Imagine you are standing on third base and waiting to sprint to home plate to win the game for your baseball team. You watch as your teammate approaches the batter’s box and raises the bat to her shoulder. You are ready to spring into action as soon as you hear the smack of the ball hitting the bat. The pitcher winds up and releases the ball. It whizzes through the air, and CRACK! The ball jolts off the bat. You are primed and ready to go, you start to accelerate, when, suddenly, the second-baseman leaps up and catches the line drive in midair. You freeze in your tracks without a moment’s hesitation, still safely on the base.
Introduction
How did you do that? You were so prepared to sprint toward home plate, with your leg muscles tense, but then, in the blink of an eye, you were able to cancel your planned actions and stop yourself.
Being able to stop actions is an important behavior. It allows humans and animals to rapidly react when situations change, whether it be stopping in your tracks when you turn a corner and see a snake in your path, or stopping from picking your nose when you realize your teacher is looking right at you!
Believe it or not, scientists study this behavior all the time! This type of behavior is an example of what is called response inhibition. Inhibition comes from the verb ‘to inhibit,’ meaning to prevent or hold back. It is called “response inhibition” because your body is activated to make a response, just like being ready to run toward home plate in the baseball example. Then, to cancel the response, you must ‘inhibit’ the activity. This inhibition may be the result of a signal, like a snake in your path, or may simply happen because you make a decision to stop, without any signal from the world around you. An interesting question concerns whether or not response inhibition can be automatic. This is one of the questions scientists are still trying to understand.
Stopping yourself may seem simple, but being able to inhibit a response requires fast adjustments to your actions. This ability relates to a wide range of real-life skills. For example, imagine you are about to chase a basketball into the street and you suddenly hear the honking of a car horn. Not being able to stop in such a situation would have serious consequences. Can you think of your own examples, possibly from sports, or maybe in a situation where you need to be very careful?
In the next section, you can read about a task (a type of computer game) that scientists use to study response inhibition. (You will even have a chance to try it!) In the section after the next, you can read about what these tasks have taught us about the brain. At the end, you can read a summary of why this research is so important.
Tasks
When scientists design tasks, they have to figure out how to make people’s brain do the same thing over and over again. This allows scientists to make better predictions about how the brain performs the task. The Stop Signal Task is used to study response inhibition. This task is simple … kind of. The task begins with the presentation of a target, for example, a picture or an arrow. You tell the participant in the experiment that when they see the target, they should push a button as fast as they can. This allows the scientists to determine how fast the person can react to the target. Pretty simple so far.
The catch is that, sometimes, a beep is played right after the target. The beep means STOP! So even if the participant has prepared to respond, they have to try to inhibit that response. This can be really tough! Playing the beep immediately after the target makes it easier to stop from pushing the button, but playing the beep later and later after the target makes it harder and harder to stop from pushing the button.
Want to give the Stop Signal Task a try? See how quickly you can respond to the target and how quickly you can stop!
Making the task very hard allows us to approximate how long it takes the participant to stop. Some people can stop really quickly, even if they are just about to push the button. Other people stop more slowly and need to hear the beep long before they start to push the button.
Using the Stop Signal Task, you can measure how quickly someone responds to the target when there is not a beep. You can also measure how quickly they stop. Typically, people can respond to a target in less than half a second, or under 500 ms, and people can stop themselves even faster, on the order of about a quarter of a second, or around 250 ms. (Remember, there are 1000 ms in a second.)
Response Inhibition and the Brain
Neuroscientists have studied response inhibition in lots of different ways. One way is to have people with damage (or lesions) to parts of the brain perform a task that uses response inhibition, like the Stop Signal Task. Then, you can compare their performance to people without any brain damage or who have damage in other parts of the brain. This technique tells you what parts of the brain are necessary for response inhibition. For example, damage to an area of the brain called the inferior frontal gyrus (IFG) (part of the frontal lobe, located underneath your temples) causes a person to be slower at inhibiting their responses (see Figure 1, adapted from Ref. [1]). In contrast, damage to other parts of the brain can result in more dramatic problems with stopping. For instance, damage to the subthalamic nucleus, deep in the center of the brain, can cause people to have uncontrollable movements all the time. This is called hemiballismus [http://www.youtube.com/watch?v=hqg2GTUq1k4]. Direct electrical stimulation of the subthalamic nucleus, which is a treatment for certain types of diseases, can also change a person’s ability to stop. This is done by implanting an electrode inside the brain.
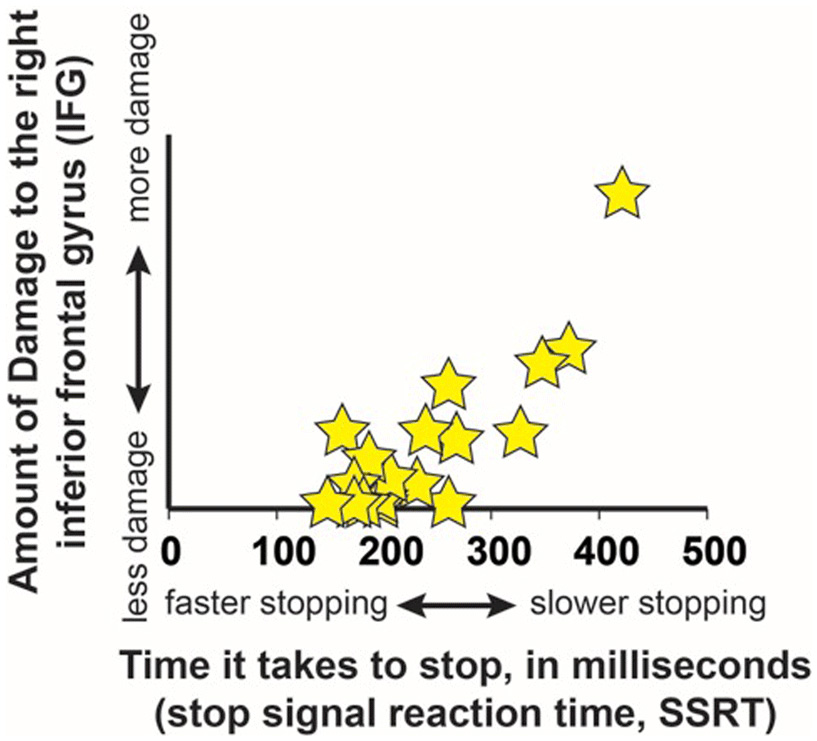
- Figure 1 - Damage to a part of the frontal lobe, the inferior frontal gyrus (IFG), on the right side, is associated with slower stopping.
- In this plot, each star represents data from one person. The horizontal axis shows the speed of stopping for each person, and the vertical axis shows how much damage they had to their right IFG. This plot shows that people with more damage to their right IFG have higher stop signal reaction times, or slower stopping. This data was published in Ref. [1].
Another way to determine if a brain area is necessary for a certain behavior is with a technique called transcranial magnetic stimulation (trans-CRAY-nee-ul mag-NET-tic stim-u-LAY-shun) or TMS. With this method, electricity is sent across the scalp and skull into the brain using a special paddle-shaped device that contains a coil of wire. This method allows scientists to temporarily disrupt activity in different parts on the surface of the brain (cortex). Scientists have used TMS to disrupt different parts of the brain while individuals performed the Stop Signal Task. They found that TMS of the IFG, on the right side of the head, impaired a person’s ability to stop quickly [2]. This agrees with the findings of the experiments done in people with lesions in the brain.
Functional Magnetic Resonance Imaging, or fMRI, is a different type of technique that takes pictures of changes in blood flow in your brain. These changes in blood flow are related to which brain areas are using up energy and tell us which areas of the brain may be involved in performing a task. Scientists have collected fMRI while people performed the Stop Signal Task to see which parts of the brain change activity during response inhibition. Studies using fMRI found activity changes in the IFG and around the subthalamic nucleus when people successfully stopped themselves. A third area called the pre-supplementary motor area, near the middle and top of the brain, also showed up in the fMRI. These findings mostly agree with the other studies. FMRI tells you a lot about which parts of the brain are active, but it does not tell you much about when activity changes occur.
Fortunately, other methods can provide faster measurements. Two of these techniques called electroencepholography (ee-LEK-tro-en-SEF-o-LOG-raf-ee), or EEG for short, and electrocorticography (ee-LEK-tro-COR-ti-COG-raf-ee), or ECoG, tell us information about when there are changes in electrical activity in the brain. These techniques are sensitive to tiny electrical signals generated by groups of brain cells (neurons). For EEG, changes in activity are detected using electrodes placed on the surface of your head, on top of the hair. For ECoG, the electrodes are placed by a surgeon right on the surface of the brain (cortex) after part of the skull has been removed. Using EEG and ECoG, scientists have detected changes in cortex activity that occur very soon after the stop signal is presented, between 100 and 200 ms. These studies also suggest that the brain cells signal at a particular rate or frequency to communicate throughout the brain during stopping.
One other method that has been useful for understanding which brain areas might communicate when inhibiting a response is called diffusion tensor imaging (di-FYOO-shun TEN-sore IM-a-jing), or DTI. Many brain cells have a special part called an axon that is a long narrow tube, almost like a tiny straw, and that is used to send signals between different brain areas (Figure 3). DTI can measure the direction that water flows inside the axons of brain cells. Most of your body is made up of water, including the brain, and some of this water is inside the axons. Scientists use this information to determine which brain areas are connected. This is important because different parts of the brain need to coordinate their activity to produce behavior. Scientists have used DTI to see if the IFG, pre-supplementary motor area, and subthalamic nucleus are directly connected to one another.
Scientists can consider the results from all of these methods to get a general idea about how the brain performs response inhibition during the Stop Signal Task. Each method adds a new piece to the puzzle: it is possible to learn which areas are necessary for stopping (with TMS or lesion studies), what parts of the brain are active during stopping (with fMRI and somewhat with ECoG and EEG), when the brain activity changes (with EEG or ECoG), and how different brain areas are connected (with DTI).
Scientists have conducted hundreds of experiments using these methods along with several other methods (that we do not have room to tell you about here). It ends up that response inhibition does not depend on one brain area, but instead depends on communication across a network of connected brain areas! Some of these areas are on the surface of the brain (the cortex), and include the IFG and pre-supplementary motor area, shown in Figure 2. There are also areas that are near the center of the brain. An example of one of these deep brain structures is the subthalamic nucleus, also shown in Figure 2. These areas communicate with each other using connections that have been identified using DTI. Scientists believe that this brain network is what allows you to stop yourself, like in the earlier example when you saw someone catch the line drive as you were about to sprint toward home plate. Scientists are still learning more about response inhibition all the time. In the future, we will probably find more brain areas that are important for response inhibition and more ways in which these brain areas communicate.
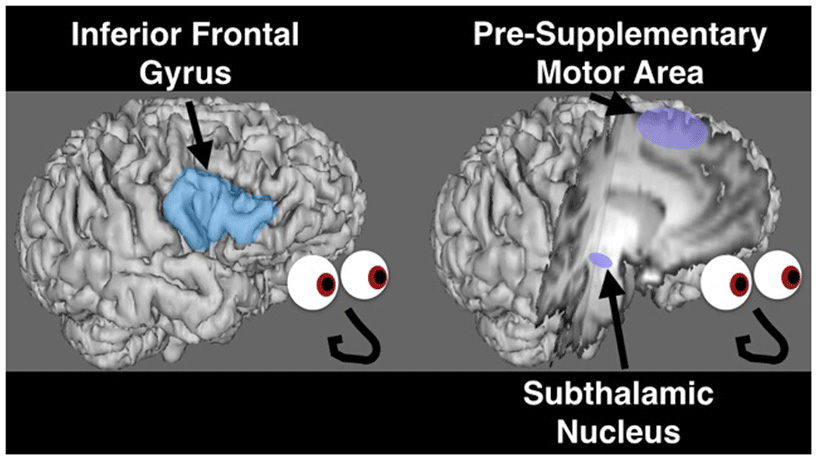
- Figure 2 - The network of brain areas believed to be involved in stopping includes the right inferior frontal gyrus, pre-supplementary motor area, and subthalamic nucleus.
- In these two images, these brain areas are highlighted on pictures of Dr. Greenhouse’s brain. The first image on the left shows the inferior frontal gyrus highlighted on the surface of the brain. The second image shows the regions of the pre-supplementary motor area and subthalamic nucleus. In the second image, the right front portion of the brain has been removed to show the inside of the brain. What a great looking brain, don’t you think?!
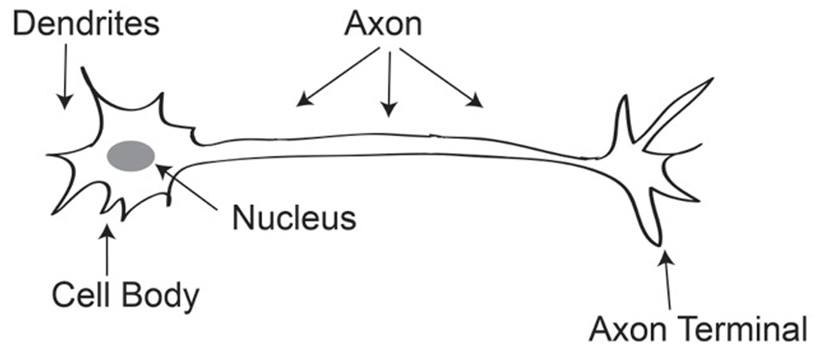
- Figure 3 - Brain cell (neuron).
- This diagram shows the key parts of a brain cell, or neuron. The axon, a straw-like portion of the cell, is labeled along with the other key parts: the nucleus, cell body, dendrites, and axon terminals.
Why is the Study of Response Inhibition Important?
Well, for one thing, stopping can save your life! Remember the example of running after a basketball that has rolled into the street and hearing the honk of an approaching car. Knowing what the horn means (STOP!), and being able to inhibit the tendency to run after the ball, saves you from a painful accident. Besides this, scientists are testing the idea that the same brain network used for inhibiting movements may be important for inhibiting unwanted thoughts and emotions as well. For example, you might rely on the same network of brain areas to stop your finger from pressing a button in the Stop Signal Task as you do to stop your attention from drifting to your favorite TV show playing in the next room when you need to stay focused on your homework. Not being able to control your attention in this way may underlie disorders such as attention deficit hyperactivity disorder (ADHD). In fact, scientists have shown that people with ADHD are impaired at stopping when they perform a Stop Signal Task [3]. The more we learn about how the brain performs response inhibition, the better we are able to understand what causes impairments, like in ADHD. This could someday lead to improved treatments or therapies to help people overcome impairments in the ability to control themselves. The study of response inhibition has also given neuroscientists a better understanding of how different parts of the brain work together to accomplish all the amazing things the brain can do!
Acknowledgements
We would like to thank Timothy Hoang for his help creating the video game and Robert for providing an excellent review of our article.
Keywords
Response Inhibition
Stop Signal Task
Lesion
Transcranial Magnetic Stimulation
Functional Magnetic Resonance Imaging
Electroencephalography
Electrocorticography
Diffusion Tensor Imaging
Inferior Frontal Gyrus
Pre-Supplementary Motor Area
Subthalamic Nucleus
Basal Ganglia
Brain Network
References
[1] ↑ Aron, A. R., Fletcher, P. C., Bullmore, T., Sahakian, B. J., and Robbins, T. W. 2003. Stop-signal inhibition disrupted by damage to right inferior frontal gyrus in humans. Nat. Neurosci. 6:115–116. doi: 10.1038/nn1003
[2] ↑ Chambers, C. D., Bellgrove, M. A., Stokes, M. G., Henderson, T. R., Garavan, H., Robertson, I. H., et al. 2006. Executive “brake failure” following deactivation of human frontal lobe. J. Cogn. Neurosci. 18:444–455. doi: 10.1162/089892906775990606
[3] ↑ Lijffijt, M., Kenemans, J. L., Verbaten, M. N., and van Engeland, H. 2005. A meta-analytic review of stopping performance in attention-deficit/hyperactivity disorder: deficient inhibitory motor control? J. Abnorm. Psychol. 114:216–222. doi: 10.1037/0021-843X.114.2.216