, with and without the endophytes WP5 and WP42. We looked at the roots with high-tech microscopes that allowed us to track the movement of phosphorus into the roots, and we also measured the plants’ production of certain proteins related to growth.
As expected, plants grown with the bacteria strains WP5 and WP42 took in more phosphate from the soil. Not only was there less insoluble phosphate left in the medium, but there was also more phosphorus inside the root (Figure 3). We observed several other changes as well. First, the plants “turned on” more genes related to metabolism in the presence of endophytes. This makes sense because the plants have more of the nutrients needed for growth when phosphorus is unlocked. Second, plants grown with endophytes also showed fewer molecules that indicate stress. Third, the roots of the plants treated with WP5 and WP42 were significantly larger, but with fewer fine root hairs. This is a sign that the plants without the bacterial helpers were stressed by lack of nutrients and were growing more roots to try to find more.
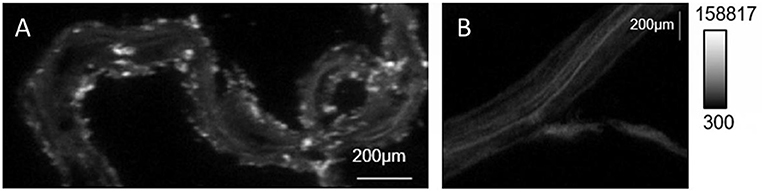
- Figure 3 - Images of phosphorus in poplar roots, taken with a high-tech microscope.
- Lighter colors indicate higher amounts of phosphorus taken up into the root. (A) The roots grown with phosphate-solubilizing bacteria have higher phosphorus intake than do (B) plants that are grown without endophyte bacteria. Source: Varga et al. [4].
The story does not end when the insoluble phosphate becomes soluble and gets taken up into the roots. Because the plant is also taking up the calcium and other minerals along with the phosphorus, it turns out that these soluble phosphates can become “locked up” again inside the plant. While this might seem odd, it makes sense: the bacteria on the root surface primarily affect what happens on the outside of the root. The reason that the plants can use the phosphate once it is inside them is because the endophyte bacteria move inside the plant as well. We found that the endophytes WP5 and WP42, which are naturally found both inside and outside of the plant, are able to keep on helping the plant! Unlike most bacteria which are limited to the soil, the behavior of endophytes may help to keep phosphate available once it is inside the plant!
The Future
These are exciting results, and they bring us another step closer to fully understanding the relationship between plants and their microscopic friends. In the future, we hope that more farmers will harness the power of the bacteria that live on plants, so that they can rely less on chemical fertilizers. By adding the proper bacteria to plants, we may be able to help plants use the legacy phosphorus that is already in the soil. The end goal is to find ways of making essential nutrients available to plants without needing to apply chemical fertilizers that pollute the natural world around us. Until then, keep an eye out in the great outdoors, and remember: for much of the plant and animal growth you can see, you have got microbes to thank!
Funding
This research was supported by the Strategic Science Area intramural program at the Environmental Molecular Sciences Laboratory (EMSL), a DOE Office of Science User Facility sponsored by the Office of Biological and Environmental Research and operated under Contract No. DE-AC05-76RL01830. Experiments performed at the ALS were supported by the DOE Office of Science under contract No. DE-AC02-05CH11231, experiments at the APS experiments were funded by the DOE Office of Science under contract No. DE-AC02-06CH11357. Microbial experiments were performed with funding from the Byron and Alice Lockwood Foundation.
Glossary
Phosphorus: ↑ The 15th element of the periodic table. Phosphorus is an essential nutrient for all forms of life. Humans and other animals get our phosphorus from the food we eat. Plants get their phosphorus from the soil around their roots.
Phosphate Ion: ↑ A phosphorus atom with a negative charge. Phosphate ions are typically bound to positively charged ions. For example, calcium phosphate is made up of calcium ions bound to phosphate ions.
Insoluble: ↑ Unable to be dissolved in a liquid. Something that is soluble can be dissolved.
Legacy Phosphorus: ↑ Phosphorus left over in agricultural fields from years of applying phosphorus-based fertilizers. Since plants only use some of the fertilizer, legacy phosphorus is often present at high levels in the environment.
Mutualism: ↑ A relationship between organisms of different species, in which each organism benefits.
Endophytes: ↑ An organism that lives inside a plant in a mutualistic relationship. Endophytes can contribute to many aspects of plant health, including nutrient availability, drought tolerance, and resistance to disease.
Strains: ↑ A lineage of genetically identical bacteria. All bacteria within a strain can trace their ancestry back to the same single cell. A strain is similar to a species, but more specific like a variety of the species.
Negative Control: ↑ A measurement in an experiment performed without the experimental treatment. In this experiment, our negative control was the plants grown without endophyte bacteria.
Conflict of Interest
The authors declare that the research was conducted in the absence of any commercial or financial relationships that could be construed as a potential conflict of interest.
Original Source Article
↑Varga, T., Hixson, K. K., Ahkami, A. H., Sher, A. W., Barnes, M. E., Chu, R. K., et al. 2020. Endophyte-promoted phosphorus solubilization in populus. Front. Plant Sci. 11:567918. doi: 10.3389/fpls.2020.567918
References
[1] ↑ Cordell, D., Drangert, J-O., and White, S. 2009. The story of phosphorus: global food security and food for thought. Glob. Environ. Change 19:292–305. doi: 10.1016/j.gloenvcha.2008.10.009
[2] ↑ Ma, L., Ma, W. Q., Velthof, G. L., Wang, F. H., Qin, W., Zhang, F. S., et al. 2016. Modeling nutrient flows in the food chain of China. J. Environ. Quality 39:1279–89. doi: 10.2134/jeq2009.0403
[3] ↑ van Dijk, K. C., Lesschen, J. P., and Oenema, O. 2016. Phosphorus flows and balances of the European Union Member States. Sci. Tot. Environ. 542:1078–93. doi: 10.1016/j.scitotenv.2015.08.048
[4] ↑ Varga, T., Hixson, K. K., Ahkami, A. H., Sher, A. W., Barnes, M. E., Chu, R. K., et al. 2020. Endophyte-promoted phosphorus solubilization in populus. Front. Plant Sci. 11:567918. doi: 10.3389/fpls.2020.567918