Abstract
Investigation of human diseases and testing new drugs require large numbers of human cells. To get enough cells, scientists force cells to proliferate (divide) and produce more cells, or they select only the cells that have the ability to proliferate indefinitely. However, the cells that can proliferate without stopping often lose their original properties and start to look and act a lot different from the original cells in the human body. We developed a tool that allows us to turn ON and OFF cellular proliferation on command and studied how this affects the cells’ function. With the use of the tool, we observed that proliferating blood vessel cells do not form vessels as efficiently as non-proliferating cells do. We also learned how to produce sufficient numbers of cells while preserving normal cell function.
From a Single Cell to the Whole Body
The human body is made up of trillions of tiny building blocks called cells. Not all cells of the body are the same. Cells that make up the skin are different from the cells in the eye, for example, in their size, shape, the way they are connected to each other, and the way they communicate with other cells. There are about 200 different cell types in the human body! And every cell type is designed to perform specific functions.
It is astonishing that the whole human body develops from a single cell, the fertilized egg. Formation of the body from a single cell is a complex process, which requires the single cell to divide many times and also requires the specialization of the cells to allow them to perform their specific tasks. The increase in cell number is achieved by a process called cell proliferation, where a single cell divides and produces two identical cells called daughter cells. Cellular specialization requires a different process called differentiation. During differentiation, daughter cells start to differ from the parental cell and become better suited for a particular function.
If you take a close look at your body, you will see that it is mainly made of fully differentiated cells that have specific functions. Your skin, liver, heart, and muscles mainly consist of specific types of fully differentiated cells. However, most of the cells in these organs do not proliferate at all. So, where does this vast number of differentiated cells come from? Differentiated cells are offspring of so-called progenitor cells. Progenitor cells proliferate easily and can produce daughter cells that can differentiate into a subset of different cell types, but the progenitor cells themselves have not developed enough to fulfill any specific function. Progenitor cells, in turn, come from completely non-differentiated cells, called stem cells. Stem cells can proliferate indefinitely, but they lack specific functions that would allow them to do any of the jobs in the body. Stem cells give rise to every tissue, and they are mainly found as the body develops. However, only very few stem cells can be detected in adult organs. In adults, stem cells mainly serve as a “repair system”, to replace damaged cells. The human body develops from stem cells that produce progenitor cells, which then give rise to fully differentiated cells. Along the way, cells gain more functions but lose the ability to proliferate. In a way, differentiation and cellular function are opposing each other during development (Figure 1A).
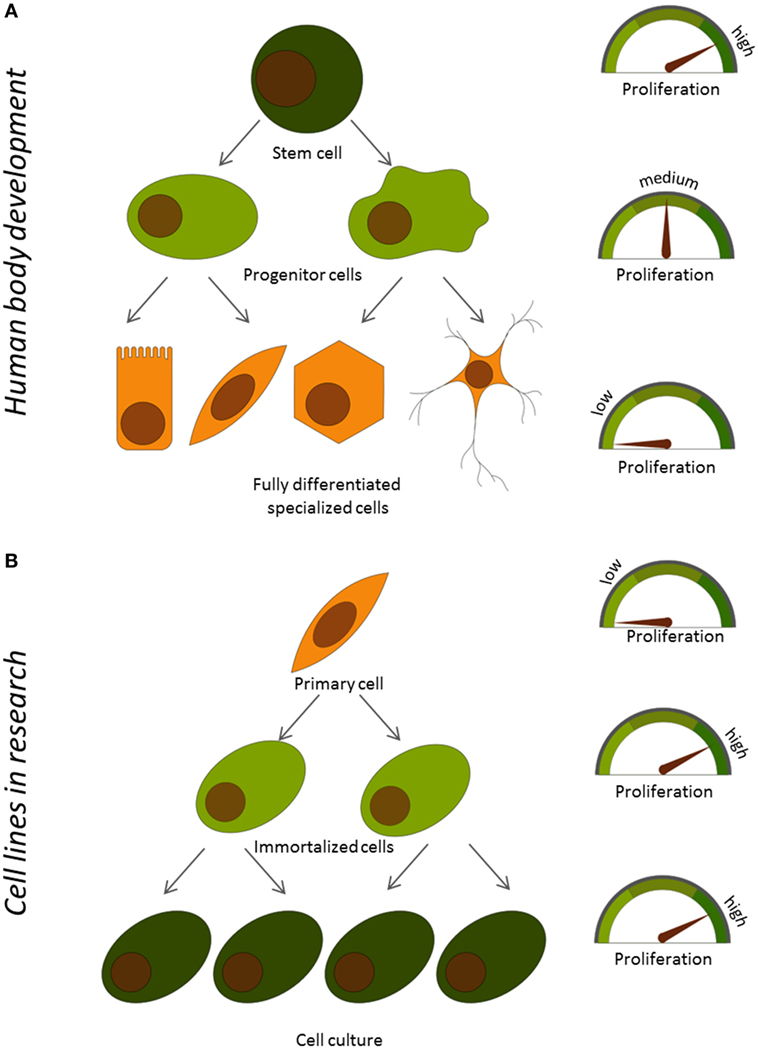
- Figure 1
- Relationship between proliferation and function of cells A. During development in the human body and B. In primary and immortalized cells used in the lab.
Vessel Cells and How to Study Them
Thinking, walking, eating, reading—anything we do depends on fully differentiated cells doing their job right. Understanding the human body requires studying the specific cell types. Let us say, we are interested in how we think. In this case, we will have to study brain cells. If we would like to learn how we walk, we will have to investigate muscle cells. If we would like to know how the body produces energy from food, we will have to have a closer look at stomach cells.
In our work, we are interested in blood vessels. Some blood vessels can be seen by the naked eye through the skin, but most of them are hidden and spread throughout the whole body! Blood vessels are responsible for transferring oxygen and nutrients from blood to the body’s tissues, making sure other cells stay healthy. Blood vessels are made of cells called endothelial cells. Endothelial cells maintain the structure of the blood vessels and if a vessel is broken, the endothelial cells start the process of blood clotting. Dysfunction of endothelial cells is thought to be the reason for some diseases, like high blood pressure, heart attack, stroke, and many others. Some viruses and bacteria infect endothelial cells and cause harm to the body. Studying endothelial cells is thus very important in order to understand these diseases and to find ways to cure or prevent them.
But, how do we get enough endothelial cells to study? “Take them from humans,” you may answer. But the question is, how do we get the large number of cells necessary to perform the experiments? Cells that are directly obtained from the human body are called primary cells. Obtaining primary endothelial cells is very difficult. More importantly, only a limited number of primary cells can be isolated from a person without doing them any harm. This number is not sufficient to study the cells or to test new drugs. Also, since primary cells are fully differentiated, they do not proliferate much after they are taken from the body, so the number of isolated cells will not increase over time. Scientists have learned how to modify primary cells in the lab, so that they proliferate. This process is called immortalization and the resulting cells are called immortalized cells.
Immortalized cells from blood vessels are called immortalized endothelial cells, and they are very useful for testing new drugs. However, immortalized endothelial cells are not exactly the same as the primary endothelial cells in the body. During immortalization, these cells often lose the traits they acquired during differentiation and are not able to perform the same functions as that of the primary endothelial cells (Figure 1B). So, we asked: can we improve the function of immortalized cells by stopping proliferation? To find the answer, we had to develop a tool to switch proliferation on and off on command, and we then had to compare what happens with proliferating versus the non-proliferating cells.
Gene Switches and How We Construct Them
Every one of us has a unique genome that consists of thousands of genes we inherited from our parents. Every cellular function has at least one specific gene responsible for it. Despite the fact that all the cells in the body have the same genome, each cell type only turns on the specific set of genes that are needed to fulfill that cell type’s job. So, cells obviously have a way to switch on genes on command—how do they do it? To control its activity, every gene has a switch called a promoter. When the promoter is active, the gene attached to the promoter is turned on and performs its function. When the promoter is inactive, the gene is turned off, and the function is absent.
For our purpose, we used an artificial promoter, called the tet-promoter. This promoter is usually inactive, because it is missing its trigger. The trigger consists of two parts: an activator (a protein) and a drug called doxycycline. If the activator and doxycycline are present, they form a complex that can bind to the tet-promoter and switch it on. However, if the drug is absent, the activator cannot do its job. If the activator is already present in the cell, we just need to feed the cells with doxycycline, so the two parts can assemble into the complex and activate the promoter (Figure 2A). That is how we can control the action of the promoter.
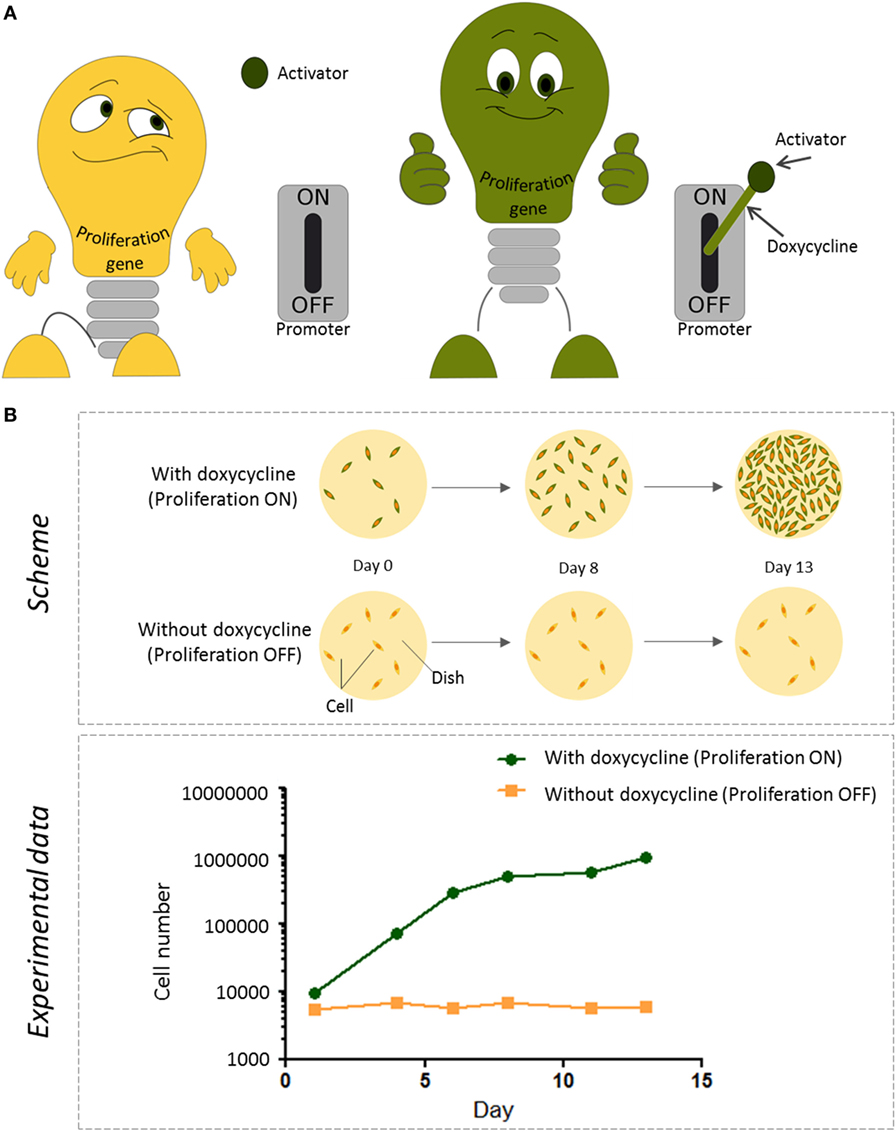
- Figure 2
- How we control proliferation of immortalized cells in the lab. A. The tet-promoter is like a switch that can be used to turn a proliferation gene on or off. Usually, the tet-promoter is switched off and the cells do not grow. Activation of the tet-promoter only happens in the presence of an activator protein (which is already in our cells) and doxycycline, a drug we can add to the cells. The presence or absence of doxycycline thus determines whether the proliferation gene is switched on or off. B. Top: the schematic representation of the experiment to monitor proliferation of cells. Immortalized cells carrying both the tet-promoter switch and the activator were grown on two culture dishes (yellow circles, viewed from top). The day the cells were put on the dish was called Day 0. Then, the cells were grown on these dishes either in the presence or absence of doxycycline, for 13 days. The addition of doxycycline switched on the proliferation gene and the cells divided, resulting in more cells per dish. Bottom: to see how much the cells proliferated, the cells in each dish were counted on days 1, 4, 6, 8, 11, and 13. In the presence of doxycycline (green line), the number of cells on the dish were increased over time; in contrast, in the absence of doxycycline (orange line), cells did not divide and the cell number stayed the same [1].
Which gene would be the best one to control to make cells divide on command? To identify which genes could do the job, we closely studied one of the most threatening human diseases—cancer. Cancer cells can proliferate indefinitely and, by doing so, they harm the body. We figured out which genes force cancer cells to keep dividing. These are called proliferation genes. Tumor cells are unable to turn these genes off and that is why cancer cells are so dangerous. But, would it be possible to control proliferation by linking the proliferation genes to switches that we control by the addition of doxycycline?
To test whether this would work, we inserted a proliferation gene attached to the tet-promoter, as well as an activator, into endothelial cells. When we did not feed the cells with doxycycline, the promoter was inactive, and the proliferation gene was not expressed. In this case, the number of endothelial cells stayed the same over time (Figure 2B). However, as soon as we added doxycycline, the promoter turned on the gene. The cells started to divide, and the cell number increased. We started with 10,000 cells and obtained a million cells within 2 weeks! Thus, we confirmed that the cells we created with the proliferation gene inserted were able to proliferate in response to doxycycline.
Does Proliferation Affect the Function of Endothelial Cells?
Having created the tool to turn proliferation on and off when we wanted, we then asked if proliferation actually messes with the function of endothelial cells. To do that, we needed to compare the functions of proliferating cells with those that cannot proliferate.
One way to compare the functions of proliferating and non-proliferating cells is to measure the activity of the genes that endothelial cells need to do their job in the body. We analyzed 45 genes that are normally active in primary endothelial cells. In the non-proliferating immortalized cells, 40 of these genes were activated, but when we switched off proliferation, only 34 genes were turned on. This told us that non-proliferating immortalized cells are more similar to primary cells. However, does it really mean that the non-proliferating cells are doing their job better?
In the human body, endothelial cells build vessels, which are hollow tubes that transport liquids (blood and another fluid called lymph) through the body. It turns out that we can mimic the construction of vessels in the lab. Normally, we keep cells in plastic dishes that allow them to randomly spread over the flat surface, forming one layer of cells. In a living body, however, the cells do not grow on plastic, but they are supported by a specific scaffold, called the extracellular matrix (ECM). If we let the cells grow on ECM in a plastic dish, we observe that, instead of being scattered on the surface, the cells attach to each other in a specific way and form tubules, which look like tiny, curved straws that can be seen with a microscope. The tubules grow in various directions and intersect with each other, forming a net. The length and number of tubes can give us information about how well the endothelial cells are able to do their job.
We compared primary human endothelial cells with our immortalized endothelial cells in this experiment. To do so, we grew the cells on an ECM and kept them either with or without doxycycline, to control proliferation. Afterward, we counted how many tubules were built under these conditions and compared the number of tubules made by primary cells versus immortalized cells. It turned out that the immortalized, proliferating cells did not form tubes as efficiently as non-proliferating cells did. Overall, the non-proliferating cells formed tubes as efficiently as primary cells (Figure 3). The data confirmed that proliferation does interfere with endothelial cell function, and that non-proliferating cells do their job better than cells that are proliferating.
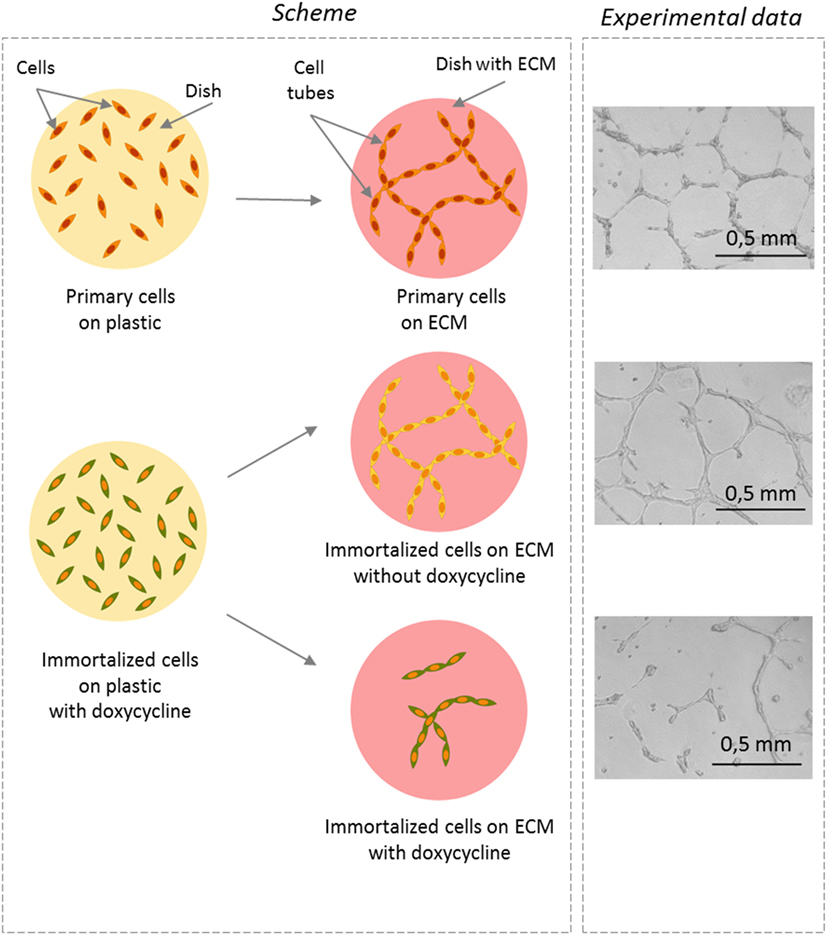
- Figure 3
- Does proliferation affect the ability to form tubules? Left panel: the schematic representation of the experiment. Drawing of how primary or immortalized endothelial cells look like when they are cultured on a simple plastic dish (round yellow circle) or on a dish covered with extracellular matrix (ECM) (red circle). You can see that the cells are dispersed on plastic but they form tubules if they are seeded on an ECM. Right panel: photographs were taken with a microscope 16 h after seeding the cells on ECM. In the absence of doxycycline, the non-proliferating cells (shown in yellow) formed tubules, as did the primary cells. This indicates that the non-proliferating cells were quite similar to the primary cells. In contrast, when doxycycline was added and the cells proliferated (shown in green), less tubes were visible and the tubes were shortened and did not form the network. This means that proliferation interferes with endothelial cell function.
How Could We Further Benefit from Proliferation Controlled Endothelial Cells?
We developed immortalized cells that we can force to grow on command. On the one hand, by adding doxycycline, we can make these cells proliferate in order to obtain the number of cells needed for our experiments. On the other hand, if we remove the doxycycline, the cells stop proliferating and perform the endothelial cell functions as well as the primary cells from the body do. Thus, by inserting a proliferation switch, we can increase the number of cells and at the same time avoid the dilemma of balancing proliferation and function.
The resulting immortalized cells can be grown indefinitely and used for various experiments. In particular, they can be used to investigate the role of endothelial cells in different diseases. Recently, we confirmed that, like primary endothelial cells, the immortalized endothelial cells can be infected by two human viruses, called Kaposi’s sarcoma-associated herpesvirus and cytomegalovirus. Kaposi’s sarcoma-associated herpesvirus is a rare virus, which mostly hides silently within the human body without causing any harm. However, in some cases, when the immune system of the patient is not working correctly, the virus causes a cancer called Kaposi’s sarcoma. This cancer is severe and there is no reliable treatment or vaccination. We are using the immortalized cells to investigate how the virus changes the cells into cancer cells, so that we can develop methods to stop it. We also use the immortalized endothelial cells to identify new drugs that eliminate the virus and slow down the cancer growth caused by the virus. With the help of the immortalized endothelial cells, we have already identified several potential drugs that might be used to cure patients in the future.
Glossary
Proliferation: ↑ A process in which a single cell divides, producing two identical daughter cells and thereby increasing the cell number.
Differentiation: ↑ Cell maturation and acquisition of specific properties, required to fulfill a certain set of functions in the various tissue of the human body.
Primary cells: ↑ Unmodified cells, isolated directly from the body.
Immortalization: ↑ Generation of continuously proliferating cells.
Immortalized cells: ↑ Continuously proliferating cells, either derived from tumors or generated in the lab by modification of primary cells.
Gene: ↑ A region of DNA, encoding the information for a specific cellular function.
Promoter: ↑ A region of DNA, controlling gene expression by switching it on or off.
Extracellular matrix: ↑ Various molecules that are secreted from cells and which provide a scaffold for cell growth and development in the body.
Conflict of Interest Statement
DW has filed a patent concerning the technology for the establishment of conditionally immortalized cell lines. The other author declares that the research was conducted in the absence of any commercial or financial relationships that could be construed as a potential conflict of interest.
Reference
[1] ↑ Lipps, C., Badar, M., Butueva, M., Dubich, T., Singh, V. V., Rau, S., et al. 2017. Proliferation status defines functional properties of endothelial cells. Cell. Mol. Life Sci. 74(7):1319–33. doi:10.1007/s00018-016-2417-5