Abstract
Matter is what we call all of the “stuff” that makes up the universe. Ordinary matter, such as atoms, makes up only about 15% of all the matter in the universe. The other 85% is called “dark matter”—it is invisible but important enough to hold our galaxy, the Milky Way, together so it does not break apart. What is dark matter? For decades, astronomers have been thinking of ideas and trying to test these ideas by observations. One popular theory called “cold dark matter (CDM)” has been widely successful in explaining dark matter in the universe as a whole, but it still needs to be tested inside our own galaxy. In this study, we show how computer simulations can help us use streams of stars in the galaxy to determine whether CDM is the correct theory to explain dark matter.
1. What Matters in the Universe
Since the early 1920s, astronomers have known that our galaxy, the Milky Way, consists of mostly stars holding onto each other by gravity. When you look at galaxies, from a photograph or through a telescope, you are looking at the light of these stars. In the 1970s, while astronomers were carefully studying the motions of stars in the Milky Way, they realized that the stars are moving too fast. In fact, the stars are moving fast enough that they should have escaped from our galaxy a long time ago. This means that if the Milky Way was made of only the stars that we can see, then it should have broken apart by now!
Therefore, the Milky Way must contain something that we cannot see, but something that is important enough to hold the stars in the galaxy. This invisible matter is now known as dark matter. Dark matter has been, and still is, a puzzle to scientists for decades. To understand why dark matter is so puzzling, let us compare it with “ordinary” matter that we understand. Everything we see and touch everyday is made of atoms of elements from the periodic table. Even the stars we see are made of hydrogen, helium, and little bits of other elements. From our everyday lives, we know that ordinary matter either shines light on its own (like stars, light bulbs, etc.) or reflects light (like walls, trees, etc.). Ordinary matter can also block light to cast shadows. The not-so-ordinary dark matter does not do any of that. It does not shine, reflect, or block any light (so it is not actually “dark,” but “invisible”). The only way to tell that there is dark matter is by its gravity. It is this gravity that is holding the stars in the Milky Way, so that they do not escape out into the universe.
Perhaps the biggest puzzle is that there are not just little bits of dark matter here and there that we can ignore. For the last 30 years or so, scientific observations have shown that the entire universe is filled with dark matter. In fact, only about 15% of all the matter in the universe is ordinary matter, and the other 85% is dark matter [1]. So, the universe is mostly filled with a kind of matter that we do not understand! Dark matter is one of the biggest research topics in astronomy today. The answer to “what is dark matter?” may just be worthy of a Nobel Prize.
2. The Quest to Understanding Dark Matter
One of the first steps of the scientific method is coming up with a hypothesis, or just an educated guess or prediction about how/why something happens. The prediction must be tested by experiments or observation to see if that guess is right or wrong. For example, a famous hypothesis is that the speed at which an object falls to the ground does not depend on that object’s mass. This hypothesis predicts that if you dropped a hammer and a feather from the same height at the same time in vacuum (without air resistance), then they should hit the ground at the same time. To test this, an astronaut actually tried dropping a hammer and a feather while standing on the Moon1 (which does not have air), and he showed that the hammer and the feather really hit the ground at the same time. This is evidence that the hypothesis was true.
Even though astronomers do not understand exactly what dark matter is, over the years they have had lots of ideas. One popular theory is called “cold dark matter (CDM).” It is “cold” not because it would feel cold when you touch it, but “cold” means that dark matter is made of particles that do not move around very quickly. The theory of CDM has a hypothesis which predicts that groups of galaxies tend to arrange themselves into organized structures. After spending years observing galaxies, astronomers found that groups of galaxies are arranged in almost exactly the same way that the hypothesis in the theory of CDM predicts they should be!
3. Almost, but Not Perfectly
There is very strong evidence for CDM’s description of how groups of galaxies are arranged, but there isn’t much evidence for the insides each individual galaxy. Let us look at what is inside a galaxy like the Milky Way [2]. The stars in the Milky Way are arranged in a flattened disk with a spiral pattern. These stars that we can see are the visible part of the galaxy. They sit in the middle of a much bigger halo that is made of dark matter, and this is the dark matter that provides the gravity to hold the stars together in the middle (Figure 1).
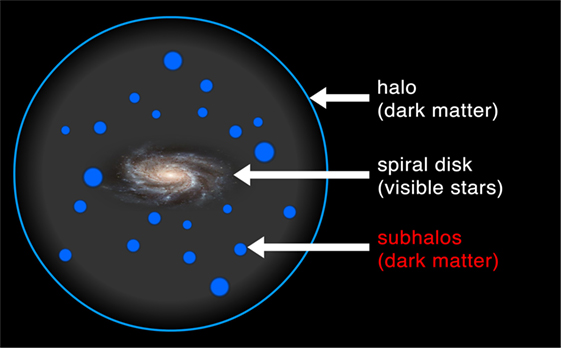
- Figure 1 - The components of the Milky Way galaxy (not drawn to scale).
- Astronomers are quite sure that the Milky Way has a dark matter halo around the outside and a spiral disk of stars at the center. A hypothesis in the theory of cold dark matter predicts that the Milky Way also has dark matter subhalos, shown as blue dots, but we do not know if this idea is correct. To confirm the theory, we need a way to see if there are subhalos.
A hypothesis in the theory of CDM predicts that our disk of stars, the Milky Way galaxy, is not alone in the halo. Rather, our galaxy is surrounded by many clumps of dark matter called subhalos. Subhalos are like mini versions of the halo nested inside the halo itself. Even though astronomers are quite sure that the Milky Way is inside a dark matter halo, they are not sure if this halo contains subhalos. If we found that the Milky Way does have subhalos, then we would have one important piece of evidence that supports the theory of CDM. Otherwise, we might need a different theory to explain dark matter.
4. Turning the Tides
How can we look for subhalos that are made of invisible matter? Even though we cannot see dark matter directly, we can see how it influences visible matter such as stars. Stars like to gather with their neighbors into clusters because of their mutual gravity—they attract each other. However, star clusters do not last forever, and they often get ripped apart by the galaxy’s tidal force.
The galaxy’s tidal force works similarly to the Moon’s tidal force on Earth. The reason the Earth has tides is that the gravitational attraction between the Moon and the Earth is not the same everywhere on Earth. The city closest to the Moon is attracted to the Moon more strongly than the city farthest away from the moon (Figure 2). The Earth feels this difference in the gravitational attraction as if the Earth is “stretched” from one end to the other. This stretching force, or tidal force, creates tides in the oceans.
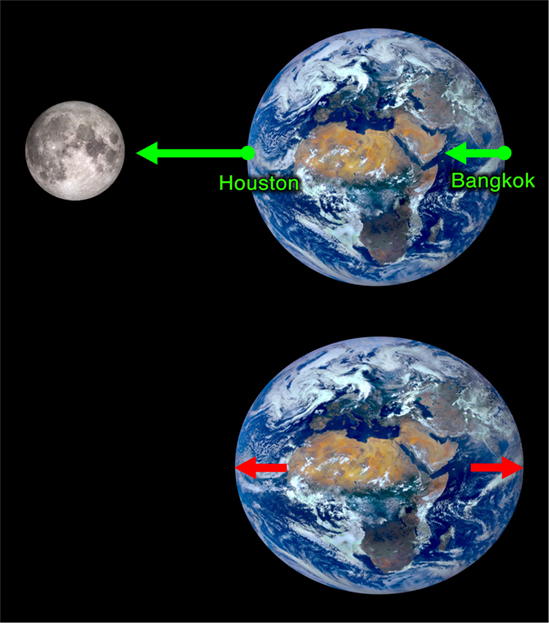
- Figure 2 - An example of tidal force.
- The Moon’s gravitational attraction is different on two sides of the Earth. In the figure (not drawn to scale), Houston is closer to the Moon, so its attraction to the Moon is stronger than Bangkok’s attraction to the Moon (top). On Earth, this difference in the attraction force is felt as if the Earth is stretched from Houston to Bangkok (bottom).
The tidal force on a star cluster works the same way. A star cluster in the Milky Way is always attracted toward the center of the Milky Way, but this attraction force is different on the two sides of the star cluster.
The galaxy stretches the star cluster just like the Moon stretches the Earth, but with one big difference. The Earth is mostly a solid body, so the Moon’s tidal force only stretches it a little bit, like an elastic. However, a star cluster is a group of stars, and the galaxy’s tidal force can easily rip the group apart! As stars get pulled off from their cluster, they form a long “stream” of stars—stars lining up one after another—that wraps around the galaxy (Figure 3). This process is sometimes known as “spaghettification.” The process of tearing a star cluster into a star stream usually takes billions of years, and a star stream can grow up to hundreds of thousands of light-years long. One light-year is the distance that light can travel in 1 year, which is about 9.5 trillion kilometers!
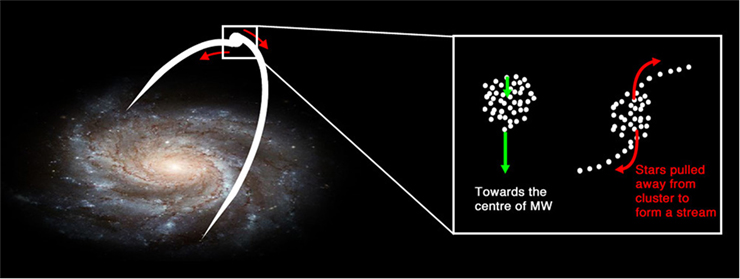
- Figure 3 - How a stream of stars form under the Milky Way’s tidal force.
- Similar to the Moon and the Earth, the Milky Way’s gravitational attraction on a star cluster is not the same everywhere on a star cluster. This figure is similar to Figure 2. The green arrows represent the difference in attraction toward the center of the Milky Way, and the red arrows represent the stretching force felt by the star cluster. As the star cluster gets stretched, stars get pulled away from the cluster one after another to become a stream that wraps around the galaxy. It is the stretching force that causes the stream to form, so the stream “grows out” from the star cluster in the middle.
5. Gaps in the Streams
How can bright streams of star help us find dark matter subhalos within our galaxy? When a subhalo gets close to a star stream, the stars very near the encounter could get knocked out of the stream by the subhalo. This creates a “gap” in the star stream (Figure 4). Think of cars moving on a busy highway. When a car takes an exit, there is a gap in the traffic. Similarly, when a star escapes from a star stream, the stream would have a gap.
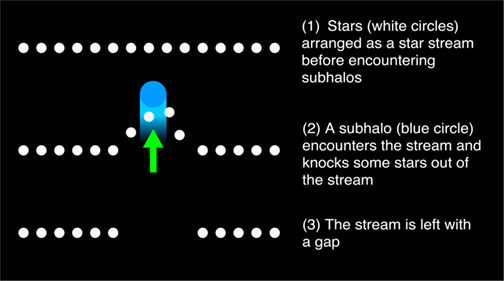
- Figure 4 - How a gap forms in a star stream.
- Before encountering a subhalo, a star stream is made of stars lined up one after the other, similar to cars in the traffic. When a subhalo encounters the stream, the subhalo knocks some stars out of the stream. The stars near the point of encounter are gone, and this creates a gap in the stream.
So, if there are subhalos in the Milky Way, then they would encounter star streams, and the star streams would have a lot of gaps in them. Looking for gaps in star streams can help us find out if there are subhalos in our galaxy, and this helps us figure out whether the theory of CDM is correct. However, star streams are complicated. They are very sensitive to other things in the galaxy. In fact, even if a galaxy does not contain subhalos, a star stream may still have gaps. This means that when we observe a star stream in reality and find gaps in it, the gaps are not always caused by subhalos.
A big challenge when looking for gaps in star streams is to tell whether an observed gap was actually caused by a subhalo. This is where computer simulations are needed. In a simulation, we have the freedom to “construct” a galaxy in any way we like. Recall from Figure 1 that we know the Milky Way has at least a halo and a disk, but we do not know if there are subhalos. We can simulate two star streams: one stream in a galaxy that has subhalos, and one stream in a galaxy that is identical to the first except for the fact that it does not have subhalos. Then, we can compare the difference between these two star streams, and we know that the difference must be caused by subhalos because everything else in the simulation is the same.
Figure 5 shows two simulated star streams. These star streams were originally very thin and wrapped around the galaxy like a long ribbon. In this figure, the star streams have been straightened out and magnified so that we can see what happened to them. Stream A formed in a galaxy that only had a halo, and Stream B formed in a galaxy that had both a halo and subhalos. Neither galaxy in the simulations included the disk to make things simple.
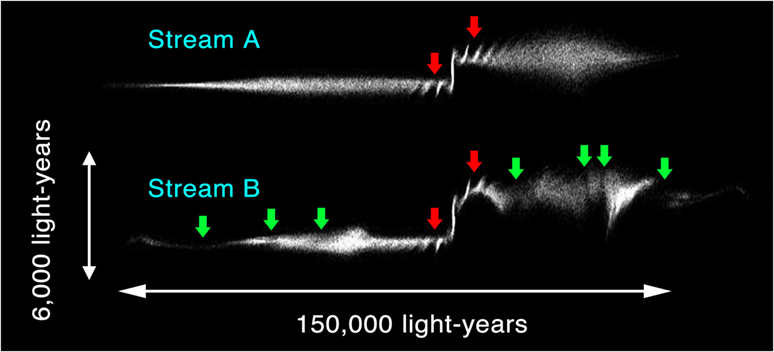
- Figure 5 - Two computer-simulated star streams.
- These star streams wrap around the simulated galaxy similar to what is illustrated in Figure 3, but these two streams have been “straightened out” so that it is easier to see what is happening to them. These two streams are identical, but Stream A was simulated in a galaxy without any subhalos, whereas Stream B was simulated in a galaxy with subhalos, like the one shown in Figure 1. The red arrows indicate gaps that show up in both streams, so we know those gaps are not caused by subhalos. On the other hand, the green arrows indicate gaps that show up only along the messy structure in Stream B, so those gaps must be caused by subhalos. To see if there are subhalos in the Milky Way, astronomers observe whether actual streams look like Stream A or B (one light-year is the distance that light can travel in 1 year).
Although Stream A did not encounter any subhalos, it still has gaps in the middle (red arrows in Figure 5). On the other hand, Stream B encountered many subhalos, and its gaps (green arrows in Figure 5) are very different than the gaps in Stream A. This tells us that gaps caused by subhalos can occur anywhere along the stream and have different sizes. These computer simulations can help us to look at star streams in the real world and determine which gaps in the stream are caused by subhalos and which are caused by other effects.
6. The Future
The research using gaps in star streams to investigate dark matter is still in a very early stage. The galaxy for Streams A and B in Figure 5 included only the halo and subhalos, but not the disk of stars. If we include the disk, will that cause gaps in star streams too? Also, the halo used for the streams in Figure 5 is roughly, but not exactly, the same as the Milky Way’s halo. Will star streams have more gaps if they are formed inside a halo that is more similar to the Milky Way’s halo? The answers to these questions require more computer simulations.
Ultimately, we must look for gaps in observed star streams in reality, and not just in computer simulations. In order for us to have confidence in the theory of CDM, we will need to observe gaps from as many star streams as possible.
Astronomers have found about 10–20 star streams so far in the Milky Way that may be good to observe for testing the theory of CDM [3]. Fortunately, they are likely to find even more star streams in the near future, bringing us closer to understanding dark matter.
Glossary
Hypothesis: ↑ An educated guess that makes a prediction. This prediction must be tested to see if the guess is right or wrong.
Cold dark matter (CDM): ↑ A theory that dark matter is made of slow-moving particles. Much research today focuses on testing the hypothesis that this theory makes.
Star cluster: ↑ A tight group of stars bound together by their own gravity, similar to a very small galaxy. The are many star clusters in the Milky Way galaxy.
Tidal force: ↑ The stretching force felt by a planet or other body of matter because of the difference in gravitational forces on different sides of the body.
Conflict of Interest Statement
The author declares that the research was conducted in the absence of any commercial or financial relationships that could be construed as a potential conflict of interest.
Original Source Article
↑ Ngan, W. H. W., and Carlberg, R. G. 2014. Using gaps in N-body tidal streams to probe missing satellites. Astrophys. J. 788:181. doi:10.1088/0004-637X/788/2/181
References
[1] ↑ Planck Collaboration, Ade, P. A. R., Aghanim, N., Armitage-Caplan, C., Arnaud, M., Ashdown, M., et al. 2014. Planck 2013 results. XVI. Cosmological parameters. Astron. Astrophys. 571:A16. doi:10.1051/0004-6361/201321591
[2] ↑ Binney, J., and Merrifield, M. 1998. Galactic Astronomy. Princeton Paperbacks. Princeton, NJ: Princeton University Press.
[3] ↑ Grillmair, C. J. 2010. Stellar debris streams: new probes of galactic structure and formation. In Galaxies and Their Masks, eds D. L. Block, K. C. Freeman, and I. Puerari, 247. New York, NY: Springer.
Footnote
[1] ↑ http://nssdc.gsfc.nasa.gov/planetary/lunar/apollo_15_feather_drop.html