Abstract
As you walk, bike, or drive down the street, your brain puts together (integrates) sensory information from different sources. It uses what you see, what you hear, and how your head and body move to help you judge your own movements. As you move, your brain must ensure that the world does not look blurry and that you can judge how fast you are moving, how far you are moving, and the direction you are heading. And of course, falling and collisions should be avoided! Self-motion is the term we use for movements we make with our bodies, such as walking, running, jumping, or even sitting still. This article explains how our brains achieve the amazing task of perceiving our own movements and how we use this information to stay balanced and navigate our world. It also describes what happens when errors in self-motion occur (due to illness/injury) and how the science of self-motion perception can enhance video games and virtual reality.
Our Senses Help Us to Know That We Are Moving
We make all kinds of movements with our bodies. Sometimes the movements we make are very small and subtle, like when we are trying to stand very still. Sometimes our movements are large, such as running after a football. But big or small, we always use several important senses (sensory systems) to interpret and guide these movements. These sensory systems include our visual system (sight), auditory system (hearing), proprioceptive system (muscles and joints), tactile system (touch), and vestibular system (velocity and acceleration) (Figure 1). How do these sensory systems work together as a team to help us move efficiently and safely?
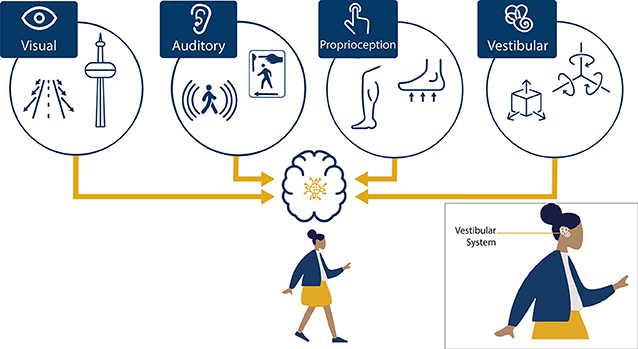
- Figure 1 - The brain uses a combination of sensory inputs, visual (sight), auditory (sound), proprioception (muscles and joints), and vestibular (velocity and acceleration), to perceive self-motion.
- The box on the lower right shows the location of the vestibular system, which is found in the inner ear.
Sensory Inputs in Action
Let us try a small movement as an experiment. First, stand in one place and try to stay still. Even this simple task requires your brain to put together information from many different sensory sources. One system that plays a big role is the visual system. When you are moving, your visual system makes use of landmark cues (like a tall tower in the middle of a city) and changing visual motion (like the lines in the highway flying by as you drive past) to know how much you are moving. While standing still, close your eyes and see what happens. It probably becomes harder to stay stable, which means that vision is used to support balance. Next, try lifting one foot off the floor. Information from the tactile (touch) sensors in your feet tell you if the ground is flat, and your muscles and joints provide information about where your body parts are in space and relative to each other. These inputs are referred to as your proprioceptive system. Finally, stand on something soft. This will further challenge your proprioceptive system, and you may move around more than when you were standing on a solid surface. The vestibular system is located in the inner ear (Figure 1) and it is our brain’s “acceleration detector,” meaning that it detects acceleration. Have you fallen over yet? Hopefully not, because once your brain detects that you are off-balance, it sends messages to your muscles to adjust your body to help you avoid falling.
For a large movement, like when you are playing soccer and running after the ball, it is important to coordinate sensory inputs so that the world is not jittery or blurry. As your head turns left and right, the signals from the vestibular system are processed in milliseconds and tell your eyes which way to move, which is known as the vestibular-ocular reflex. In this way, the vestibular system helps you to keep your eyes stably on the ball while your head bobs up and down, without the image of the ball becoming blurry… GOAL!
Even though the sensory systems typically work together to help us with self-motion, sometimes certain sensory inputs are more reliable than others. For instance, at night-time, or when it is foggy out, visual information is less reliable, and therefore the brain relies more heavily on other sensory inputs, such as proprioception and/or vestibular inputs. Recent studies have investigated how vision, proprioception, hearing, and vestibular information each contribute to the experience of self-motion [1].
Self-Motion Experiments
To understand how each sensory input contributes to the experience of self-motion, it is helpful to remove individual sensory inputs to see what happens. For example, how does removing vision, using a blindfold for example, affect our ability to judge the distance we have traveled, our movement speed, or our movement direction? It turns out that we can actually still perform pretty well when one sense is missing! But if we want to know how each sensory input contributes when all inputs are available, as is the case during most everyday interactions, it becomes difficult to manipulate each input independently. Modern virtual reality (VR) technologies have made this challenge easier (Read more in this Young Minds article; to the experience of self-motion [2]). To see some videos of the VR equipment that the authors have used in their experiments see the links [3, 4].
Using VR, the authors studied the contributions of visual and vestibular information for judging heading direction (Figure 2A), which means knowing which way you are going. Participants were seated on a moving platform that moved them forward to the left or forward to the right, at very precise angles [5]. Participants also watched a projection screen that made it look like they were in space, flying through a cloud of stars. The task for participants was simply to judge whether they moved to the left or to the right. Sometimes the platform moved them at very large and obvious angles and sometimes the angles were very small. We measured the smallest possible angle that participants could sense. The heading task was performed with vision only, motion only (vestibular), and with both vision and motion together. The results showed that participants were equally good at the heading task using their visual system or their vestibular system, but that they were better when using both systems together.
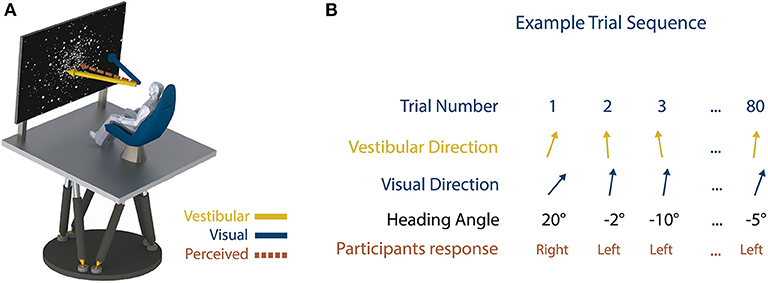
- Figure 2 - (A) Experiment setup; motion platform and visual display.
- The participants were moved forward to the left or to the right but the vestibular and visual input moved at slightly different angles creating a sensory conflict. Participants were asked to say whether they felt like they moved left or right. (B) Sample results from one participant. The experiment consisted of 80 trials (repeats). The top row is the trial number, the second and third row show the angle of direction for the visual and vestibular stimuli and the fourth row is the heading direction. The fifth row shows the participant’s response. Overall, the results of this experiment showed that participants used the vestibular information more than the visual information.
To further investigate whether participants relied more on the visual or vestibular estimates when both systems were available, we used a VR experimental trick (very difficult to achieve in the real world). Specifically, participants were presented with a visual heading direction moving forward and 5° to the left, but a vestibular heading direction (motion) moving forward and 5° to the right! This is called introducing a sensory conflict (Figures 2A,B), and most participants are not aware that a conflict has been introduced. This technique can be used to estimate how each sensory input is “weighted” by the brain. By analyzing participants’ responses, we showed that participants weighted the vestibular cues higher than the visual cues, meaning that they used the vestibular information more than the visual information when deciding on their heading direction.
Self-Motion Illusions
Most of the time we remain completely unaware that the brain is smoothly combining inputs from our senses as we go through our daily lives. However, sometimes we can experience self-motion illusions. A good example of this is the “train illusion.” This illusion is experienced when you are sitting on a non-moving train and the train beside you begins to move–your brain is briefly tricked into thinking that it is your train that is moving. This is because when most of your visual field is moving, it usually means that you are moving through the world and not that the world is moving past you. The scientific term for the visual illusion that makes you think you are moving is vection.
Video game developers and movie producers often use vection to enhance the feeling that you are actually moving as you play a first-person action adventure video game or view a movie. The more of the visual field that the visual motion occupies and the more the experience stimulates most of your sensory systems, the greater vection you will feel. However, care must be taken not to cause a unique kind of motion sickness referred to as “visually induced motion sickness,” or simulator/cyber sickness.
Self-Motion Perception Can Be Different for Different People
The way we perceive self-motion changes from birth to older adulthood. As we get older, we often experience declines in our sensory systems, such as poor vision, muscle weakness, and hearing loss. These declines mean that it is even more important for the brain to integrate sensory information as we age. For example, if you receive strong vestibular signals while walking down the street, these signals may be good at judging your body movements with little help from your other sensory systems; however, if the vestibular signals are weak, it would help to have additional evidence from the visual or auditory system. This is important because as people experience age-related declines in their sensory systems and/or differences in how they combine sensory inputs, there may be serious consequences, including falls and car crashes. For this reason, we are researching these questions in older adults to help prevent these types of accidents from happening [6].
Other medical disorders can affect self-motion perception and mobility. For instance, Parkinson’s disease is a disorder of the brain that causes individuals to have difficulties controlling their own movements, such as during walking. Some of these difficulties may be due to problems coordinating sensory inputs that usually support safe mobility (See more in this Young Minds article; What are neurodegenerative diseases and how do they affect the brain?) [7]. The more research we do to understand diseases like Parkinson’s, the better we will be able to help people with these diseases in the future [8].
Conclusion
Recent research has shown that effective self-motion perception relies on the successful integration of visual, proprioceptive, auditory, and vestibular information to support safe mobility when walking, biking, and driving. Failure to coordinate all the sensory inputs could have serious consequences, including getting lost, colliding with objects, or falling. By understanding how the brain puts together these sensory inputs, advances can be made to support healthy aging, to treat movement disorders, and even to develop new virtual reality technologies for education and entertainment.
Glossary
Proprioceptive System: ↑ Senses our muscles and joints.
Vestibular System: ↑ Senses velocity and acceleration and helps inform eye movements, it is located in the inner ear (Figure 1).
Self-motion: ↑ When someone moves, like walking, running, cycling, driving, or even sitting on a moving plane.
Heading Direction: ↑ Is the direction you are moving.
Sensory Conflict: ↑ When two senses give different information, like if the visual system says you are moving right and the vestibular system says you are moving left.
Vection: ↑ Is the illusionary feeling of self-motion.
Conflict of Interest
The authors declare that the research was conducted in the absence of any commercial or financial relationships that could be construed as a potential conflict of interest.
Original Source Article
↑Butler, J. S., Smith, S. T., Campos, J. L., and Bülthoff, H. H. 2010. Bayesian integration of visual and vestibular signals for heading. J. Vis. 10:23. doi: 10.1167/10.11.23
References
[1] ↑ Campos, J. L., and Bülthoff, H. H. 2012. “Multisensory integration during self-motion in virtual reality,” in Frontiers in the Neural Bases of Multisensory Processes, eds M. M. Murray and M. Wallace (Boca Raton, FL: CRC Press; Taylor & Francis). p. 603–28.
[2] ↑ Penn, R., and Hout, M. 2018. Making reality virtual: how VR “tricks” your brain. Front. Young Minds 6:62. doi: 10.3389/frym.2018.00062
[3] ↑ iDAPT Young Innovators. 2018. CEAL Mini Tour. Available online at: https://www.idaptyouth.com/blank (retrieved November 26, 2019).
[4] ↑ Bülthoff, H. H. 2013. The MPI CyberMotionSimulator. Retrieved from: https://www.youtube.com/watch?v=ThkymYRP1g8
[5] ↑ Butler, J. S., Smith, S. T., Campos, J. L., and Bülthoff, H. H. 2010. Bayesian integration of visual and vestibular signals for heading. J. Vis. 10:23. doi: 10.1167/10.11.23
[6] ↑ Ramkhalawansingh, R., Keshavarz, B., Haycock, B., Shahab, S., and Campos, J. L. 2017. Examining the effect of age on visual–vestibular self-motion perception using a driving paradigm. Perception 46:566–85. doi: 10.1177/0301006616675883
[7] ↑ Berman, T., and Bayati, A. 2018. What are neurodegenerative diseases and how do they affect the brain? Front. Young Minds 6:70. doi: 10.3389/frym.2018.00070
[8] ↑ Fearon, C., Butler, J. S., Newman, L., Lynch, T., and Reilly, R. B. 2015. Audiovisual processing is abnormal in Parkinson’s disease and correlates with freezing of gait and disease duration. J. Parkinsons Dis. 5:925–36. doi: 10.3233/JPD-150655